REVIEW article
Obesity: epidemiology, pathophysiology, and therapeutics.
- Department of Endocrinology, Sir Run Run Shaw Hospital, School of Medicine, Zhejiang University, Hangzhou, China
Obesity is a complex multifactorial disease that accumulated excess body fat leads to negative effects on health. Obesity continues to accelerate resulting in an unprecedented epidemic that shows no significant signs of slowing down any time soon. Raised body mass index (BMI) is a risk factor for noncommunicable diseases such as diabetes, cardiovascular diseases, and musculoskeletal disorders, resulting in dramatic decrease of life quality and expectancy. The main cause of obesity is long-term energy imbalance between consumed calories and expended calories. Here, we explore the biological mechanisms of obesity with the aim of providing actionable treatment strategies to achieve a healthy body weight from nature to nurture. This review summarizes the global trends in obesity with a special focus on the pathogenesis of obesity from genetic factors to epigenetic factors, from social environmental factors to microenvironment factors. Against this background, we discuss several possible intervention strategies to minimize BMI.
There has been a significant global increase in obesity rate during the last 50 years. Obesity is defined as when a person has a body mass index [BMI (kg/m 2 ), dividing a person’s weight by the square of their height] greater than or equal to 30, overweight is defined as a BMI of 25.0-29.9. Being overweight or obesity is linked with more deaths than being underweight and is a more common global occurrence than being underweight. This is a global phenomenon occurring in every region except parts of sub-Saharan Asia and Africa ( 1 ), and also countries with low obesity rates (i.e., Sri Lanka, Indonesia, Sudan, Singapore, Djibouti, etc.) ( 2 ).
Obesity increases the likelihood of various diseases and conditions which are linked to increased mortality. These include Type 2 diabetes mellitus (T2DM), cardiovascular diseases (CVD), metabolic syndrome (MetS), chronic kidney disease (CKD), hyperlipidemia, hypertension, nonalcoholic fatty liver disease (NAFLD), certain types of cancer, obstructive sleep apnea, osteoarthritis, and depression ( 3 ).Treating these conditions can place an additional load on healthcare systems: for example, it is estimated that obese have a 30% higher medical cost than those with a normal BMI ( 4 ). As related total health-care costs double every decade, treating the consequences of obesity poses an expensive challenge for patients ( 5 ).
There are several possible mechanisms leading to obesity. Actually, the traditional view is usually that the main cause is the significantly more excess energy stored than the energy the body used. The excess energy is stored in fat cells, thereby developing the characteristic obesity pathology. The pathologic enlargement of fat cells will alter the nutrient signals responsible for obesity ( 6 ).However, the latest research showed that the food sources and quality of nutrients matter more than their quantities in the diet for weight control, and also for disease prevention ( 7 ). More and more etiologies or defects that lead to obesity can be identified under the background of struggle between nurture and nature, genetic and epigenetic, environmental and microenvironment. We are increasingly understanding how food cravings are upregulated in obesity individuals’ brains, how gut hormones, adipose tissue, or gut microbiota regulate appetite and satiety in the hypothalamus, as well as the roles of gut dysbiosis played in obesity development and how dysfunction of glucose and lipids metabolism causes secondary health problems ( 8 ). In addition, genetic factors are known to play critical roles in determining an individual’s predisposition to weight gain ( 9 ). Recent epigenetic studies have provided very useful tools for understanding the worldwide increase in obesity ( 10 ). Studies have discussed the relationships between genetics, epigenetics, and environment in obesity and explored the roles of epigenetic factors in metabolism regulation and obesity risk as well as its complications ( 11 ).
The field of obesity is rapidly evolving as an abundance of new scientific data continue to emerge. Herein, we discuss the epidemiology of obesity, covering the pathophysiology, pathogenesis, genetics, epigenetics, and environmental (macro and micro) causes that result in obesity. We end by summarizing possible management and prevention strategies.

Epidemiology of Obesity
BMI is used to define and diagnose obesity according to World Health Organization (WHO) guidelines ( 4 ). In adults, WHO defines ‘overweight’ as a BMI of 25.0 to 29.9 and ‘obese’ as a BMI ≥ 30.0. Obesity is further classified into three severity levels: class I (BMI 30.0-34.9), class II (BMI 35.0-39.9) and class III (BMI ≥ 40.0) ( 12 ).However, large individual differences exist in the percent body fat for the given BMI value, which can be attributed to sex, ethnicity and age ( 13 ).Excess fat deposition in the abdominal region is termed ‘abdominal obesity’ and is associated with greater health risks ( 14 ).The definition and measurement guidelines of abdominal obesity differed from WHO, IDF (International Diabetes Federation) to AHA (American Heart Association) ( 15 ). However, there is no international standard suitable for all countries or regions.
The prevalence of excessive weight gain has doubled worldwide since 1980, and about a third of the global population has been determined to be obese or overweight ( 16 ). Obesity rate has dramatically enhanced in both male and female, and across all ages, with proportionally higher prevalence in older persons and women ( 4 ). While this trend is present globally, absolute prevalence rates vary across regions, countries, and ethnicities. The prevalence of obesity also varies with socioeconomic status, with slower rates of BMI increase in high-income and some middle-income countries. While obesity was once considered a problem of high-income countries, the incidence rates of obese or overweight children in high-income countries, including the United States, Sweden, Denmark, Norway, France, Australia and Japan, have decreased or plateaued since the early 2000s ( 17 ).
In low- and middle-income countries, rates of overweight and obesity are rising especially in urban areas. In China, one study based on 12,543 participants monitored over 22 years revealed that the prevalence of age-adjusted obesity rose from 2.15% to 13.99% in both sexes, going from 2.78 to 13.22% in female and from 1.46 to 14.99% in male, respectively ( 18 , 19 ). The overweight rate of African children under 5 years old has increased by 24% since 2000. As of 2019, almost half of the Asian children under 5 years old were obese or overweight ( 20 ). WHO datasets from sub-Sarahan Africa reveal that prevalence of overweight and obese in adults and stunting, underweight, and wasting in children are inversely associated ( 21 ).
Pathogenesis of Obesity
The pathogenesis of obesity involves regulation of calorie utilization, appetite, and physical activity, but have complex interactions with availability of health-care systems, the role of socio-economic status, and underlying hereditary and environmental factors.
Food Intake and Energy Balance
The essential causes of obesity remain somewhat controversial. Current health recommendations to manage obesity are based on the underlying physiological property that fat accumulation is driven by an energy imbalance between consumed and expended calories. The obesity epidemic has been fueled in large part by increased energy from greater availability of highly rewarding and energy-dense food. Diet and various social, economic, and environmental factors related to food supply have a significant effect on patient’s ability to achieve the balance ( 22 ). In a 13-year follow-up study on 3,000 young, those who consumed much more fast-food were found to weigh an average of ~6kg more and have larger waist circumferences than those with the lowest fast-food-intake. They were also found to have higher incidences of negative weight-related health issues, such as elevated triglycerides and twice the odds of developing MetS ( 23 ). These issues are compounded in certain individuals that possess a genetic susceptibility to fat accumulation, which may be caused by significant interactions between homeostatic circuits and brain reward. Accumulation of lipid metabolites, inflammatory signaling, or other hypothalamic neuron impairing mechanisms may also lead to obesity, which might explain the biological defense of elevated body fat mass ( 24 ).
Obesogenic marketing to promote beverages or foods that are high in sugar and fat negatively modulates human behavior. Such advertisements may increase preference for energy-dense foods and beverages ( 25 ). Analysis showed that African American programs had more food advertisements than other general market programs. More food advertisements were for meat, candy, soda, and fast food than for grains, pasta, cereals, vegetables, and fruits. Advertised products were designed to be cheap, have a long shelf-life, and taste ‘irresistible’. This applies particularly to high-fat, high-sugar junk foods that can stimulate the brain reward centers, the same part of the brain that’s stimulated by cocaine, heroin, and other addictive drugs, that is, these products are specifically engineered to be addictive ( 25 ). The brain reward offers a plausible mechanism to explain the elevated body fat mass, however, it seems that only certain individuals present this characteristic according to this theory.
For clinicians, a systematic evaluation of patient health factors affecting energy intake, metabolism, and expenditure is required for effective management of obesity. However, attempting to manage obesity through behavioral alterations aiming at addressing these three factors is more often than not unsuccessful. This suggests that our understanding of energy management and the interactions between intake, metabolism, and expenditure are not yet fully understood ( 26 ).
Family History and Lifestyle
Family history, lifestyle, and psychological factors all function in propensity for obesity. The likelihood of becoming obese can be affected by nature and nurture, enhanced by family genetics (propensity to accumulate fat) ( 27 ) or life style (poor dietary or exercise habits) ( 28 ). A child with one obese parent has a three-time risk to become obese as an adult, while when a child’s parents are both obese, this child has a 10-fold risk of future obesity. A cross-sectional observational study of 260 children (139 female, 121 male, aged 2.4 and 17.2 years) demonstrated that the family history of cardiometabolic diseases and obesity are critical risk factors for severity of obesity in childhood ( 29 ).
A prospective survey of 3148 school boys (aged six to ten years) in Ariana highlighted several child obesity risk factors, including parental obesity of parents, the snacks between meals especially after the dinner, lack of sleep (< 8 hours), and daily consumption of juice, sparkling drink, sweets, and sugary foods ( 30 ). Two studies of mother-child pairs in the United States found that the healthy lifestyle of mothers during the childhood and adolescence of their offspring was closely associated with a significantly reduced risk of obesity in their children ( 31 ). These results underscore the benefits of intervening at the family- or parental-level to reduce the risk of obesity in children ( 31 ).
However, parents are not the sole instigators of childhood obesity. For example, in the United States, physical education was used as a regular part of a public education curriculum ( 32 ). Starting 2011 physical education programs were curtailed such that 25 percent of students could achieve four out of five the national standards of at least 225 minutes weekly at the senior school levels and at least 150 minutes weekly at the primary level ( 33 ). Other factors that may have resulted in the decline of physical activity in children include increasing time spent on video game consoles and mobile devices at a reduction of time spent actively or outdoors. It is hard to argue against technological progress, but based on these studies, such innovations may be taking a toll on children’s health ( 34 ).
Microenvironment and Gut Microbiome
Our knowledge of the intestinal microbiome has grown substantially over recent years, as has our understanding of its intricate relationship to disease. For example, obesity is involved in an altered gut microenvironment that supports more diverse viral species than found in leaner hosts ( 35 ). This environment is more susceptible to the generation of pathogenic variants that can induce more serious disease ( 36 ). Increasing evidence shows that variations of gut microbiome cause alterations in host weight and metabolism. For example, compared with those with normal gut microbiota, germ-free male mice (without gut microflora) had 42% less total body fat, even while consuming 29% more food a day. However, after cecal microbe colonization, the total body fat of these mice increased 57%in, lean body mass decreased 7%, and daily food intake decreased 27% ( 35 ). A follow-up study suggested that these alterations resulted from decreased metabolic rates, with concomitant increased adipose tissue deposition, as capillary density in distal small intestinal villi increased 25% after microflora colonization. Similar results were also observed from female mice ( 37 ).
The human body contains around 3.8 × 10 13 microorganisms and the majority of them occupy the gastrointestinal tract. Over half of the microbial population are bacteria, followed by Archaea and Eukarya ( 38 ). The diversity of healthy gut microbiome allows for functional redundancy, in which multiple microbes can perform similar functions. Normally, gut microbiota have substantial beneficial roles in the host, including involvement in metabolism of carbohydrate and lipid, synthesis of vitamins and amino acids, epithelial cell proliferation, protection against pathogens, and hormone modulation. Gut bacteria can also break down indigestible molecules such as human milk oligosaccharides and plant polysaccharides ( 39 ). Imbalance of microbial populations (‘dysbiosis’) has been show to associate with a wide range of diseases including neurological disorders, inflammatory bowel disease, malnutrition, cancer, diabetes, and obesity ( 40 ). Recent research suggests that caloric restriction can beneficially reshape the gut microbiome and that antibiotic use can negatively harm gut microflora in ways that result in diabetes and obesity. Human studies support findings that microbiome alterations are associated with obesity; however, the exact mechanisms (i.e., ratios and amounts of microflora diversity) are still unknown ( 41 ).
Gut microbiota are central players in the host immune system. Disturbances in gut microflora can lead to inflammation of the intestinal lining ( 42 ). This response has been demonstrated to be mediated by TLRs (toll-like receptors), which identify and attack host microbes. For example, TLR4 recognizes the bacterial LPS (lipopolysaccharides) in the cell walls of Gram-negative bacteria while TLR5 recognizes bacterial flagellin. The body mass of TLR5-knockout mice increased 20% and their epididymal fat pad size increased 100% when compared to the wild-type controls ( 43 ). The dietary fiber and starch fermentation in lower gastrointestinal tract induced by microbiome can also produce SCFAs (Short-chain fatty acids), which can regulate production of gut hormone such as peptide YY (PYY) in the intestinal epithelium and GLP-1, GLP-2 (glucagon-like peptides), and the secretion of gastric inhibitory peptides by K cells ( 44 ). In obese patients, enzymes participated in or glucose signaling pathways are downregulated. It may be that alterations in specific microbial populations are more important than overall phylogenetic ratios, resulting in alterations in enzymes and SCFAs production, which further influence regulation of insulin and glucose, ultimately leading to development of obesity ( 41 ).
Genetic Factors and Causes
The studies from family and twin studies showed that around 40-70% of the obesity variation in human are resulted from genetic factors ( 45 ). While during the last 20 years, environmental alterations have increased obesity rates, the genetic factors play key roles in development of obesity ( 46 ). GWAS (Genome-wide association scans) approaches have identified over 400 genes associated with T2DM ( 47 , 48 ), however, these genes only predict 5% of obesity risk ( 49 ). The low predictive power may be due to the situation that gene-gene, gene-environment, and epigenetic interactions have not been thoroughly identified using the current methods based on population genetics ( 50 ). Many obesity -associated genes have been identified to be involved in energy homeostasis regulating pathways.
Genetic causes of obesity can be broadly classified as: 1) monogenic causes that result from a single gene mutation, primarily located in the leptin- melanocortin pathway. Many of the genes, such as AgRP (Agouti-related peptide), PYY (orexogenic), or MC4R (the melanocortin-4 receptor), were identified for monogenic obesity disrupt the regulatory system of appetite and weight, hormonal signals (ghrelin, leptin, insulin) are sensed by the receptors located in the arcuate nucleus of the hypothalamus ( 51 ). 2) Syndromic obesity were severe obesity results from neurodevelopmental abnormalities and other organ/system malformations. This may be caused by alterations in a single gene or a larger chromosomal region encompassing several genes ( 52 ). 3) Polygenic obesity is caused by cumulative contribution of many genes. Further, some people with obesity gain excess weight due to the multiple genes they have ( 53 ), and these genes make them to favor food and thereby have a higher caloric intake. The presence of these types of genes can cause increased caloric intake, increased hunger levels, reduced control overeating, reduced satiety, increased tendency to store body fat, and increased tendency to be sedentary ( 54 ).
Rare single-gene defects are associated with high level of hunger and can cause dramatic obesity in young children. Those individuals with severe obesity developed before two years old should consult obesity medicine specialists and consider to be involved in screening for MC4R Deficiency, leptin deficiency, and POMC deficiency ( 55 ). Leptin deficiency can cause diet-induced obesity and metabolic dysregulation. About 50% of female with polymorphism came up with binge eating ( 56 ). The MC4R polymorphism influences the release of ghrelin ( 57 ). The chromosome 2p22 (a region encompassing the POMC gene) has been identified as the site of gene(s) affecting obesity and obesity-related traits ( 58 ). These studies suggest that childhood obesity should be considered in the light of both environmental context and genetic heritage ( 59 ).
There are several genetic, neuroendocrine, and chromosomal precursors that can result in obesity. PWS (Prader-Willi Syndrome) is a neurodevelopmental disorder with hypothalamic dysfunction, due to the deficiency of imprinted genes ( 60 ). Endocrine disorders such as PCOS (Polycystic Ovary Syndrome) can also lead to increased body fat ( 61 ). Chromosomal defects can lead to obesity, including deletion of 16p11.2, 2q37 (brachydactyly mental retardation syndrome; BDMR), 1p36 (monosomy 1p36 syndrome), 9q34 (Kleefstra syndrome), 6q16 (PWS-like syndrome), 17p11.2 (Smith Magenis syndrome; SMS), and 11p13 (WAGR syndrome) ( 62 ). These conditions rely on the conventional current health recommendations that energy imbalance between calories consumed and expended is the key cause of obesity and present circumstances under which traditional weight management methods may not help.
Epigenetic Modification
We have been able to identify some of the genes that contribute to monogenic forms of obesity, but the human genome alterations on timescales that are too long for the genome to be a major player in the current obesity pandemic. Epigenetics, however, may offer a logical explanation for increasing obesity prevalence over the past few decades without necessitating a radical change in the genome ( 63 ). In multicellular organisms, the genetic code is homogenous throughout the body, but the expression of code can vary across cell types. Epigenetics studies showed that the heritable regulatory alterations in the genetic expression do not require alterations in the nucleotide sequence ( 64 ). Epigenetic modifications can be thought of as the differential packaging of the DNA that either allows or silences the expression of certain genes across tissues. Environmental and gut microbiota can influence the epigenetic programming of parental gametes, or programming in later stages of life ( 10 ).
The known epigenetic mechanisms include DNA methylation, histone modifications, and miRNA-mediated regulation. These can be passed from one generation to another meiotically or mitotically. There is evidence showing that the perinatal and embryo-fetal development period plays a critical role in human tissues and organs programming ( 65 ). DNA methylation appears to be the most important epigenetic mechanism for regulating gene expression. Alterations in DNA methylation can be a hallmark of many diseases such as cancers ( 66 ). LEP (Leptin) plays critical roles in adipose tissue regulation. The maternal metabolic status can affect DNA methylation of LEP profile at birth, affecting metabolic remodeling of obesity ( 67 ). The Adiponectin (ADIPOQ) epigenetic status also has relationship with obesity, and association has been reported between LDL-cholesterol levels and DNA methylation of both LEP and ADIPOQ ( 68 ). Paternal obesity has also been associated with inhibited methylation levels in IGF2 (insulin-like growth factor 2) regions, which promote the division and growth of various types of cells ( 69 ). Other genes investigated in the context of metabolism and obesity include: tumor necrosis factor (TNF), hypoxia-inducible factor 3a (HIF3A), neuropeptide Y (NPY), insulin receptor substrate 1 (IRS1), mitochondrial transcription factor A (TFAM), interleukin 6 (IL6), lymphocyte antigen 86 (LY86) and glucose transport 4 (GLUT4) ( 10 , 63 ).
Histones are proteins function in DNA packaging and modifications to histones are associated with epigenetic regulation of adipogenesis and obesity development ( 70 ). Five key regulatory genes in adipogenesis, CCAAT-enhancer-binding protein β (C/EBP β), pre-adipocyte factor-1 (Pref-1), adipocyte protein 2 (aP2), PPARγ, and C/EBPα, are modulated by histone modifications during adipocyte differentiation ( 71 ). The enzymes play roles in histone modification also function in obesity. They also regulate the expression of HDACs (histone deacetylases), which participated in the epigenetic control of gene expression involved in a large amount of environmental factors ( 72 ).
MicroRNAs (miRNAs) are 18 to 25 nucleotides long short noncoding RNA sequences that can regulate gene expression by gene silencing and post-transcriptional alterations. MicroRNAs function in a variety of biological processes, including adipocyte differentiation and proliferation, and are associated with low-grade inflammation and insulin resistance displayed in obese individuals ( 73 ). Increased levels of miRNAs including miR-486-3p, miR-142-3p, miR-486-5p, miR-423-5p and miR-130b were seen in children with high BMI values, among which 10 miRNAs exhibited significant alterations with increasing body weight ( 74 ). Zhao et al. identified miRNA as a signature for weight gain and showed that the individuals with a high-risk score for 8 of these miRNAs had over 3-fold higher odds of weight gain ( 75 ). Alterations in adipocyte-derived exosomal miRNAs is also seen following weight loss and decrease in insulin resistance after gastric bypass ( 76 ). miRNAs have been shown to play a key role in obesity and that the associated metabolic alterations can serve as biomarkers, or potentially therapeutic targets for intervention. Consideration of genetic and epigenetic causes of obesity provide valuable tools for the clinical treatment of obesity.
Therapeutics of Obesity
Lifestyle modifications.
Given the lack of specific pharmacological interventions, ‘lifestyle modification’ remains the cornerstone of obesity management ( 4 ). Individuals with obesity are suggested to lose at least 10% body weight via combination of diet, physical activity, and behavior therapy (or lifestyle modification) ( 77 ). Significant short-term weight loss can be achieved by consumption of portion-controlled diets ( 78 ). Long-term weight control can be achieved via high levels of physical activity and continued patient–practitioner contact. In many cases, lifestyle modification results in dramatic loss of body weight, leading to significant reduction of cardiovascular risk ( 79 ).
Since food choices are mainly determined by peoples’ surroundings, it is imperative that governments improve policies and environment to reduce the availability of unhealthful foods and make healthy foods more accessible. Policies should be changed to increase development of foods with reduced sugar, fat, and salt and decrease availability of obesogenic foods aimed at children ( 80 ). Policy makers and Practitioners must be made aware of the potential impact of food advertisements on human health and behavior and should encourage food manufacturers to create and promote weight-friendly foods. Nutrition educators should help teach how to evaluate food advertisements ( 81 ). Interventions aimed at motivating behavioral alterations (e.g., health promotion, nutrition education, incentives for healthy living, sugar-sweetened beverage tax, and social marketing) and enforcing actions that reduce causes of obesity (e.g., policy changes, regulations and laws) are likely to have strong impacts on reducing the obesity crisis ( 82 ).
Anti-Obesity Medications
Pharmacotherapy is recommended for those whose BMI ≥30 (or a BMI ≥27 with comorbid conditions) and are unable to lose weight using lifestyle modification alone ( 83 ). The U.S. FDA (Food and Drug Administration) approved some new pharmacotherapy drugs for short-term obesity treatment ( Table 1 ) and since Lorcaserin was withdrawn, there are only four [Naltrexone-Bupropion (Contrave), Orlistat (Xenical, Alli), Liraglutide (Saxenda) and Phentermine-Topiramate (Qsymia)] approved in addition to Gelesis which is now the fifth, have been approved for long-term use ( 84 , 86 , 87 ). The FDA also approved the MC4R agonist-Setmelanotide for use in individuals with severe obesity due to either POMC, PCSK1 (proprotein convertase subtilisin/kexin type 1), or LEPR (leptin receptor) deficiency at the end of 2020 ( 85 ).
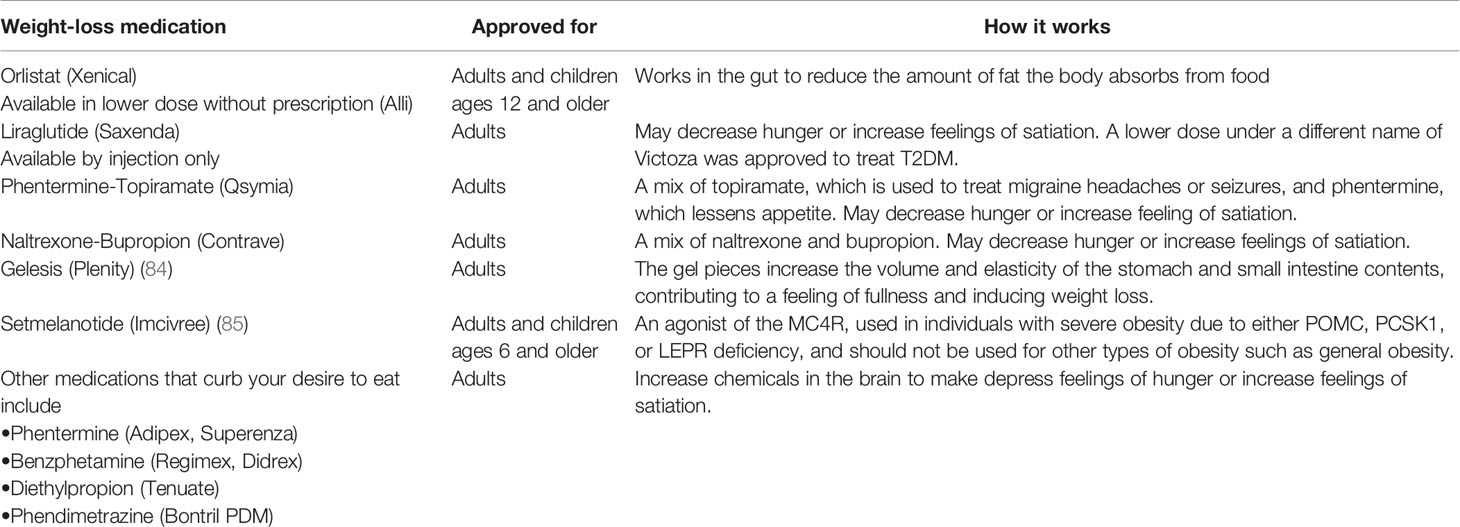
Table 1 Prescription medications approved for obesity treatment.
In addition, 11 different components have been identified from 54 families of the plants to have anti-obesity potential. These families include Celastraceae, Zingiberaceae, Theaceae, Magnoliaceae, and Solanaceae ( 88 ). Traditional Chinese medicine delivers unique solutions to treat obesity, such as regulating fat metabolism, enhancing hormone level, regulating intestinal microflora, among other pathways ( 89 ). These findings are helpful for selection of herbal medicine or traditional Chinese medicine for further research.
Bariatric Surgery
For individuals with a BMI > 40 or BMI > 35 with comorbidities who are unable to lose weight by lifestyle modifications or pharmacotherapy bariatric surgery or weight loss surgery is another option ( 83 ). Standard bariatric operations, including BPD (Bilio-pancreatic diversion), SG (sleeve gastrectomy), RYGB (Roux-en-Y gastric bypass), and AGB (adjustable gastric banding), benefits individuals׳ metabolic profiles to varying degrees ( 90 ). Studies reported that the benefits of bariatric surgery go beyond just losing weight. Bariatric surgery reduces chronic inflammation involved in obesity and alters biomarkers, the gut microbiota, and long-term remission for T2DM ( 91 – 93 ). Take RYGB for example, in human subjects, overall gut microbial richness increased after RYGB surgery ( 94 ). Further analysis revealed RYGB contributed to increase of expression of some specific white adipose tissue genes, upregulation of genes central to the transforming growth factor-β signaling pathway, and remarkable downregulation of genes involved in metabolic pathways and inflammatory responses ( 95 ). Decrease of serum leptin levels, which are associated with leaned BMI, typically results from bariatric surgery. Interestingly, those women who had a higher presurgical baseline leptin level were easier to remain the post-procedure weight loss, while those with a lower presurgical baseline level were easier to regain the weight. There is a correlation between the baseline leptin level and alterations in body mass, BMI, as well as total weight loss although the success degree of surgery cannot be predicted by a patient’s serum leptin level ( 96 ).
Fecal Microbiota Transplantation
FMT has attracted considerable research interest recently in the treatment of obesity ( 97 ). There are promising indications that FMT of microbes from healthy individuals into patients with obesity may be affected in weight loss and maintenance. In a groundbreaking key study, Ridaura et al. transplanted fecal slurries from human twins discordant for obesity into germ-free mice ( 98 ). The mice with obese individuals’ microbiota successfully developed obesity, while those with healthy individuals’ microbiota remained lean. The sequencing results of mice post-procedure stool samples showed that the human microbiomes were successfully infused, indicative of the transfer of functions related to the obese or lean microbial communities, respectively ( 98 ). Promising studies in humans are also being attempted: Vrieze et al. were able to improve microbial diversity and insulin sensitivity in obese, diabetic adult males after the transplantation with the taxa from lean donors ( 99 ). An increase was observed in butyrate-producing bacteria and Bacteroidetes, indicative of a shift toward a leaner phenotype related microbial community. While in early stages, FMT may be an option for replacing obesogenic microbial communities ( 100 ).
Summary and Conclusions
The global prevalence of obesity has nearly tripled since 1975 and continues to grow at an exponential rate. Obesity has become the number one lifestyle-related risk factor for premature death. As such, public health policies focused on reducing and treating obesity must be developed ( 17 ). The WHO “Global Action Plan for the Prevention and Control of Noncommunicable Diseases 2013-2020” defines strategies to prevent further increase in obesity prevalence, but progress so far has been slow ( 101 ). However, with the identification of the main obesity causes the modulating factors, the challenge remains is to translate them into effective actions.
Epigenetic modifications and interactions between our genes and environment have strong influences on human health and disease. Increasing evidence is revealing the involvement of epigenetics in obesity prevalence ( 9 ). Propensity for obesity can result from the effects of environmental factors, such as nutrition and lifestyle to the epigenetic remodeling of the early postnatal development, and parental gametes. Epigenetic marks could also significantly affect the obesity risk of the child and thus be transmitted trans-generationally ( 11 ). This epigenetic ‘memory’ may help explain our lack of evidence for genetic heritability in obesity and other diseases. A foundational knowledge of the mechanisms of epigenetic inheritance is of great importance for treating and preventing obesity. Exploration of epigenetic changes is a key for predicting disease trajectories and choosing effective treatment. The reversible characteristic of these modifications makes them ideal targets for epigenetic treatment, and promising “epigenetic drugs” for therapies of obesity are already in the marketplace or in various stages of development ( 102 ). These types of therapies include DNA methyltransferase inhibitors (DNMTis), protein arginine methyltransferase inhibitors (PRMTis), histone acetyltransferase inhibitors (HATi), histone deacetylase inhibitors (HDACi), sirtuin-activating compounds (STACs) and histone demethylating inhibitors (HDMis) ( 103 ).
Microbiome research holds much promise for treating pandemics such as obesity and diabetes. On-going developments in technology and bioinformatics of microbiology are increasingly allowing for the development of a microbiome-manipulating capsule to favor a healthy, lean, and insulin-sensitive profile, but this is still an area of active research ( 8 , 104 ). More targeted therapies will also become possible as we increase our understanding of microbial metabolites, allowing for clinal treatment of inflammation, weight gain and insulin resistance, and ultimately preventing the progression to obesity.
In conclusion, improved understanding of the various dimension of obesity, including propensity to regain lost weight, interindividual differences in pathogenesis, and response to therapy, is needed for developing effective as well as cost-effective interventions. The insights will in turn benefit the related health complications such as incidence of diabetes. More research is required to identify behavioral modification that are effective and available to people from diverse backgrounds. More studies were performed to develop more effective and safer medications to help obese people lose body weight and maintain a healthy weight for long term. Moreover, we must devote greater efforts and resources to the prevention of obesity in children as well as adults. Prevention is a key as treatment alone is not very effective and cannot well reverse the epidemic of obesity for long term.
Author Contributions
HL and XL conceived and wrote the manuscript. All authors contributed to the article and approved the submitted version.
This project was funded by grants from the Zhejiang Provincial Medical Science and Technology Program (2020KY166).
Conflict of Interest
The authors declare that the research was conducted in the absence of any commercial or financial relationships that could be construed as a potential conflict of interest.
Publisher’s Note
All claims expressed in this article are solely those of the authors and do not necessarily represent those of their affiliated organizations, or those of the publisher, the editors and the reviewers. Any product that may be evaluated in this article, or claim that may be made by its manufacturer, is not guaranteed or endorsed by the publisher.
1. Al Kibria GM. Prevalence and Factors Affecting Underweight, Overweight and Obesity Using Asian and World Health Organization Cutoffs Among Adults in Nepal: Analysis of the Demographic and Health Survey 2016. Obes Res Clin Pract (2019) 13(2):129–36. doi: 10.1016/j.orcp.2019.01.006
PubMed Abstract | CrossRef Full Text | Google Scholar
2. Mbogori T, Kimmel K, Zhang M, Kandiah J, Wang Y. Nutrition Transition and Double Burden of Malnutrition in Africa: A Case Study of Four Selected Countries With Different Social Economic Development. AIMS Public Health (2020) 7:425–39. doi: 10.3934/publichealth.2020035
3. Swinburn BA, Sacks G, Hall KD, McPherson K, Finegood DT, Moodie ML, et al. The Global Obesity Pandemic: Shaped by Global Drivers and Local Environments. Lancet (2011) 378(9793):804–14. doi: 10.1016/S0140-6736(11)60813-1
4. WHO Consultation on Obesity (1999: Geneva, Switzerland) & World Health Organization. Obesity: Preventing and Managing the Global Epidemic. Report of a WHO Consultation. World Health Organ Tech Rep Ser (2000) 894:i–xii, 1-253.
PubMed Abstract | Google Scholar
5. Bray GA, Kim KK, Wilding J, World Obesity Federation. Obesity: A Chronic Relapsing Progressive Disease Process. A Position Statement of the World Obesity Federation. Obes Rev (2017) 18(7):715–23.doi: 10.1111/obr.12551
6. Lee SJ, Shin SW. Mechanisms, Pathophysiology, and Management of Obesity. N Engl J Med (2017) 376(15):1491–2. doi: 10.1056/NEJMc1701944
7. Sacks FM, Bray GA, Carey VJ, Smith SR, Ryan DH, Anton SD, et al. Comparison of Weight-Loss Diets With Different Compositions of Fat, Protein, and Carbohydrates. N Engl J Med (2009) 360(9):859–73. doi: 10.1056/NEJMoa0804748
8. Singer-Englar T, Barlow G, Mathur R. Obesity, Diabetes, and the Gut Microbiome: An Updated Review. Expert Rev Gastroenterol Hepatol (2019) 13(1):3–15. doi: 10.1080/17474124.2019.1543023
9. Singh RK, Kumar P, Mahalingam K. Molecular Genetics of Human Obesity: A Comprehensive Review. C R Biol (2017) 340(2):87–108. doi: 10.1016/j.crvi.2016.11.007
10. Lopomo A, Burgio E, Migliore L. Epigenetics of Obesity. Prog Mol Biol Transl Sci (2016) 140:151–84. doi: 10.1016/bs.pmbts.2016.02.002
11. Dubern B. Genetics and Epigenetics of Obesity: Keys to Understand. Rev Prat (2019) 69(9):1016–9.
12. Poirier P, Giles TD, Bray GA, Hong Y, Stern JS, Pi-Sunyer FX, et al. Obesity and Cardiovascular Disease: Pathophysiology, Evaluation, and Effect of Weight Loss. Arterioscler Thromb Vasc Biol (2006) 26(5):968–76. doi: 10.1161/01.ATV.0000216787.85457.f3
13. Kok P, Seidell JC, Meinders AE. [The Value and Limitations of the Body Mass Index (BMI) in the Assessment of the Health Risks of Overweight and Obesity]. Ned Tijdschr Geneeskd (2004) 148(48):2379–82.
14. Løvsletten O, Jacobsen BK, Grimsgaard S, Njølstad I, Wilsgaard T, Løchen ML, et al. Prevalence of General and Abdominal Obesity in 2015-2016 and 8-Year Longitudinal Weight and Waist Circumference Changes in Adults and Elderly: The Tromsø Study. BMJ Open (2020) 10(11):e038465. doi: 10.1136/bmjopen-2020-038465
15. Paley CA, Johnson MI. Abdominal Obesity and Metabolic Syndrome: Exercise as Medicine. BMC Sports Sci Med Rehabil (2018) 10:7. doi: 10.1186/s13102-018-0097-1
16. Ataey A, Jafarvand E, Adham D, Moradi-Asl E. The Relationship Between Obesity, Overweight, and the Human Development Index in World Health Organization Eastern Mediterranean Region Countries. J Prev Med Public Health (2020) 53(2):98–105. doi: 10.3961/jpmph.19.100
17. NCD Risk Factor Collaboration (NCD-RisC). Worldwide Trends in Body-Mass Index, Underweight, Overweight, and Obesity From 1975 to 2016: A Pooled Analysis of 2416 Population-Based Measurement Studies in 128·9 Million Children, Adolescents, and Adults. Lancet (2017) 390(10113):2627–42. doi: 10.1016/S0140-6736(17)32129-3
18. Chen Y, Peng Q, Yang Y, Zheng S, Wang Y, Lu W. The Prevalence and Increasing Trends of Overweight, General Obesity, and Abdominal Obesity Among Chinese Adults: A Repeated Cross-Sectional Study. BMC Public Health (2019) 19(1):1293. doi: 10.1186/s12889-019-7633-0
19. Jia W. Obesity in China: Its Characteristics, Diagnostic Criteria, and Implications. Front Med (2015) 9(2):129–33. doi: 10.1007/s11684-015-0387-x
20. Wariri O, Alhassan J, Mark G, Adesiyan O, Hanson L. Trends in Obesity by Socioeconomic Status Among non-Pregnant Women Aged 15-49 Y: A Cross-Sectional, Multi-Dimensional Equity Analysis of Demographic and Health Surveys in 11 Sub-Saharan Africa Countries, 1994-2015. Int Health (2020) 0:1–10. doi: 10.1093/inthealth/ihaa093
CrossRef Full Text | Google Scholar
21. Tydeman-Edwards R, Van Rooyen FC, Walsh CM. Obesity, Undernutrition and the Double Burden of Malnutrition in the Urban and Rural Southern Free State, South Africa. Heliyon (2018) 4(12):e00983. doi: 10.1016/j.heliyon.2018.e00983
22. Yoo S. Dynamic Energy Balance and Obesity Prevention. J Obes Metab Syndr (2018) 27(4):203–12. doi: 10.7570/jomes.2018.27.4.203
23. Duffey KJ, Gordon-Larsen P, Jacobs DR Jr, Williams OD, Popkin BM. Differential Associations of Fast Food and Restaurant Food Consumption With 3-Y Change in Body Mass Index: The Coronary Artery Risk Development in Young Adults Study. Am J Clin Nutr (2007) 85(1):201–8. doi: 10.1093/ajcn/85.1.201
24. Obri A, Claret M. The Role of Epigenetics in Hypothalamic Energy Balance Control: Implications for Obesity. Cell Stress (2019) 3(7):208–20. doi: 10.15698/cst2019.07.191
25. Sadeghirad B, Duhaney T, Motaghipisheh S, Campbell NR, Johnston BC. Influence of Unhealthy Food and Beverage Marketing on Children's Dietary Intake and Preference: A Systematic Review and Meta-Analysis of Randomized Trials. Obes Rev (2016) 17(10):945–59. doi: 10.1111/obr.12445
26. Willett WC, Leibel RL. Dietary Fat Is Not a Major Determinant of Body Fat. Am J Med (2002) 113(Suppl 9B):47S–59S. doi: 10.1016/S0002-9343(01)00992-5
27. Fitzgerald MP, Hennigan K, O'Gorman CS, McCarron L. Obesity, Diet and Lifestyle in 9-Year-Old Children With Parentally Reported Chronic Diseases: Findings From the Growing Up in Ireland Longitudinal Child Cohort Study. Ir J Med Sci (2019) 188(1):29–34. doi: 10.1007/s11845-018-1814-1
28. Romero-Ibarguengoitia ME, Vadillo-Ortega F, Caballero AE, Ibarra-González I, Herrera-Rosas A, Serratos-Canales MF, et al. Family History and Obesity in Youth, Their Effect on Acylcarnitine/Aminoacids Metabolomics and Non-Alcoholic Fatty Liver Disease (NAFLD). Structural Equation Modeling Approach. PloS One (2018) 13(2):e0193138. doi: 10.1371/journal.pone.0193138
29. Corica D, Aversa T, Valenzise M, Messina MF, Alibrandi A, De Luca F, et al. Does Family History of Obesity, Cardiovascular, and Metabolic Diseases Influence Onset and Severity of Childhood Obesity. Front Endocrinol (Lausanne) (2018) 9:187. doi: 10.3389/fendo.2018.00187
30. Ben Slama F, Achour A, Belhadj O, Hsairi M, Oueslati M, Achour N. [Obesity and Life Style in a Population of Male School Children Aged 6 to 10 Years in Ariana (Tunisia)]. Tunis Med (2002) 80(9):542–7.
31. Dhana K, Haines J, Liu G, Zhang C, Wang X, Field AE, et al. Association Between Maternal Adherence to Healthy Lifestyle Practices and Risk of Obesity in Offspring: Results From Two Prospective Cohort Studies of Mother-Child Pairs in the United States. BMJ (2018) 362:k2486. doi: 10.1136/bmj.k2486
32. Mulligan EP, Rauh MJ, Heiderscheit B, Jenkins WL. Sports Physical Therapy Education in the United States: Where Do We Go From Here? A Survey of American Academy of Sports Physical Therapy Members. J Allied Health (2020) 49:e79–79e87.
33. Beets MW, Wallner M, Beighle A. Defining Standards and Policies for Promoting Physical Activity in Afterschool Programs. J Sch Health (2010) 80:411–7. doi: 10.1111/j.1746-1561.2010.00521.x
34. Salam RA, Padhani ZA, Das JK, Shaikh AY, Hoodbhoy Z, Jeelani S, et al. Effects of Lifestyle Modification Interventions to Prevent and Manage Child and Adolescent Obesity: A Systematic Review and Meta-Analysis. Nutrients (2020) 12(8):2208. doi: 10.3390/nu12082208
35. Bäckhed F, Ding H, Wang T, Hooper LV, Koh GY, Nagy A, et al. The Gut Microbiota as an Environmental Factor That Regulates Fat Storage. Proc Natl Acad Sci USA (2004) 101(44):15718–23. doi: 10.1073/pnas.0407076101
36. Honce R, Karlsson EA, Wohlgemuth N, Estrada LD, Meliopoulos VA, Yao J, et al. Obesity-Related Microenvironment Promotes Emergence of Virulent Influenza Virus Strains. mBio (2020) 11(2):e03341–19. doi: 10.1128/mBio.03341-19
37. Stappenbeck TS, Hooper LV, Gordon JI. Developmental Regulation of Intestinal Angiogenesis by Indigenous Microbes via Paneth Cells. Proc Natl Acad Sci USA (2002) 99(24):15451–5. doi: 10.1073/pnas.202604299
38. Sender R, Fuchs S, Milo R. Revised Estimates for the Number of Human and Bacteria Cells in the Body. PloS Biol (2016) 14(8):e1002533. doi: 10.1371/journal.pbio.1002533
39. Jandhyala SM, Talukdar R, Subramanyam C, Vuyyuru H, Sasikala M, Nageshwar Reddy D. Role of the Normal Gut Microbiota. World J Gastroenterol (2015) 21(29):8787–803. doi: 10.3748/wjg.v21.i29.8787
40. DeGruttola AK, Low D, Mizoguchi A, Mizoguchi E. Current Understanding of Dysbiosis in Disease in Human and Animal Models. Inflammation Bowel Dis (2016) 22(5):1137–50. doi: 10.1097/MIB.0000000000000750
41. Gao R, Zhu C, Li H, Yin M, Pan C, Huang L, et al. Dysbiosis Signatures of Gut Microbiota Along the Sequence From Healthy, Young Patients to Those With Overweight and Obesity. Obes (Silver Spring) (2018) 26(2):351–61. doi: 10.1002/oby.22088
42. Chassaing B, Raja SM, Lewis JD, Srinivasan S, Gewirtz AT. Colonic Microbiota Encroachment Correlates With Dysglycemia in Humans. Cell Mol Gastroenterol Hepatol (2017) 4(2):205–21. doi: 10.1016/j.jcmgh.2017.04.001
43. Vijay-Kumar M, Aitken JD, Carvalho FA, Cullender TC, Mwangi S, Srinivasan S, et al. Metabolic Syndrome and Altered Gut Microbiota in Mice Lacking Toll-Like Receptor 5. Science (2010) 328(5975):228–31. doi: 10.1126/science.1179721
44. Gill PA, van Zelm MC, Muir JG, Gibson PR. Review Article: Short Chain Fatty Acids as Potential Therapeutic Agents in Human Gastrointestinal and Inflammatory Disorders. Aliment Pharmacol Ther (2018) 48(1):15–34. doi: 10.1111/apt.14689
45. Wu Y, Duan H, Tian X, Xu C, Wang W, Jiang W, et al. Genetics of Obesity Traits: A Bivariate Genome-Wide Association Analysis. Front Genet (2018) 9:179. doi: 10.3389/fgene.2018.00179
46. Kasuga M. [Genetic Factor for Diabetes and Obesity]. Nihon Rinsho (2010) 68(Suppl 8):359–63.
47. Srinivasan S, Chen L, Todd J, Divers J, Gidding S, Chernausek S, et al. The First Genome-Wide Association Study for Type 2 Diabetes in Youth: The Progress in Diabetes Genetics in Youth (ProDiGY) Consortium. Diabetes (2021) 70:996–1005. doi: 10.2337/db20-0443
48. Chen J, Sun M, Adeyemo A, Pirie F, Carstensen T, Pomilla C, et al. Genome-Wide Association Study of Type 2 Diabetes in Africa. Diabetologia (2019) 62:1204–11. doi: 10.1007/s00125-019-4880-7
49. Bogardus C. Missing Heritability and GWAS Utility. Obes (Silver Spring) (2009) 17(2):209–10. doi: 10.1038/oby.2008.613
50. Wang T, Xu M, Bi Y, Ning G. Interplay Between Diet and Genetic Susceptibility in Obesity and Related Traits. Front Med (2018) 12(6):601–7. doi: 10.1007/s11684-018-0648-6
51. Thaker VV. Genetic And Epigenetic Causes Of Obesity. Adolesc Med State Art Rev (2017) 28(2):379–405.
52. Huvenne H, Dubern B, Clément K, Poitou C. Rare Genetic Forms of Obesity: Clinical Approach and Current Treatments in 2016. Obes Facts (2016) 9(3):158–73. doi: 10.1159/000445061
53. Czajkowski P, Adamska-Patruno E, Bauer W, Fiedorczuk J, Krasowska U, Moroz M, et al. The Impact of FTO Genetic Variants on Obesity and Its Metabolic Consequences Is Dependent on Daily Macronutrient Intake. Nutrients (2020) 12(11):3255. doi: 10.3390/nu12113255
54. Koochakpour G, Esfandiar Z, Hosseini-Esfahani F, Mirmiran P, Daneshpour MS, Sedaghati-Khayat B, et al. Evaluating the Interaction of Common FTO Genetic Variants, Added Sugar, and Trans-Fatty Acid Intakes in Altering Obesity Phenotypes. Nutr Metab Cardiovasc Dis (2019) 29(5):474–80. doi: 10.1016/j.numecd.2019.01.005
55. Martins MC, Trujillo J, Freitas-Vilela AA, Farias DR, Rosado EL, Struchiner CJ, et al. Associations Between Obesity Candidate Gene Polymorphisms (Fat Mass and Obesity-Associated (FTO), Melanocortin-4 Receptor (MC4R), Leptin (LEP) and Leptin Receptor (LEPR)) and Dietary Intake in Pregnant Women. Br J Nutr (2018) 120(4):454–63. doi: 10.1017/S0007114518001423
56. Yupanqui-Lozno H, Bastarrachea RA, Yupanqui-Velazco ME, Alvarez-Jaramillo M, Medina-Méndez E, Giraldo-Peña AP, et al. Congenital Leptin Deficiency and Leptin Gene Missense Mutation Found in Two Colombian Sisters With Severe Obesity. Genes (Basel) (2019) 10(5):342. doi: 10.3390/genes10050342
57. Magno F, Guaraná HC, da Fonseca A, Pedrosa AP, Zembrzuski VM, Cabello PH, et al. Association of the MC4R Rs17782313 Polymorphism With Plasma Ghrelin, Leptin, IL6 and Tnfα Concentrations, Food Intake and Eating Behaviors in Morbidly Obese Women. Eat Weight Disord (2020) 26(4):1079–87. doi: 10.1007/s40519-020-01003-5
58. Yu H, Chhabra KH, Thompson Z, Jones GL, Kiran S, Shangguan G, et al. Hypothalamic POMC Deficiency Increases Circulating Adiponectin Despite Obesity. Mol Metab (2020) 35:100957. doi: 10.1016/j.molmet.2020.01.021
59. Cunha M, Aparício G, Duarte J, Pereira A, Albuquerque C, Oliveira A. Genetic Heritage as a Risk Factor Enabling Chilhood Obesity. Aten Primaria (2013) 45(Suppl 2):201–7. doi: 10.1016/S0212-6567(13)70023-4
60. Gupta N, Jain V. Prader Willi Syndrome - A Common Epigenetic Cause of Syndromic Obesity. Indian J Pediatr (2017) 84:809–10. doi: 10.1007/s12098-017-2512-0
61. Cena H, Chiovato L, Nappi RE. Obesity, Polycystic Ovary Syndrome, and Infertility: A New Avenue for GLP-1 Receptor Agonists. J Clin Endocrinol Metab (2020) 105(8):e2695–709. doi: 10.1210/clinem/dgaa285
62. D'Angelo CS, Koiffmann CP. Copy Number Variants in Obesity-Related Syndromes: Review and Perspectives on Novel Molecular Approaches. J Obes (2012) 2012:845480. doi: 10.1155/2012/845480
63. Rosen ED, Kaestner KH, Natarajan R, Patti ME, Sallari R, Sander M, et al. Epigenetics and Epigenomics: Implications for Diabetes and Obesity. Diabetes (2018) 67(10):1923–31. doi: 10.2337/db18-0537
64. Jaenisch R, Bird A. Epigenetic Regulation of Gene Expression: How the Genome Integrates Intrinsic and Environmental Signals. Nat Genet (2003) 33(Suppl):245–54. doi: 10.1038/ng1089
65. Holmes D. Epigenetics: On-Off Switch for Obesity. Nat Rev Endocrinol (2016) 12(3):125. doi: 10.1038/nrendo.2016.18
66. Burgio E, Lopomo A, Migliore L. Obesity and Diabetes: From Genetics to Epigenetics. Mol Biol Rep (2015) 42(4):799–818. doi: 10.1007/s11033-014-3751-z
67. Sherwood WB, Bion V, Lockett GA, Ziyab AH, Soto-Ramírez N, Mukherjee N, et al. Duration of Breastfeeding Is Associated With Leptin (LEP) DNA Methylation Profiles and BMI in 10-Year-Old Children. Clin Epigenet (2019) 11(1):128. doi: 10.1186/s13148-019-0727-9
68. Houde AA, Légaré C, Biron S, Lescelleur O, Biertho L, Marceau S, et al. Leptin and Adiponectin DNA Methylation Levels in Adipose Tissues and Blood Cells are Associated With BMI, Waist Girth and LDL-Cholesterol Levels in Severely Obese Men and Women. BMC Med Genet (2015) 16:29. doi: 10.1186/s12881-015-0174-1
69. Soubry A, Schildkraut JM, Murtha A, Wang F, Huang Z, Bernal A, et al. Paternal Obesity is Associated With IGF2 Hypomethylation in Newborns: Results From a Newborn Epigenetics Study (NEST) Cohort. BMC Med (2013) 11:29. doi: 10.1186/1741-7015-11-29
70. Bannister AJ, Kouzarides T. Regulation of Chromatin by Histone Modifications. Cell Res (2011) 21(3):381–95. doi: 10.1038/cr.2011.22
71. Zhang Q, Ramlee MK, Brunmeir R, Villanueva CJ, Halperin D, Xu F. Dynamic and Distinct Histone Modifications Modulate the Expression of Key Adipogenesis Regulatory Genes. Cell Cycle (2012) 11(23):4310–22. doi: 10.4161/cc.22224
72. Funato H, Oda S, Yokofujita J, Igarashi H, Kuroda M. Fasting and High-Fat Diet Alter Histone Deacetylase Expression in the Medial Hypothalamus. PloS One (2011) 6(4):e18950. doi: 10.1371/journal.pone.0018950
73. Pasquinelli AE. MicroRNAs and Their Targets: Recognition, Regulation and an Emerging Reciprocal Relationship. Nat Rev Genet (2012) 13(4):271–82. doi: 10.1038/nrg3162
74. Cruz K, de Oliveira A, Morais J, Severo JS, Marreiro D. Role of microRNAs on Adipogenesis, Chronic Low-Grade Inflammation, and Insulin Resistance in Obesity. Nutrition (2017) 35:28–35. doi: 10.1016/j.nut.2016.10.003
75. Zhao H, Shen J, Daniel-MacDougall C, Wu X, Chow WH. Plasma MicroRNA Signature Predicting Weight Gain Among Mexican-American Women. Obes (Silver Spring) (2017) 25(5):958–64. doi: 10.1002/oby.21824
76. Hubal MJ, Nadler EP, Ferrante SC, Barberio MD, Suh JH, Wang J, et al. Circulating Adipocyte-Derived Exosomal MicroRNAs Associated With Decreased Insulin Resistance After Gastric Bypass. Obes (Silver Spring) (2017) 25(1):102–10. doi: 10.1002/oby.21709
77. Guidelines (2013) for Managing Overweight and Obesity in Adults. Preface to the Expert Panel Report (Comprehensive Version Which Includes Systematic Evidence Review, Evidence Statements, and Recommendations). Obes (Silver Spring) (2014) 22 Suppl 2:S40. doi: 10.1002/oby.20822
78. Lee EY, Yoon KH. Epidemic Obesity in Children and Adolescents: Risk Factors and Prevention. Front Med (2018) 12(6):658–66. doi: 10.1007/s11684-018-0640-1
79. Nguyen B, Clements J. Obesity Management Among Patients With Type 2 Diabetes and Prediabetes: A Focus on Lifestyle Modifications and Evidence of Antiobesity Medications. Expert Rev Endocrinol Metab (2017) 12(5):303–13. doi: 10.1080/17446651.2017.1367285
80. Mozaffarian D, Hao T, Rimm EB, Willett WC, Hu FB. Changes in Diet and Lifestyle and Long-Term Weight Gain in Women and Men. N Engl J Med (2011) 364(25):2392–404. doi: 10.1056/NEJMoa1014296
81. Struben J, Chan D, Dubé L. Policy Insights From the Nutritional Food Market Transformation Model: The Case of Obesity Prevention. Ann N Y Acad Sci (2014) 1331:57–75. doi: 10.1111/nyas.12381
82. Lal A, Mantilla-Herrera AM, Veerman L, Backholer K, Sacks G, Moodie M, et al. Correction: Modelled Health Benefits of a Sugar-Sweetened Beverage Tax Across Different Socioeconomic Groups in Australia: A Cost-Effectiveness and Equity Analysis. PloS Med (2020) 17(7):e1003310. doi: 10.1371/journal.pmed.1003310
83. Telles S, Gangadhar BN, Chandwani KD. Lifestyle Modification in the Prevention and Management of Obesity. J Obes (2016) 2016:5818601. doi: 10.1155/2016/5818601
84. Greenway FL, Aronne LJ, Raben A, Astrup A, Apovian CM, Hill JO, et al. A Randomized, Double-Blind, Placebo-Controlled Study of Gelesis100: A Novel Nonsystemic Oral Hydrogel for Weight Loss. Obes (Silver Spring) (2019) 27:205–16. doi: 10.1002/oby.22347
85. Yeo G, Chao D, Siegert AM, Koerperich ZM, Ericson MD, Simonds SE, et al. The Melanocortin Pathway and Energy Homeostasis: From Discovery to Obesity Therapy. Mol Metab (2021) 48:101206. doi: 10.1016/j.molmet.2021.101206
86. Gomez G, Stanford FC. US Health Policy and Prescription Drug Coverage of FDA-Approved Medications for the Treatment of Obesity. Int J Obes (Lond) (2018) 42(3):495–500. doi: 10.1038/ijo.2017.287
87. Rebello CJ, Greenway FL. Obesity Medications in Development. Expert Opin Investig Drugs (2020) 29:63–71. doi: 10.1080/13543784.2020.1705277
88. Karri S, Sharma S, Hatware K, Patil K. Natural Anti-Obesity Agents and Their Therapeutic Role in Management of Obesity: A Future Trend Perspective. BioMed Pharmacother (2019) 110:224–38. doi: 10.1016/j.biopha.2018.11.076
89. Gong S, Ye T, Wang M, Wang M, Li Y, Ma L, et al. Traditional Chinese Medicine Formula Kang Shuai Lao Pian Improves Obesity, Gut Dysbiosis, and Fecal Metabolic Disorders in High-Fat Diet-Fed Mice. Front Pharmacol (2020) 11:297. doi: 10.3389/fphar.2020.00297
90. Aminian A, Zelisko A, Kirwan JP, Brethauer SA, Schauer PR. Exploring the Impact of Bariatric Surgery on High Density Lipoprotein. Surg Obes Relat Dis (2015) 11:238–47. doi: 10.1016/j.soard.2014.07.017
91. Osto M, Abegg K, Bueter M, le Roux CW, Cani PD, Lutz TA. Roux-En-Y Gastric Bypass Surgery in Rats Alters Gut Microbiota Profile Along the Intestine. Physiol Behav (2013) 119:92–6. doi: 10.1016/j.physbeh.2013.06.008
92. Al-Rubaye H, McGlone ER, Farzaneh B, Mustafa L, Johnson M, Kayal A, et al. Roux-En-Y Gastric Bypass or Sleeve Gastrectomy for Obstructive Sleep Apnea: A Systematic Review and Meta-Analysis. Laparoscopic Endoscopic Robotic Surg (2019) 2(3):53–8. doi: 10.1016/j.lers.2019.05.002
93. Kops NL, Vivan MA, Fülber ER, Fleuri M, Fagundes J, Friedman R. Preoperative Binge Eating and Weight Loss After Bariatric Surgery: A Systematic Review and Meta-Analysis. Obes Surg (2020) 31(3):1239–48. doi: 10.1007/s11695-020-05124-9
94. Kong LC, Tap J, Aron-Wisnewsky J, Pelloux V, Basdevant A, Bouillot JL, et al. Gut Microbiota After Gastric Bypass in Human Obesity: Increased Richness and Associations of Bacterial Genera With Adipose Tissue Genes. Am J Clin Nutr (2013) 98(1):16–24. doi: 10.3945/ajcn.113.058743
95. Zhang H, DiBaise JK, Zuccolo A, Kudrna D, Braidotti M, Yu Y, et al. Human Gut Microbiota in Obesity and After Gastric Bypass. Proc Natl Acad Sci USA (2009) 106(7):2365–70. doi: 10.1073/pnas.0812600106
96. van Leiden HA, Dekker JM, Moll AC, Nijpels G, Heine RJ, Bouter LM, et al. Blood Pressure, Lipids, and Obesity are Associated With Retinopathy: The Hoorn Study. Diabetes Care (2002) 25(8):1320–5. doi: 10.2337/diacare.25.8.1320
97. Yu EW, Gao L, Stastka P, Cheney MC, Mahabamunuge J, Torres Soto M, et al. Fecal Microbiota Transplantation for the Improvement of Metabolism in Obesity: The FMT-TRIM Double-Blind Placebo-Controlled Pilot Trial. PloS Med (2020) 17(3):e1003051. doi: 10.1371/journal.pmed.1003051
98. Ridaura VK, Faith JJ, Rey FE, Cheng J, Duncan AE, Kau AL, et al. Gut Microbiota From Twins Discordant for Obesity Modulate Metabolism in Mice. Science (2013) 341(6150):1241214. doi: 10.1126/science.1241214
99. Vrieze A, Van Nood E, Holleman F, Salojärvi J, Kootte RS, Bartelsman JF, et al. Transfer of Intestinal Microbiota From Lean Donors Increases Insulin Sensitivity in Individuals With Metabolic Syndrome. Gastroenterology (2012) 143(4):913–6.e7. doi: 10.1053/j.gastro.2012.06.031
100. Zhang Z, Mocanu V, Cai C, Dang J, Slater L, Deehan EC, et al. Impact of Fecal Microbiota Transplantation on Obesity and Metabolic Syndrome-A Systematic Review. Nutrients (2019) 11(10):2291. doi: 10.3390/nu11102291
101. Phan HD, Nguyen T, Bui PL, Pham TT, Doan TV, Nguyen, et al. Overweight and Obesity Among Vietnamese School-Aged Children: National Prevalence Estimates Based on the World Health Organization and International Obesity Task Force Definition. PloS One (2020) 15(10):e0240459. doi: 10.1371/journal.pone.0240459
102. Epigenetic Drugs. Nat Biotechnol (2020) 38(5):558. doi: 10.1038/s41587-020-0523-2
103. Arguelles AO, Meruvu S, Bowman JD, Choudhury M. Are Epigenetic Drugs for Diabetes and Obesity at Our Door Step. Drug Discov Today (2016) 21(3):499–509. doi: 10.1016/j.drudis.2015.12.001
104. Ley RE, Turnbaugh PJ, Klein S, Gordon JI. Microbial Ecology: Human Gut Microbes Associated With Obesity. Nature (2006) 444(7122):1022–3. doi: 10.1038/4441022a
Keywords: obesity, epidemiology, pathophysiology, genetics, epigenetics, microenvironment
Citation: Lin X and Li H (2021) Obesity: Epidemiology, Pathophysiology, and Therapeutics. Front. Endocrinol. 12:706978. doi: 10.3389/fendo.2021.706978
Received: 08 May 2021; Accepted: 10 August 2021; Published: 06 September 2021.
Reviewed by:
Copyright © 2021 Lin and Li. This is an open-access article distributed under the terms of the Creative Commons Attribution License (CC BY) . The use, distribution or reproduction in other forums is permitted, provided the original author(s) and the copyright owner(s) are credited and that the original publication in this journal is cited, in accordance with accepted academic practice. No use, distribution or reproduction is permitted which does not comply with these terms.
*Correspondence: Hong Li, [email protected]
Disclaimer: All claims expressed in this article are solely those of the authors and do not necessarily represent those of their affiliated organizations, or those of the publisher, the editors and the reviewers. Any product that may be evaluated in this article or claim that may be made by its manufacturer is not guaranteed or endorsed by the publisher.
Loading metrics
Open Access
Perspective
The Perspective section provides experts with a forum to comment on topical or controversial issues of broad interest.
See all article types »
Obesity research: Moving from bench to bedside to population
* E-mail: [email protected]
Affiliation Diabetes Research Program, Department of Medicine, New York University Grossman School of Medicine, New York, New York, United States of America

- Ann Marie Schmidt
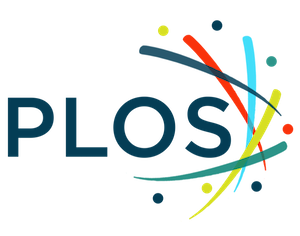
Published: December 4, 2023
- https://doi.org/10.1371/journal.pbio.3002448
- Reader Comments
Globally, obesity is on the rise. Research over the past 20 years has highlighted the far-reaching multisystem complications of obesity, but a better understanding of its complex pathogenesis is needed to identify safe and lasting solutions.
Citation: Schmidt AM (2023) Obesity research: Moving from bench to bedside to population. PLoS Biol 21(12): e3002448. https://doi.org/10.1371/journal.pbio.3002448
Copyright: © 2023 Ann Marie Schmidt. This is an open access article distributed under the terms of the Creative Commons Attribution License , which permits unrestricted use, distribution, and reproduction in any medium, provided the original author and source are credited.
Funding: AMS received funding from U.S. Public Health Service (grants 2P01HL131481 and P01HL146367). The funders had no role in study design, data collection and analysis, decision to publish, or preparation of the manuscript.
Competing interests: The author has declared that no competing interests exist.
Abbreviations: EDC, endocrine disruptor chemical; GIP, gastric inhibitory polypeptide; GLP1, glucagon-like peptide 1; HFCS, high-fructose corn syrup
This article is part of the PLOS Biology 20th anniversary collection.
Obesity is a multifaceted disorder, affecting individuals across their life span, with increased prevalence in persons from underrepresented groups. The complexity of obesity is underscored by the multiple hypotheses proposed to pinpoint its seminal mechanisms, such as the “energy balance” hypothesis and the “carbohydrate–insulin” model. It is generally accepted that host (including genetic factors)–environment interactions have critical roles in this disease. The recently framed “fructose survival hypothesis” proposes that high-fructose corn syrup (HFCS), through reduction in the cellular content of ATP, stimulates glycolysis and reduces mitochondrial oxidative phosphorylation, processes that stimulate hunger, foraging, weight gain, and fat accumulation [ 1 ]. The marked upswing in the use of HFCS in beverages and foods, beginning in the 1980s, has coincided with the rising prevalence of obesity.
The past few decades of scientific progress have dramatically transformed our understanding of pathogenic mechanisms of obesity ( Fig 1 ). Fundamental roles for inflammation were unveiled by the discovery that tumor necrosis factor-α contributed to insulin resistance and the risk for type 2 diabetes in obesity [ 2 ]. Recent work has ascribed contributory roles for multiple immune cell types, such as monocytes/macrophages, neutrophils, T cells, B cells, dendritic cells, and mast cells, in disturbances in glucose and insulin homeostasis in obesity. In the central nervous system, microglia and their interactions with hypothalamic neurons affect food intake, energy expenditure, and insulin sensitivity. In addition to cell-specific contributions of central and peripheral immune cells in obesity, roles for interorgan communication have been described. Extracellular vesicles emitted from immune cells and from adipocytes, as examples, are potent transmitters of obesogenic species that transfer diverse cargo, including microRNAs, proteins, metabolites, lipids, and organelles (such as mitochondria) to distant organs, affecting functions such as insulin sensitivity and, strikingly, cognition, through connections to the brain [ 3 ].
- PPT PowerPoint slide
- PNG larger image
- TIFF original image
Basic, clinical/translational, and epidemiological research has made great strides in the past few decades in uncovering novel components of cell-intrinsic, intercellular, and interorgan communications that contribute to the pathogenesis of obesity. Both endogenous and exogenous (environmental) stressors contribute to the myriad of metabolic perturbations that impact energy intake and expenditure; mediate innate disturbances in the multiple cell types affected in obesity in metabolic organelles and organs, including in immune cells; and impair beneficial interkingdom interactions of the mammalian host with the gut microbiome. The past few decades have also witnessed remarkable efforts to successfully treat obesity, such as the use of the incretin agonists and bariatric surgery. Yet, these and other strategies may be accompanied by resistance to weight loss, weight regain, adverse effects of interventions, and the challenges of lifelong implementation. Hence, through leveraging novel discoveries from the bench to the bedside to the population, additional strategies to prevent obesity and weight regain post-weight loss, such as the use of “wearables,” with potential for implementation of immediate and personalized behavior modifications, may hold great promise as complementary strategies to prevent and identify lasting treatments for obesity. Figure created with BioRender.
https://doi.org/10.1371/journal.pbio.3002448.g001
Beyond intercellular communication mediated by extracellular vesicles, the discovery of interactions between the host and the gut microbiome has suggested important roles for this interkingdom axis in obesity. Although disturbances in commensal gut microbiota species and their causal links to obesity are still debated, transplantation studies have demonstrated relationships between Firmicutes/Bacteroidetes ratios and obesity [ 4 ]. Evidence supports the concept that modulation of gut microbiota phyla modulates fundamental activities, such as thermogenesis and bile acid and lipid metabolism. Furthermore, compelling discoveries during the past few decades have illustrated specific mechanisms within adipocytes that exert profound effects on organismal homeostasis, such as adipose creatine metabolism, transforming growth factor/SMAD signaling, fibrosis [ 5 ], hypoxia and angiogenesis, mitochondrial dysfunction, cellular senescence, impairments in autophagy, and modulation of the circadian rhythm. Collectively, these recent discoveries set the stage for the identification of potential new therapeutic approaches in obesity.
Although the above discoveries focus largely on perturbations in energy metabolism (energy intake and expenditure) as drivers of obesity, a recently published study suggests that revisiting the timeline of obesogenic forces in 20th and 21st century society may be required. The authors tracked 320,962 Danish schoolchildren (born during 1930 to 1976) and 205,153 Danish male military conscripts (born during 1939 to 1959). Although the overall trend of the percentiles of the distributions of body mass index were linear across the years of birth, with percentiles below the 75th being nearly stable, those above the 75th percentile demonstrated a steadily steeper rise the more extreme the percentile; this was noted in the schoolchildren and the military conscripts [ 6 ]. The authors concluded that the emergence of the obesity epidemic might have preceded the appearance of the factors typically ascribed to mediating the obesogenic transformation of society by several decades. What are these underlying factors and their yet-to-be-discovered mechanisms?
First, in terms of endogenous factors relevant to individuals, stressors such as insufficient sleep and psychosocial stress may impact substrate metabolism, circulating appetite hormones, hunger, satiety, and weight gain [ 7 ]. Reduced access to healthy foods rich in vegetables and fruits but easy access to ultraprocessed ingredients in “food deserts” and “food swamps” caused excessive caloric intake and weight gain in clinical studies [ 8 ]. Second, exogenous environmental stresses have been associated with obesity. For example, air pollution has been directly linked to adipose tissue dysfunction [ 9 ], and ubiquitous endocrine disruptor chemicals (EDCs) such as bisphenols and phthalates (found in many items of daily life including plastics, food, clothing, cosmetics, and paper) are linked to metabolic dysfunction and the development of obesity [ 10 ]. Hence, factors specific to individuals and their environment may exacerbate their predisposition to obesity.
In addition to the effects of exposure to endogenous and exogenous stressors on the risk of obesity, transgenerational (passed through generations without direct exposure of stimulant) and intergenerational (direct exposure across generations) transmission of these stressors has also been demonstrated. A leading proposed mechanism is through epigenetic modulation of the genome, which then predisposes affected offspring to exacerbated responses to obesogenic conditions such as diet. A recent study suggested that transmission of disease risk might be mediated through transfer of maternal oocyte-derived dysfunctional mitochondria from mothers with obesity [ 11 ]. Additional mechanisms imparting obesogenic “memory” may be evoked through “trained immunity.”
Strikingly, the work of the past few decades has resulted in profound triumphs in the treatment of obesity. Multiple approved glucagon-like peptide 1 (GLP1) and gastric inhibitory polypeptide (GIP) agonists [ 12 ] (alone or in combinations) induce highly significant weight loss in persons with obesity [ 13 ]. However, adverse effects of these agents, such as pancreatitis and biliary disorders, have been reported [ 14 ]. Therefore, the long-term safety and tolerability of these drugs is yet to be determined. In addition to pharmacological agents, bariatric surgery has led to significant weight loss as well. However, efforts to induce weight loss through reduction in caloric intake and increased physical activity, pharmacological approaches, and bariatric surgery may not mediate long-term cures in obesity on account of resistance to weight loss, weight regain, adverse effects of interventions, and the challenges of lifelong implementation of these measures.
Where might efforts in combating obesity lie in the next decades? At the level of basic and translational science, the heterogeneity of metabolic organs could be uncovered through state-of-the-art spatial “omics” and single-cell RNA sequencing approaches. For example, analogous to the deepening understanding of the great diversity in immune cell subsets in homeostasis and disease, adipocyte heterogeneity has also been suggested, which may reflect nuances in pathogenesis and treatment approaches. Further, approaches to bolster brown fat and thermogenesis may offer promise to combat evolutionary forces to hoard and store fat. A better understanding of which interorgan communications may drive obesity will require intensive profiling of extracellular vesicles shed from multiple metabolic organs to identify their cargo and, critically, their destinations. In the three-dimensional space, the generation of organs-on-a-chip may facilitate the discovery of intermetabolic organ communications and their perturbations in the pathogenesis of obesity and the screening of new therapies.
Looking to prevention, recent epidemiological studies suggest that efforts to tackle obesity require intervention at multiple levels. The institution of public health policies to reduce air pollution and the vast employment of EDCs in common household products could impact the obesity epidemic. Where possible, the availability of fresh, healthy foods in lieu of highly processed foods may be of benefit. At the individual level, focused attention on day-to-day behaviors may yield long-term benefit in stemming the tide of obesity. “Wearable” devices that continuously monitor the quantity, timing, and patterns of food intake, physical activity, sleep duration and quality, and glycemic variability might stimulate on-the-spot and personalized behavior modulation to contribute to the prevention of obesity or of maintenance of the weight-reduced state.
Given the involvement of experts with wide-ranging expertise in the science of obesity, from basic science, through clinical/translational research to epidemiology and public health, it is reasonable to anticipate that the work of the next 2 decades will integrate burgeoning multidisciplinary discoveries to drive improved efforts to treat and prevent obesity.
Acknowledgments
The author is grateful to Ms. Latoya Woods of the Diabetes Research Program for assistance with the preparation of the manuscript and to Ms. Kristen Dancel-Manning for preparation of the Figure accompanying the manuscript.
- View Article
- PubMed/NCBI
- Google Scholar
Thank you for visiting nature.com. You are using a browser version with limited support for CSS. To obtain the best experience, we recommend you use a more up to date browser (or turn off compatibility mode in Internet Explorer). In the meantime, to ensure continued support, we are displaying the site without styles and JavaScript.
- View all journals
- Explore content
- About the journal
- Publish with us
- Sign up for alerts
- Review Article
- Published: 23 September 2021
The genetics of obesity: from discovery to biology
- Ruth J. F. Loos ORCID: orcid.org/0000-0002-8532-5087 1 , 2 , 3 , 4 &
- Giles S. H. Yeo ORCID: orcid.org/0000-0001-8823-3615 5
Nature Reviews Genetics volume 23 , pages 120–133 ( 2022 ) Cite this article
155k Accesses
448 Citations
481 Altmetric
Metrics details
- Disease genetics
- Endocrine system and metabolic diseases
- Genetic association study
- Genetic variation
The prevalence of obesity has tripled over the past four decades, imposing an enormous burden on people’s health. Polygenic (or common) obesity and rare, severe, early-onset monogenic obesity are often polarized as distinct diseases. However, gene discovery studies for both forms of obesity show that they have shared genetic and biological underpinnings, pointing to a key role for the brain in the control of body weight. Genome-wide association studies (GWAS) with increasing sample sizes and advances in sequencing technology are the main drivers behind a recent flurry of new discoveries. However, it is the post-GWAS, cross-disciplinary collaborations, which combine new omics technologies and analytical approaches, that have started to facilitate translation of genetic loci into meaningful biology and new avenues for treatment.
Similar content being viewed by others
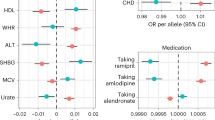
A phenome-wide comparative analysis of genetic discordance between obesity and type 2 diabetes
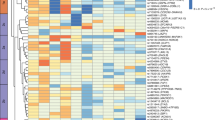
Genome-wide discovery of genetic loci that uncouple excess adiposity from its comorbidities
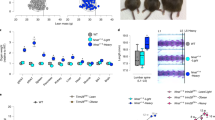
Independent phenotypic plasticity axes define distinct obesity sub-types
Introduction.
Obesity is associated with premature mortality and is a serious public health threat that accounts for a large proportion of the worldwide non-communicable disease burden, including type 2 diabetes, cardiovascular disease, hypertension and certain cancers 1 , 2 . Mechanical issues resulting from substantially increased weight, such as osteoarthritis and sleep apnoea, also affect people’s quality of life 3 . The impact of obesity on communicable disease, in particular viral infection 4 , has recently been highlighted by the discovery that individuals with obesity are at increased risk of hospitalization and severe illness from COVID-19 (refs 5 , 6 , 7 ).
On the basis of the latest data from the NCD Risk Factor Collaboration, in 2016 almost 2 billion adults (39% of the world’s adult population) were estimated to be overweight (defined by a body mass index (BMI) of ≥25 kg m − 2 ), 671 million (12% of the world’s adult population) of whom had obesity (BMI ≥30 kg m − 2 ) — a tripling in the prevalence of obesity since 1975 (ref. 8 ) (Fig. 1 ). Although the rate of increase in obesity seems to be declining in most high-income countries, it continues to rise in many low-income and middle-income countries and prevalence remains high globally 8 . If current trends continue, it is expected that 1 billion adults (nearly 20% of the world population) will have obesity by 2025. Particularly alarming is the global rise in obesity among children and adolescents; more than 7% had obesity in 2016 compared with less than 1% in 1975 (ref. 8 ).
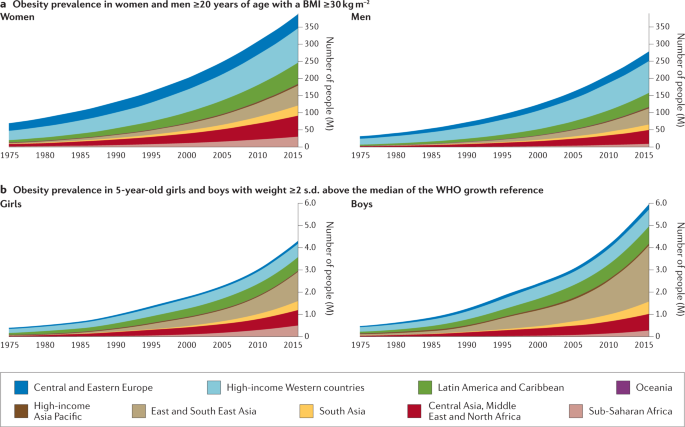
The prevalence of obesity has risen steadily over the past four decades in children, adolescents (not shown) and adults worldwide. a | Prevalence of obesity (body mass index (BMI) ≥30 kg m −2 ) in women and men ≥20 years of age, from 1975 to 2016. b | Prevalence of obesity (weight ≥2 s.d. above the median of the WHO growth reference) in 5-year-old girls and boys from 1975 to 2016. Geographical regions are represented by different colours. Graphs are reproduced from the NCD Risk Factor Collaboration (NCD RisC) website and are generated from data published in ref. 8 .
Although changes in the environment have undoubtedly driven the rapid increase in prevalence, obesity results from an interaction between environmental and innate biological factors. Crucially, there is a strong genetic component underlying the large interindividual variation in body weight that determines people’s response to this ‘obesogenic’ environment . Twin, family and adoption studies have estimated the heritability of obesity to be between 40% and 70% 9 , 10 . As a consequence, genetic approaches can be leveraged to characterize the underlying physiological and molecular mechanisms that control body weight.
Classically, we have considered obesity in two broad categories (Fig. 2 ): so-called monogenic obesity , which is inherited in a Mendelian pattern, is typically rare, early-onset and severe and involves either small or large chromosomal deletions or single-gene defects; and polygenic obesity (also known as common obesity), which is the result of hundreds of polymorphisms that each have a small effect. Polygenic obesity follows a pattern of heritability that is similar to other complex traits and diseases. Although often considered to be two distinct forms, gene discovery studies of monogenic and polygenic obesity have converged on what seems to be broadly similar underlying biology. Specifically, the central nervous system (CNS) and neuronal pathways that control the hedonic aspects of food intake have emerged as the major drivers of body weight for both monogenic and polygenic obesity. Furthermore, early evidence shows that the expression of mutations causing monogenic obesity may — at least in part — be influenced by the individual’s polygenic susceptibility to obesity 11 .
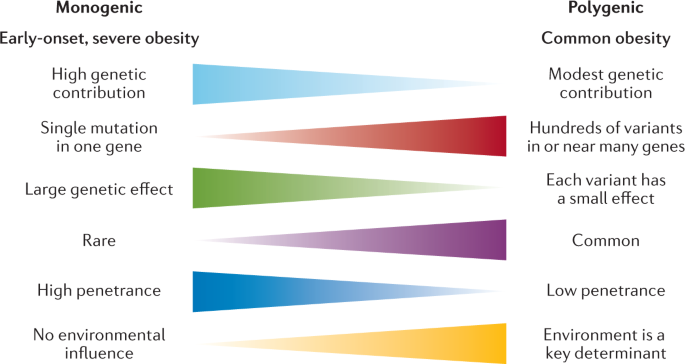
Key features of monogenic and polygenic forms of obesity .
In this Review, we summarize more than 20 years of genetic studies that have characterized the molecules and mechanisms that control body weight, specifically focusing on overall obesity and adiposity, rather than fat distribution or central adiposity. Although most of the current insights into the underlying biology have been derived from monogenic forms of obesity, recent years have witnessed several successful variant-to-function translations for polygenic forms of obesity. We also explore how the ubiquity of whole-exome sequencing (WES) and genome sequencing has begun to blur the line that used to demarcate the monogenic causes of obesity from common polygenic obesity. Syndromic forms of obesity, such as Bardet–Biedl, Prader–Willi, among many others 12 , are not reviewed here. Although obesity is often a dominant feature of these syndromes, the underlying genetic defects are often chromosomal abnormalities and typically encompass multiple genes, making it difficult to decipher the precise mechanisms directly related to body-weight regulation. Finally, as we enter the post-genomic era, we consider the prospects of genotype-informed treatments and the possibility of leveraging genetics to predict and hence prevent obesity.
Gene discovery approaches
The approaches used to identify genes linked to obesity depend on the form of obesity and genotyping technology available at the time. Early gene discovery studies for monogenic forms of obesity had a case-focused design: patients with severe obesity, together with their affected and unaffected family members, were examined for potential gene-disrupting causal mutations via Sanger sequencing. By contrast, genetic variation associated with common forms of obesity have been identified in large-scale population studies, either using a case–control design or continuous traits such as BMI. Gene discovery for both forms of obesity was initially hypothesis driven; that is, restricted to a set of candidate genes that evidence suggests have a role in body-weight regulation. Over the past two decades, however, advances in high-throughput genome-wide genotyping and sequencing technologies, combined with a detailed knowledge of the human genetic architecture, have enabled the interrogation of genetic variants across the whole genome for their role in body-weight regulation using a hypothesis-generating approach.
Gene discovery for monogenic obesity
Many of the candidate genes and pathways linked to body-weight regulation were initially identified in mice, such as the obese ( ob ) 13 and diabetes ( db ) 14 mouse lines, in which severe hyperphagia and obesity spontaneously emerged. Using reverse genetics , the ob gene was shown to encode leptin, a hormone produced from fat, and it was demonstrated that leptin deficiency resulting from a mutation in the ob gene caused the severe obesity seen in the ob/ob mouse 15 (Fig. 3 ). Shortly after the cloning of ob , the db gene was cloned and identified as encoding the leptin receptor (LEPR) 16 . Reverse genetics was also used to reveal that the complex obesity phenotype of Agouti ‘lethal yellow’ mice is caused by a rearrangement in the promoter sequence of the agouti gene that results in ectopic and constitutive expression of the agouti peptide 17 , 18 , which antagonizes the melanocortin 1 and 4 receptors (MC1R and MC4R) 19 , 20 . This finding linked the melanocortin pathway to body-weight regulation, thereby unveiling a whole raft of new candidate genes for obesity.
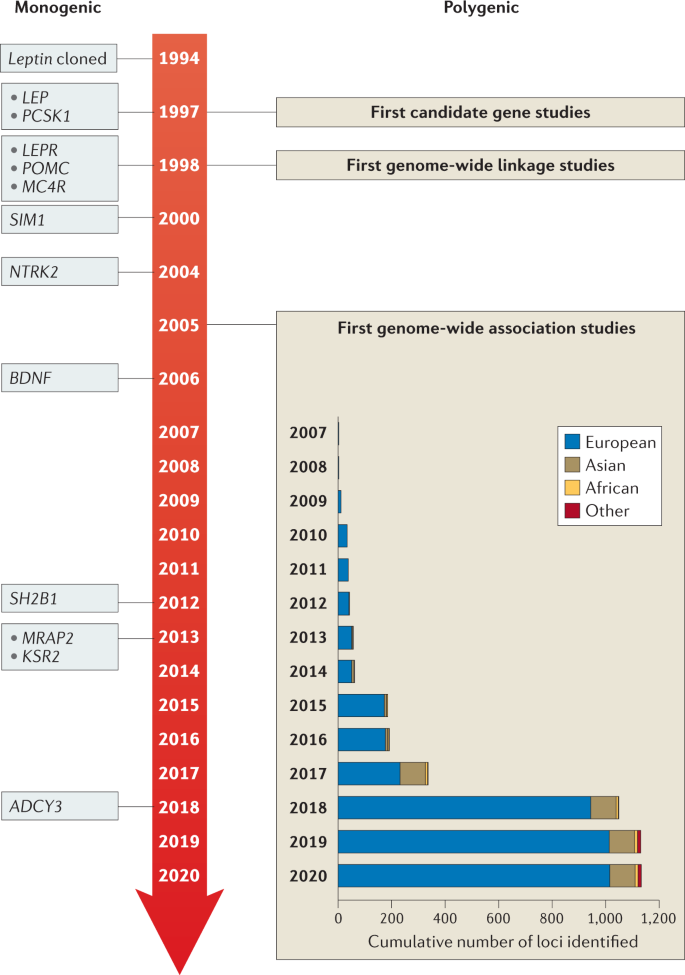
Genes identified for monogenic obesity in a given year are shown on the left. Discoveries made for polygenic obesity are shown on the right, including a cumulative count of newly discovered loci per year and by ancestry. Although candidate gene and genome-wide linkage studies became available in the late 1990s, findings were limited, and these study designs are not as frequently used as genome-wide association studies.
Once the genes for leptin and its receptor were identified, they became candidate genes for human obesity, and in 1997 the first humans with congenital leptin deficiency were identified 21 . This discovery was rapidly followed by the report of humans with mutations in the gene encoding the leptin receptor ( LEPR ) 22 , as well as in genes encoding multiple components of the melanocortin pathway, including PCSK1 (ref. 23 ), MC4R 24 , 25 , 26 and POMC 27 , 28 , 29 , all of which were found to result in severe early-onset obesity (Table 1 ).
Advances in high-throughput DNA sequencing led to candidate gene screening being replaced by WES, an unbiased approach that allows all coding sequences to be screened for mutations. However, it rapidly became clear that, whereas candidate gene studies yielded few mutations, WES identified too many potential obesity-associated variants such that the noise often masked the true causative mutations. However, with improved algorithms to predict the pathogenicity of mutations, as well as a rapidly expanding toolkit of functional assays, it has become easier to filter the likely pathogenic mutations. Several success stories have been reported in which WES has identified novel pathways and genes linked to obesity, such as the class 3 semaphorins (SEMA3A–G), which have been shown to direct the development of certain hypothalamic neurons, including those expressing pro-opiomelanocortin (POMC) 30 (see ‘Other neuronal circuits and molecules linked to severe obesity’).
Most monogenic obesity mutations have been identified in cohorts of patients with severe and early-onset (<10 years old) obesity. Additionally, as monogenic obesity often demonstrates a recessive inheritance pattern 31 , consanguinity in populations has further increased the chance of identifying mutations, owing to greater chances of homozygosity of deleterious mutations 32 . For example, studies have reported that mutations in the genes encoding leptin, LEPR and MC4R explain 30% of cases of severe obesity in children from a consanguineous Pakistani population 33 , and single-gene defects more broadly account for nearly 50% 34 .
Gene discovery for polygenic obesity
The discovery of genes that influence polygenic obesity, which is common in the general population, started off slowly with candidate gene studies and genome-wide linkage studies . The candidate gene approach was first applied in the mid-1990s and aimed to validate genes identified through human and animal models of extreme obesity for a role in common obesity (Fig. 3 ). Common variants in such candidate genes were tested for association with obesity risk, BMI or other body composition traits. Over the subsequent 15 years, hundreds of genes were studied as candidates, but variants in only six ( ADRB3 (ref. 35 ), BDNF 36 , CNR1 (ref. 37 ), MC4R 38 , PCSK1 (ref. 39 ) and PPARG 40 ) showed reproducible association with obesity outcomes. The genome-wide linkage approach made its entrance into the field towards the end of the 1990s (Fig. 3 ). Genome-wide linkage studies rely on the relatedness of individuals and test whether certain chromosomal regions co-segregate with a disease or trait across generations. Even though more than 80 genome-wide linkage studies identified >300 chromosomal loci with suggestive evidence of linkage with obesity traits, few loci were replicated and none was successfully fine-mapped to pinpoint the causal gene or genes 41 . Ultimately, candidate gene and genome-wide linkage studies, constrained by small sample sizes, sparse coverage of genetic variation across the genome and lack of replication, only had a marginal impact on the progression of gene discovery for common obesity outcomes.
However, the pace of gene discovery for common diseases accelerated with the advent of genome-wide association studies (GWAS) (Fig. 3 ). The first GWAS for obesity traits were published in 2007 and identified a cluster of common variants in the first intron of the FTO locus that was convincingly associated with BMI 42 , 43 . Many more GWAS followed and, to date, nearly 60 GWAS have identified more than 1,100 independent loci associated with a range of obesity traits 44 (Supplementary Tables 1 , 2 ).
As sample sizes increase with each consecutive GWAS, the statistical power to identify more loci also increases, in particular for loci that are less common and/or have smaller effects. For example, the first GWAS were relatively small ( n = ~5,000) and identified only the FTO locus 42 , 43 . The BMI-increasing allele of FTO is common, particularly in populations of European ancestry (minor allele frequency (MAF) 40–45%), and has a relatively large effect on BMI (0.35 kg m −2 per allele; equivalent to 1 kg for a person who is 1.7 m tall). Ten years and numerous GWAS later, the most recent GWAS for BMI included nearly 800,000 individuals, identified more than 750 loci, with MAFs as small as 1.6% and per-allele effects as low as 0.04 kg m −2 per allele (equivalent to 120 g for a person who is 1.7 m tall) 45 . Combined, these genome-wide significant loci explained 6% of variation in BMI 45 . Large-scale international collaborations have been formed, such as the Genetic Investigation for Anthropometric Traits (GIANT) consortium , that combine summary statistics of individual GWAS to generate data sets comprising hundreds of thousands of individuals. Furthermore, many GWAS efforts have maximized sample size by focusing on BMI as the primary obesity outcome, an inexpensive and easy-to-obtain measurement that is readily available in most studies. As such, the vast majority of loci have been identified first in GWAS of BMI, but their effects typically transfer to other overall adiposity outcomes.
Even though BMI is widely used, it is considered a crude proxy of overall adiposity because it does not distinguish between lean and fat mass 46 . Therefore, GWAS have been performed for more refined obesity traits, such as body fat percentage 47 , 48 , fat-free mass 49 , imaging-derived adipose tissue 50 , circulating leptin levels 51 and LEPR levels 52 . In addition, two GWAS have focused on persistent healthy thinness, assuming that genes that determine resistance to weight gain may also inform obesity prevention and weight loss maintenance 53 , 54 . Although GWAS of more refined and alternative obesity outcomes are generally much smaller than those for BMI, the phenotypes are often a more accurate representation of body-weight regulation and, as such, the loci identified tend to more often point to relevant biological pathways that underlie obesity.
Almost all GWAS loci for obesity outcomes were first identified in adults. Most of these loci also associate with obesity and/or BMI in children and adolescents, highlighting the fact that the genetic underpinning of obesity is relatively constant across the course of life 55 , 56 , 57 . Similarly to gene discovery for other common diseases, the obesity genetics field has suffered from a strong bias in population representation, with the vast majority of GWAS being performed in populations that are exclusively or predominantly of European ancestry. Nevertheless, some loci have first been discovered in populations of Asian 58 , African 59 , 60 , Hispanic or other ancestry 61 , despite their much smaller sample sizes. Broadly, loci identified in one ancestry demonstrate good transferability (that is, directionally consistent associations) across other ancestries, even though effect sizes and allele frequencies may differ. The modest-to-high genetic correlations across ancestries observed for BMI ( r = 0.78) are consistent with good transferability 62 , but also suggest that ancestry-specific loci remain to be discovered. Besides increasing the sample sizes of GWAS in populations of non-European ancestry, demographic, evolutionary and/or genomic features of specific populations (such as founder, consanguineous or isolated populations) have been leveraged for gene discovery, identifying genetic variants with large effects that are common in the discovery population, such as CREBRF , first identified in Samoan populations, and ADCY3 , first identified in the Greenlandic population, but rare or nonexistent in most others 63 , 64 , 65 , 66 . CREBRF has been shown to play a role in cellular energy storage and use, and may be implicated in cellular and organismal adaptation to nutritional stress 65 . ADCY3 colocalizes with MC4R at the primary cilia of a subset of hypothalamic neurons that have been implicated in body-weight regulation 67 .
GWAS have typically focused on biallelic, common genetic variation (MAF >5%), but have also been used to screen for the role of copy number variants (CNVs) in obesity. So far, only a few CNVs have been identified that have a convincing association with BMI, such as the 1p31.1 45-kb deletion near NEGR1 (ref. 68 ), which encodes a cell-adhesion molecule expressed in the brain 69 ; the 16p12.3 21-kb deletion upstream of GPRC5B 70 , which may modulate insulin secretion 71 ; the 10q11.22 CNV in PPYR1 (also known as NPY4R ) 72 , which encodes a potent anti-obesity agent known to inhibit food intake 73 ; and the 1p21.1 multi-allele CNV encompassing AMY1A 74 , which produces salivary α-amylase, a key enzyme in starch digestion 75 .
To determine the role of other types of variation in obesity, alternative genome-wide screens have been performed. For example, the impact of low-frequency and rare protein-coding variants has been tested using exome sequencing and exome array data 76 , 77 , 78 , 79 . It was speculated that low-frequency (MAF 1–5%) and rare (MAF <1%) variants would have larger effects than common variants, and thus be easier to detect. Nevertheless, even large-scale studies identified only a few robust associations for rare coding variants. For example, exome-wide screening based on array data from more than 400,000 individuals identified p.Tyr35Ter (rs13447324) in MC4R ; p.Arg190Gln (rs139215588) and p.Glu288Gly (rs143430880) in GIPR , which stimulates insulin secretion and mediates fat deposition 80 ; p.Arg95Ter (rs114285050) in GRP151 , which modulates habenular function that controls addiction vulnerability 81 ; and p.Arg769Ter (rs533623778) in PKHD1L1 , which has been involved in cancer development 77 , 78 . A recent study that leveraged WES data for more than 600,000 individuals identified 16 genes for which the burden of rare nonsynonymous variants was associated with BMI, including five brain-expressed G protein-coupled receptors ( CALCR , MC4R , GIPR , GPR151 and GPR75 ) 79 .
As obesity is a complex, multifactorial condition, some GWAS have integrated demographic factors (such as sex and age 82 ) and environmental factors (such as physical activity 83 , diet 84 or smoking 85 ) into their analyses. Despite sample sizes of more than 200,000 individuals, these genome-wide gene-by-environment (G×E) interaction analyses remain challenging and so far only 12 loci have been identified, the effects of which on obesity are attenuated or exacerbated by non-genetic factors. Nevertheless, the G×E interaction between the FTO locus and a healthy lifestyle has been robustly replicated. Specifically, increased physical activity or a healthy diet can attenuate the effect of the FTO locus on obesity risk by 30–40% 86 , 87 .
The increasing availability of large-scale cohorts and biobanks, such as the UK Biobank , the Million Veterans Project , All of Us , Biobank Japan and 23andMe , combined with ongoing work by the GIANT consortium, will boost sample sizes further to easily exceed 4 million participants in meta-analyses, expediting the discovery of many more obesity-associated loci. However, translation of GWAS-identified loci into new biological insights remains a major challenge.
From genes to biology
Despite the difficulties in validating causative mutations and variants, genetic studies into both rare and common obesity over the past two decades have revealed two surprisingly cogent, overarching biological messages: first, the leptin–melanocortin pathway is a key appetitive control circuit 31 , 88 (Fig. 4 ); and second, genes that are either enriched or exclusively expressed within the brain and CNS have a central role in obesity 89 .
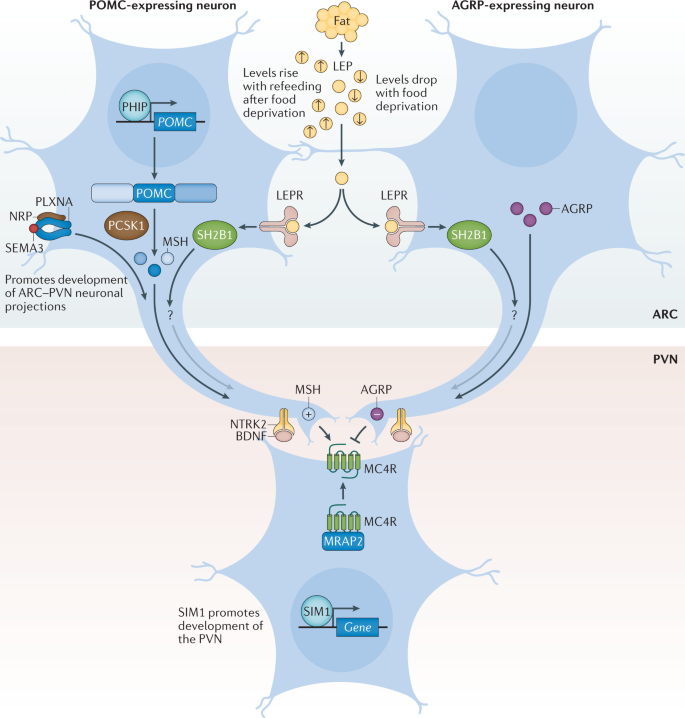
Pro-opiomelanocortin (POMC)-expressing neurons and agouti-related protein (AGRP)-expressing neurons within the arcuate nucleus of the hypothalamus (ARC) act to sense circulating leptin (LEP) levels, which reflect fat mass. These neurons signal to melanocortin 4 receptor (MC4R)-expressing neurons in the paraventricular nucleus of the hypothalamus (PVN), which controls appetite, thus linking long-term energy stores to feeding behaviour. Binding of class 3 semaphorins (SEMA3) to their receptors NRP and PLXNA influences the projection of POMC neurons to the PVN. Binding of brain-derived neurotrophic factor (BDNF) to its receptor neurotrophic receptor tyrosine kinase 2 (NTRK2) is thought to be an effector of leptin-mediated synaptic plasticity of neurons, including those in the ARC and PVN. The transcription factor SIM1 is crucial for the proper development of the PVN. +, agonist; −, antagonist; LEPR, leptin receptor; MRAP2, melanocortin receptor accessory protein 2; MSH, melanocyte-stimulating hormone; SH2B1, SH2B adaptor protein 1.
The leptin–melanocortin pathway and MC4R
Leptin is a key hormone secreted by adipocytes, which circulates at levels in proportion to fat mass 90 . Leptin also responds to acute changes in energy state, as its levels decrease with food deprivation and are restored during re-feeding. Administration of leptin to fasted mice abrogates many of the neuroendocrine consequences of starvation, suggesting that the normal biological role of leptin is to initiate the starvation response 91 . Leptin signals through the LEPR, which exists in several different isoforms. However, obesity-related effects of leptin are predominantly mediated by a long isoform that contains an intracellular domain (LEPRb), which is expressed in various regions of the CNS 90 .
Within the arcuate nucleus (ARC) of the hypothalamus, LEPRb is found on two populations of neurons at the heart of the melanocortin pathway, one of which expresses POMC and the other agouti-related protein (AGRP) 92 (Fig. 4 ). POMC is post-translationally processed by prohormone convertases to produce several biologically active moieties, including β-lipotrophin and β-endorphin, and, crucially, the melanocortin peptides adrenocorticotrophin (ACTH) and α-, β- and γ-melanocyte-stimulating hormone (MSH) 93 . The ARC POMC neurons project to MC4R neurons within the paraventricular nucleus (PVN) where melanocortin peptides signal to decrease food intake 92 . By contrast, AGRP acts as an endogenous antagonist of MC4R to increase food intake 92 , 94 . MC3R is another centrally expressed receptor that binds to both melanocortin peptides and AGRP; however, as mice with targeted deletions in the gene are not obese but instead have altered fat to lean mass ratio, MC3R is less likely to be related to food intake and more likely to be involved in nutrient partitioning 95 , 96 .
We can state with confidence that the fine balance of melanocortinergic agonism and AGRP antagonism of MC4R, in response to peripheral nutritional cues such as leptin, plays a central part in influencing appetitive drive 92 . The genetic evidence clearly supports this contention, with mutations in most genes of the melanocortin pathway resulting in hyperphagia and severe obesity in both humans and mice 31 , 88 . In fact, the vast majority of single-gene disruptions causing severe early-onset obesity in humans fall within this pathway, including LEPR , POMC , AGRP , MCR4R , PCSK1 (ref. 23 ), SH2B1 (ref. 97 ), PHIP 98 , MRAP2 (ref. 99 ) and SIM1 (ref. 100 ) (Fig. 4 ; Table 1 ). Mutations in MC4R in particular, are the most common single-gene defect leading to hyperphagia and obesity. Pathogenic mutations in MC4R are found in up to 5% of cases of severe childhood obesity 101 and up to 0.3% of the general population 101 , 102 . Of note, the degree of receptor dysfunction, as measured by in vitro assays, can predict the amount of food eaten at a test meal by an individual harbouring that particular mutation 101 . Thus MC4R does not act in a binary on/off manner, but as a rheostat; put simply, the melanocortin pathway is a ‘tunable’ system. In addition to regulating food intake, it also regulates food preference, with individuals who carry mutations in MC4R showing a preference for food with higher fat content 103 .
The importance of the melanocortin pathway in regulating feeding behaviour is highlighted by the identification of naturally occurring mutations in pathway genes in a wide range of different species where the appropriate selection pressure has been present (Table 1 ). For example, studies have found that 20–25% of Labrador retrievers, which are known to be more food-motivated than other dog breeds, carry a 14-bp deletion in POMC that disrupts the β-MSH and β-endorphin coding sequences and is associated with greater food motivation and increased body weight 104 . Also, certain breeds of pig have been shown to carry MC4R missense mutations that are associated with fatness, growth and food intake traits 105 . MC4R mutations even contribute to the adaptation and survival of blind Mexican cavefish to the nutrient-poor conditions of their ecosystem 106 .
Other neuronal circuits and molecules linked to severe obesity
It is now clear that in addition to engaging classical neuropeptide–receptor systems within the brain, leptin also rapidly modifies synaptic connections between neurons 107 , and that this structural plasticity is crucial to its downstream functions. One of the ways in which this plasticity is thought to be achieved is via brain-derived neurotrophic factor (BDNF) signalling to its receptor TrkB. BDNF is widely expressed in the CNS where it plays an important part in neuronal development 108 , 109 . In the hippocampus, BDNF contributes to synaptic plasticity and long-term potentiation associated with memory and learning 110 . However, evidence has emerged that implicates BDNF and TrkB in the regulation of mammalian eating behaviour and energy balance 111 . BDNF is downregulated by nutritional deprivation and upregulated by leptin within the ventromedial nucleus (VMN) of the hypothalamus 112 , although this regulation is probably indirect, as very few VMN BDNF neurons express the LEPR 113 (Fig. 4 ) and some evidence indicates that it acts at least in part downstream of melanocortin signalling 112 . Crucially, genetic disruption of BDNF 114 , 115 and TrkB 112 , 116 in both humans and mice results in hyperphagia and severe obesity.
Another group of neuronal proteins important in the development of neuronal circuitry and linked to energy balance are the class 3 semaphorins (SEMA3A–G). A study in humans found that 40 rare loss-of-function variants in SEMA3A–G and their receptors (PLXNA1–4, NRP1 and NRP2) were significantly enriched in 982 individuals with severe obesity compared with 4,449 controls 30 . Disruption of several of these genes in zebrafish caused increased somatic growth and/or adiposity, and experiments with mouse hypothalamic explants suggest that SEMA3 signalling via NRP2 receptors drives the development of POMC projections from the ARC to the PVN 30 . However, given that these results are from a single study, more data are required to confirm the exact role of class 3 semaphorins in energy homeostasis.
Insights from genetic loci linked to common obesity
Unlike candidate gene studies, GWAS make no a priori assumptions about the underlying biology that links genetic variants to a disease of interest. While this agnostic approach allows for new biological insights, the vast majority of GWAS-identified variants map to the non-coding parts of genes or to regions between genes. As such, they do not directly disrupt the protein-coding regions, but instead overlap with regulatory elements that influence expression of genes in close proximity or even over long distances.
However, even if the causative genes are unknown, pathway, tissue and functional enrichment analyses based on the genes located in the GWAS loci can provide insights into potential mechanisms. Since the very first GWAS for BMI 68 , 117 , such analyses have pointed to the CNS being a key player in body-weight regulation, consistent with insights from human and animal models of extreme obesity. Recent analyses that include the latest BMI-associated loci, combined with updated multi-omics databases and advanced computational tools, have further refined these observations. In addition to the hypothalamus and pituitary gland (which are both known appetite regulation sites), other brain areas have been highlighted, including the hippocampus and the limbic system (which are involved in learning, cognition and emotion) and the insula and the substantia nigra (which are related to addiction and reward) 58 , 89 , 118 , 119 . The enrichment of immune-related cells (such as lymphocytes and B cells) and adipose tissue was found to be weaker 58 .
Although enrichment analyses provide preliminary insights into the broad biology represented by genes in the GWAS loci, determining which genes, variants and/or underlying mechanisms are causal has proved an arduous task. For example, the FTO locus, which was identified more than a decade ago and harbours six genes, is the most extensively studied GWAS-identified obesity locus (Fig. 5 ). Despite its highly significant and widely replicated association with obesity 120 , the causal variants and/or genes in the FTO locus have not yet been pinpointed with convincing evidence, and the mechanisms by which the locus affects body weight have not been fully elucidated. Early functional follow-up analyses suggested that FTO itself might be responsible, as Fto deficiency in mice results in a lean phenotype, whereas Fto overexpression is associated with increased body weight 121 , 122 . Studies in mice have suggested that FTO plays a role in cellular nutrient sensing 123 , 124 . Other studies found evidence that FTO influences brain regions that affect appetite, reward processing and incentive motivation by regulating ghrelin levels in humans 125 or by controlling dopaminergic signalling in mice 126 , 127 . In addition, variants in the FTO locus were shown to alter a regulatory element that controls the transcription of Rpgrip1l in mice, a ciliary gene located immediately upstream of Fto 128 , 129 , 130 . Mice with reduced Rpgrip1l activity exhibit hyperphagic obesity, possibly mediated through diminished leptin signalling 128 , 129 , 130 . In recent years, studies in human and animal models have shown that variants in the FTO locus directly interact with the promoter of Irx3 , a gene located 0.5 Mb downstream of FTO . Irx3 -deficient mice were found to exhibit weight loss and increased metabolic rate with browning of white adipose tissue, without changes in physical activity or appetite 131 , 132 . Further in-depth functional characterization showed that rs1421085 in the FTO locus disrupts a conserved binding motif for the transcriptional repressor ARID5B, which leads to a doubling of IRX3 and IRX5 expression during early adipocyte differentiation 132 . The authors argue that increased expression of these genes results in a developmental shift from energy-dissipating beige adipocytes to energy-storing white adipocytes, a fivefold reduction in mitochondrial thermogenesis and increased lipid storage 132 . However, given that multiple studies have shown that the FTO locus is robustly associated with food intake, with no evidence to date linking it to changes in energy expenditure, the relevance of this observation to the actual observed human phenotype still needs to be explored 133 . A recent study reports that the FTO locus affects gene expression in multiple tissues, including adipose tissue and brain, and, more broadly, that the genetic architecture of disease-associated loci may involve extensive pleiotropy and allelic heterogeneity across tissues 134 .
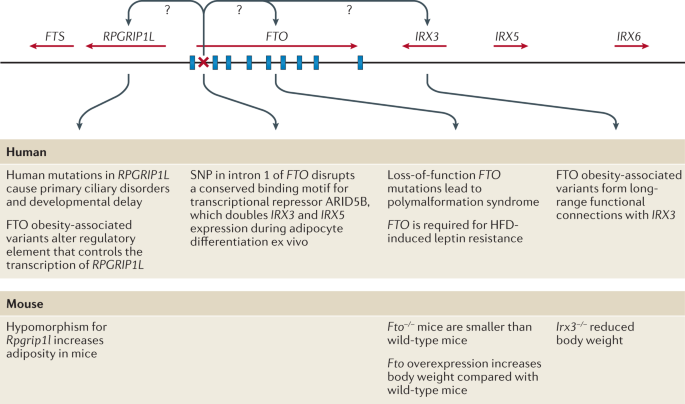
FTO contains nine exons (depicted by blue rectangles) and the body mass index (BMI)-associated SNP identified in genome-wide association studies (depicted by a red ×) maps to intron 1. IRX3 and RPGRIP1L have both been proposed to be the causal genes for obesity within the locus and to act on body weight through distinct mechanisms. HFD, high-fat diet.
Besides the FTO locus, functional follow-up analyses have been performed for only a few obesity-associated GWAS loci. For example, early studies identified a cluster of variants just downstream of TMEM18 (refs 68 , 117 ). TMEM18 encodes a poorly characterized transmembrane protein that is highly conserved across species and widely expressed across tissues, including in several regions of the brain 135 , 136 . Tmem18 deficiency in mice results in a higher body weight owing to increased food intake, whereas Tmem18 overexpression reduces food intake and limits weight gain 136 . A knockdown experiment in Drosophila melanogaster suggests that TMEM18 affects carbohydrate and lipid levels by disrupting insulin and glucagon signalling 137 .
Two other GWAS loci for which functional analyses have been performed are located just upstream of CADM1 (ref. 82 ) and in CADM2 (ref. 70 ), genes that encode cell-adhesion proteins of the immunoglobulin superfamily and mediate synaptic assembly in the CNS 138 . The BMI-increasing alleles at each locus are associated with increased expression of CADM1 and CADM2 in the hypothalamus 139 , 140 . Deficiency of either Cadm1 or Cadm2 in mice results in a lower body weight and increased insulin sensitivity, glucose tolerance and energy expenditure without any change in food intake 139 , 140 . Conversely, increased neuronal expression of either Cadm1 or Cadm2 is associated with elevated body weight 139 , 140 . Furthermore, CADM1 is expressed in POMC neurons and Cadm1 deficiency leads to an increase in the number of excitatory synapses, suggestive of an increased synaptic plasticity 140 . Cadm2 -deficient mice exhibit increased locomotor activity and higher core body temperature 139 .
Another GWAS locus, just upstream of NEGR1 , harbours two deletions associated with increased obesity risk 68 , 117 , 141 . These deletions do not overlap with the coding sequence of NEGR1 , but encompass a conserved transcription factor-binding site for NKX6.1 , a potent transcriptional repressor 68 , 141 . Loss of binding of NKX6.1 leads to higher NEGR1 expression 141 , which is consistent with the observation that BMI-increasing alleles (that is, deletions) at this locus are associated with higher NEGR1 expression in the brain. Similar to CADM1 and CADM2, NEGR1 is a cell-adhesion molecule of the immunoglobulin superfamily that is expressed in several regions of the brain and has been shown to have a role in brain connectivity 69 , 142 , a process believed to be important in obesity 143 . NEGR1 deficiency in mice was shown to result in lower body weight, mainly due to reduced lean mass, mediated by lower food intake 144 . However, two other functional studies, one in mice and one in rats, found that knockdown of Negr1 expression resulted in the opposite phenotype — increased body weight and food intake 145 , 146 . While NEGR1 deficiency in mice was found to impair core behaviours, so far, findings and proposed mechanisms are not fully aligned 69 , 147 , 148 , 149 .
Taken together, functional follow-up analyses for these loci are slowly expanding our understanding of the pathophysiology that drives weight gain. However, many more obesity-associated loci are waiting to be translated into new biological insights. A major hurdle in translating GWAS loci into plausible candidate genes and appropriate paradigms for functional research is the annotation of the associated variants in a locus. Defining the regulatory function of the non-coding variants, identifying their putative effector transcripts and determining their tissues of action remains an ongoing challenge. The advent of high-throughput genome-scale technologies for mapping regulatory elements, combined with comprehensive multi-omics databases, advanced computational tools and the latest genetic engineering and molecular phenotyping approaches, is poised to speed up the translation of GWAS loci into meaningful biology 150 .
Converging results from monogenic and polygenic forms of obesity
Gene discovery is often dichotomized by allele frequency and disease prevalence; that is, mutations are sought for monogenic forms of obesity and common variants for polygenic obesity (Fig. 2 ). However, it is increasingly recognized that monogenic and polygenic forms of obesity are not discrete entities. Instead, they lie on a spectrum and share — at least in part — the same biology. As GWAS have continued to discover more obesity-associated loci, an increasing number of these loci harbour genes that were first identified for extreme and early-onset obesity in humans or animal models, including MC4R 151 , 152 , BDNF 117 , SH2B1 (refs 68 , 117 ), POMC 70 , LEP 51 , 153 , LEPR 52 , 154 , NPY 155 , SIM1 (ref. 155 ), NTRK2 (ref. 58 ), PCSK1 (ref. 154 ) and KSR2 (ref. 77 ). In fact, most of these genes encode components of the leptin–melanocortin and BDNF–TrkB signalling pathways (Table 1 ). Thus, whereas genetic disruption of components of these pathways results in severe obesity, genetic variants in or near these same genes that have more subtle effects on their expression will influence where an individual might sit in the normal distribution of BMI.
Although most genes have been first identified for extreme forms of obesity, a locus harbouring ADCY3 was first identified in GWAS for common obesity 77 , and ADCY3 was subsequently confirmed as having a role in extreme obesity 63 , 64 . ADCY3 encodes an adenylate cyclase that catalyses the synthesis of cAMP, an important second messenger in signalling pathways. There is some evidence that ADCY3 (adenylate cyclase) colocalizes with MC4R at the primary cilia of PVN neurons 67 and that cilia are required specifically on MC4R-expressing neurons for the control of energy homeostasis 156 . In mice, disruption of Adcy3 or Mc4r in the cilia of these neurons impairs melanocortin signalling, resulting in hyperphagia and obesity 67 .
As more GWAS loci are reported, we expect that findings across different lines of obesity research will continue to converge, providing accumulating evidence for new biology.
From genes to clinical care
Genetic insights from gene discovery efforts are increasingly being used in the context of precision medicine in ways that directly affect health. Knowing a patient’s genotype may enable a more precise diagnosis of the type of obesity, which in turn allows the prescription of personalized treatment or prevention strategies. Furthermore, knowing an individual’s genetic susceptibility to obesity early in life may help to more accurately predict those most at risk of gaining weight in the future.
Use of genotype information in treatment of obesity
When a disease is caused by a single mutation and the environmental contribution is limited, as is the case for some forms of extreme and early-onset obesity, a genetic test can be instrumental in correctly diagnosing patients. Although no standard genetic testing panel is currently available for extreme and early-onset obesity, some clinics, research centres and pharmaceutical companies sequence well-known candidate genes to identify the functional mutation that may be the cause of a patient’s excess body weight. Such a genetic diagnosis can lessen the feelings of guilt and blame for the patient, and alleviate social stigma and discrimination. Importantly, a genetic diagnosis can inform disease prognosis and, in some cases, it will determine treatment. To date, there are two treatments for obesity that are tailored to patient genotype.
The prototype of genotype-informed treatment for obesity is the administration of recombinant human leptin in patients who are leptin-deficient owing to mutations in the LEP gene 157 , 158 . Although congenital leptin deficiency is exceptionally rare (only 63 cases have been reported to date 28 ), leptin replacement therapy has been remarkably beneficial for these patients by substantially reducing food intake, body weight and fat mass, and normalizing endocrine function 157 , 158 . It has literally transformed their lives.
The second genotype-informed treatment for obesity is setmelanotide, a selective MC4R agonist that was recently approved by the FDA for rare monogenic obesity conditions including LEPR, PCSK1 and POMC deficiency 159 . Setmelanotide acts as a substitute for the absent MSH in patients with POMC deficiency owing to mutations in POMC or PCSK1 , and in patients with LEPR deficiency owing to mutations in LEPR , which is essential for POMC function 160 , 161 , 162 . Daily subcutaneous injection of setmelanotide results in substantial weight loss and in reduction of hunger 160 , 161 , 162 . After a 1-year treatment with setmelanotide in phase III trials, patients with POMC deficiency lost on average 25.6% of their initial weight, with 80% of patients achieving at least a 10% weight loss 162 . The adverse effects of setmelanotide treatment are minor, and include hyperpigmentation, nausea and/or vomiting, penile erection and injection site reactions. Weight loss in patients with LEPR deficiency was less pronounced; on average, they lost 12.5% of their initial weight, with only 45% of patients achieving at least a 10% weight loss 162 . The difference in weight loss between the two patient groups may be because POMC deficiency directly affects the production of MC4R ligands (α-MSH and β-MSH), whereas LEPR deficiency affects signalling upstream of POMC 162 . As such, setmelanotide may be able to completely restore MC4R signalling in POMC deficiency, but only partially in LEPR deficiency. Even though the average weight loss in POMC-deficient patients was twice that in LEPR-deficient patients, the reduction in hunger was substantially larger in LEPR-deficient patients (−43.7%) than in POMC-deficient patients (−27.1%) 162 . The reasons for the discrepancy between weight loss and reduction in hunger remain to be studied in greater depth. It has been estimated that in the USA, >12,800 individuals carry mutations in the melanocortin pathway for whom setmelanotide may be more effective for weight loss than any other treatment 163 . Although 12,800 carriers represent only a fraction (0.004%) of the adult population in the USA, and not all of these mutation carriers are overweight or obese, for the patients for whom setmelanotide is effective, it may end a lifelong battle to lose weight 163 . In patients without genetic defects, neither setmelanotide nor leptin administration have, to date, demonstrated a substantial effect on weight loss 164 , 165 .
These two genotype-informed treatments show how insight into the underlying biological mechanisms can guide the development of molecules and medications that restore impaired pathways, at least in monogenic forms of obesity caused by deficiency of one protein. Nevertheless, there remain substantial obstacles in the transition from conventional to precision medicine for monogenic obesity, which would require the adoption of systematic WES for individuals suspected to be carriers of deleterious mutations, and eventually even standardized screening at birth. We are clearly a long way from such a scenario at present.
Use of genotype information in prediction of obesity
As more variants are being discovered for common obesity, there is a growing expectation that genetic information will soon be used to identify individuals at risk of obesity. Knowing a person’s genetic susceptibility would allow for a more accurate prediction of who is at risk of gaining weight and give an opportunity to intervene earlier to prevent obesity more effectively. Genetic susceptibility to complex disease, including obesity, is assessed using a polygenic score (PGS). PGSs to assess obesity susceptibility are based on GWAS for BMI (PGS BMI ), the latest of which includes data on more than 2 million variants and explains 8.4% of the variation in BMI 166 . The average BMI of individuals with a high PGS BMI (top decile) is 2.9 kg m −2 (equivalent to 8 kg in body weight) higher and their odds of severe obesity (BMI ≥40 kg m −2 ) is 4.2-fold higher than those with a lower PGS BMI (lowest nine deciles) 166 .
Despite these strong associations with BMI and obesity, the predictive performance of the PGS BMI is weak, which is unsurprising given its limited explained variance. For example, using the same PGS BMI and data from the UK Biobank, we estimate that the area under the receiver operating characteristic curve (AUC ROC ) is only 0.64 to predict obesity. This means that the probability that an individual with obesity has a higher PGS BMI than an individual without obesity is 0.64. However, for a PGS to have clinical utility, the AUC ROC needs to be much higher (>0.80). In addition, we calculated the extent to which a PGS BMI ≥90th percentile correctly classifies individuals with obesity (Fig. 6 ). We found that such a predictive test (PGS BMI ≥90th percentile) has a positive predictive value of 0.43, meaning that of those who were predicted to develop obesity, only 43% actually developed obesity. Its sensitivity is 0.19, which means that of the individuals who developed obesity, only 19% had been correctly classified by the PGS BMI . Given that the current treatment options for obesity are low risk, or even generally beneficial, the high false-positive rate is less concerning than the low sensitivity, as some at-risk individuals may miss the opportunity for early prevention.
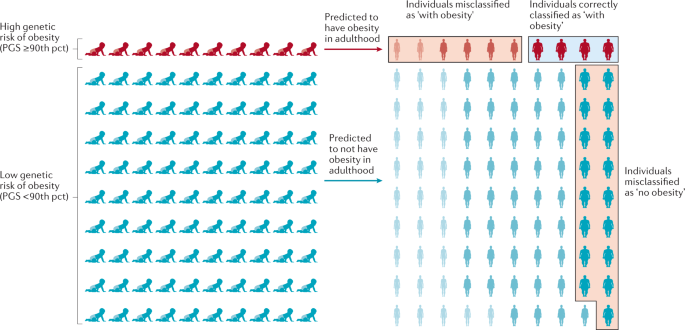
The outcome is illustrated for a polygenic score (PGS) that assumes that individuals with a score in the highest decile (≥90th percentile (pct)) will develop obesity, has a positive predictive value of 0.4 and a sensitivity of 0.19. Of ten individuals with a high score classified by the PGS as ‘with obesity’, four will be classified correctly but the other six will be misclassified and will not develop obesity — a positive predictive value of 0.4. Likewise, 17 of the 90 individuals with a score <90th pct who are predicted to not develop obesity, will develop obesity. Thus, only four of the 21 individuals who developed obesity were correctly classified by the PGS — a sensitivity of 0.19. Misclassified individuals are indicated by the red boxes, individuals correctly classified as ‘with obesity’ are indicated by a blue box. Adapted with permission from ref. 170 , Elsevier.
Thus, the current PGS BMI has a high rate of misclassification and does not reliably predict who is at risk of developing obesity and who is not. The predictive ability of PGSs are expected to improve as GWAS increase in sample size and algorithms to calculate the scores become more refined. Nevertheless, given the importance of socio-demographic, lifestyle and clinical risk factors in the aetiology of obesity, it is unlikely that a PGS BMI will ever be able to accurately predict obesity on its own. Instead, effective prediction models will have to include genetic and non-genetic factors, including a broad spectrum of demographic, environmental, clinical and possibly molecular markers, as well.
Conclusions and future perspectives
What initially began as two apparently distinct approaches, one studying rare Mendelian causes of extreme obesity, and the other exploring complex polygenic influences of population body-weight distribution, have eventually converged on the central role of the brain in regulating body weight. In particular, both approaches have highlighted the roles of the leptin–melanocortin pathway and TrkB–BDNF signalling. Perhaps it seems obvious now, but it was by no means certain that, just because genetic disruption of a pathway resulted in a severe phenotype, polymorphisms within that same pathway would produce a more subtle and nuanced result.
The GWAS approach is hypothesis-free, with the promise to reveal new genes that point to new biology and pathways. However, for the vast majority of the >1,000 GWAS-identified loci, we do not know which genes are causal, what cells, tissues and organs they act in to affect body weight, and we do not understand the underlying mechanisms. The translation from variant to function is a well-known challenge 167 , but with increasing availability of new omics data, high-throughput technologies and advanced analytical approaches, there is an unprecedented opportunity to speed up the translation of hundreds of GWAS loci.
Sample size remains a major driver for gene discovery. In an ongoing collaboration that combines data from more than 3 million individuals of diverse ancestry from the GIANT consortium, the UK Biobank and 23andMe, the number of BMI-associated GWAS loci is set to double. Also, a recent WES effort of more than 640,000 individuals has demonstrated that rare mutations are discoverable when sample sizes are sufficiently large 79 . However, alternative study designs, a focus on more refined phenotypes or a focus on population subgroups (that is, more homogeneous groups of individuals with similar outcomes) could further add to gene discovery.
Translation of only a few dozen of the GWAS-identified loci could tremendously improve our insights into the biology of obesity and possibly reveal new therapeutic targets. It would also take us a little closer to the ‘holy grail’ — the ability to move away from a failed ‘one-size-fits-all’ strategy, and towards true precision medicine for obesity, metabolic disease and other diet-related illnesses.
GBD 2015 Obesity Collaborators. Health effects of overweight and obesity in 195 countries over 25 years. N. Engl. J. Med. 377 , 13–27 (2017).
Article Google Scholar
Must, A. et al. The disease burden associated with overweight and obesity. JAMA 282 , 1523–1529 (1999).
Article CAS PubMed Google Scholar
Fontaine, K. R. & Barofsky, I. Obesity and health-related quality of life. Obes. Rev. 2 , 173–182 (2001).
Bhattacharya, I., Ghayor, C., Perez Dominguez, A. & Weber, F. E. From influenza virus to novel corona virus (SARS-CoV-2)—the contribution of obesity. Front. Endocrinol. 11 , 556962 (2020).
Petrilli, C. M. et al. Factors associated with hospital admission and critical illness among 5279 people with coronavirus disease 2019 in New York City: prospective cohort study. BMJ 369 , m1966 (2020).
Article PubMed PubMed Central Google Scholar
Cummings, M. J. et al. Epidemiology, clinical course, and outcomes of critically ill adults with COVID-19 in New York City: a prospective cohort study. Lancet 395 , 1763–1770 (2020).
Article CAS PubMed PubMed Central Google Scholar
Zhao, X. et al. Obesity increases the severity and mortality of influenza and COVID-19: a systematic review and meta-analysis. Front. Endocrinol. 11 , 595109 (2020).
Abarca-Gómez, L. et al. Worldwide trends in body-mass index, underweight, overweight, and obesity from 1975 to 2016: a pooled analysis of 2416 population-based measurement studies in 128.9 million children, adolescents, and adults. Lancet 390 , 2627–2642 (2017).
Maes, H. H., Neale, M. C. & Eaves, L. J. Genetic and environmental factors in relative body weight and human obesity. Behav. Genet. 27 , 325–351 (1997).
Elks, C. E. et al. Variability in the heritability of body mass index: a systematic review and meta-regression. Front. Endocrinol. 3 , 29 (2012). This paper reports a large-scale meta-analysis of heritability data of twin and family studies .
Chami, N., Preuss, M., Walker, R. W., Moscati, A. & Loos, R. J. F. The role of polygenic susceptibility to obesity among carriers of pathogenic mutations in MC4R in the UK Biobank population. PLoS Med. 17 , e1003196 (2020). This study shows that the effect of MC4R mutations on BMI and obesity risk is attenuated by the polygenic background .
Kaur, Y., de Souza, R. J., Gibson, W. T. & Meyre, D. A systematic review of genetic syndromes with obesity. Obes. Rev. 18 , 603–634 (2017).
Ingalls, A. M., Dickie, M. M. & Snell, G. D. Obese, a new mutation in the house mouse. J. Hered. 41 , 317–318 (1950).
Hummel, K. P., Dickie, M. M. & Coleman, D. L. Diabetes, a new mutation in the mouse. Science 153 , 1127–1128 (1966).
Zhang, Y. et al. Positional cloning of the mouse obese gene and its human homologue. Nature 372 , 425–432 (1994). This paper reports the cloning of leptin, which provided the first molecular evidence that feeding and regulation of body weight had a hormonal basis .
Article CAS Google Scholar
Chen, H. et al. Evidence that the diabetes gene encodes the leptin receptor: identification of a mutation in the leptin receptor gene in db / db mice. Cell 84 , 491–495 (1996). This paper reports the cloning of the LEPR and the localization of its signalling version, which highlights the role of the brain in the control of appetitive behaviour .
Bultman, S. J., Michaud, E. J. & Woychik, R. P. Molecular characterization of the mouse agouti locus. Cell 71 , 1195–1204 (1992).
Miller, M. W. et al. Cloning of the mouse agouti gene predicts a secreted protein ubiquitously expressed in mice carrying the lethal yellow mutation. Genes Dev. 7 , 454–467 (1993).
Lu, D. et al. Agouti protein is an antagonist of the melanocyte-stimulating-hormone receptor. Nature 371 , 799–802 (1994).
Fan, W., Boston, B. A., Kesterson, R. A., Hruby, V. J. & Cone, R. D. Role of melanocortinergic neurons in feeding and the agouti obesity syndrome. Nature 385 , 165–168 (1997).
Montague, C. T. et al. Congenital leptin deficiency is associated with severe early-onset obesity in humans. Nature 387 , 903–908 (1997). This work demonstrates that leptin signalling is also relevant in the human context, and provides early evidence, together with mutations in PCSK1 , of a monogenic cause of severe human obesity .
Clement, K. et al. A mutation in the human leptin receptor gene causes obesity and pituitary dysfunction. Nature 392 , 398–401 (1998). This work confirms the observations from mice about leptin signalling to the brain .
Jackson, R. S. et al. Obesity and impaired prohormone processing associated with mutations in the human prohormone convertase 1 gene. Nat. Genet. 16 , 303–306 (1997). This study, together with mutations in the leptin gene, provides early evidence of a monogenic cause of severe human obesity, and also highlights the role of the nascent melanocortin pathway in body-weight regulation .
Huszar, D. et al. Targeted disruption of the melanocortin-4 receptor results in obesity in mice. Cell 88 , 131–141 (1997).
Yeo, G. S. et al. A frameshift mutation in MC4R associated with dominantly inherited human obesity. Nat. Genet. 20 , 111–112 (1998).
Vaisse, C., Clement, K., Guy-Grand, B. & Froguel, P. A frameshift mutation in MC4R is associated with a dominant form of obesity. Nat. Genet. 20 , 113–114 (1998). This paper, together with Yeo et al. (1998), shows that heterozygous mutations in MC4R result in severe human obesity, establishing the role of the central melanocortin pathway in regulating human appetitive behaviour .
Krude, H. et al. Severe early-onset obesity, adrenal insufficiency and red hair pigmentation caused by POMC mutations in humans. Nat. Genet. 19 , 155–157 (1998). This paper provides direct evidence that melanocortin peptides play a key role in the regulation of energy homeostasis .
Yaswen, L., Diehl, N., Brennan, M. B. & Hochgeschwender, U. Obesity in the mouse model of pro-opiomelanocortin deficiency responds to peripheral melanocortin. Nat. Med. 5 , 1066–1070 (1999).
Challis, B. G. et al. Mice lacking pro-opiomelanocortin are sensitive to high-fat feeding but respond normally to the acute anorectic effects of peptide-YY(3-36). Proc. Natl Acad. Sci. USA 101 , 4695–4700 (2004).
van der Klaauw, A. A. et al. Human semaphorin 3 variants link melanocortin circuit development and energy balance. Cell 176 , 729–742.e18 (2019).
Farooqi, S. & O’Rahilly, S. Genetics of obesity in humans. Endocr. Rev. 27 , 710–718 (2006).
Saeed, S., Arslan, M. & Froguel, P. Genetics of obesity in consanguineous populations: toward precision medicine and the discovery of novel obesity genes. Obesity 26 , 474–484 (2018).
Article PubMed Google Scholar
Saeed, S. et al. Genetic variants in LEP, LEPR, and MC4R explain 30% of severe obesity in children from a consanguineous population. Obesity 23 , 1687–1695 (2015).
Saeed, S. et al. Genetic causes of severe childhood obesity: a remarkably high prevalence in an inbred population of Pakistan. Diabetes 69 , 1424–1438 (2020).
Kurokawa, N. et al. The ADRB3 Trp64Arg variant and BMI: a meta-analysis of 44 833 individuals. Int. J. Obes. 32 , 1240–1249 (2008).
Shugart, Y. Y. et al. Two British women studies replicated the association between the Val66Met polymorphism in the brain-derived neurotrophic factor (BDNF) and BMI. Eur. J. Hum. Genet. 17 , 1050–1055 (2009).
Benzinou, M. et al. Endocannabinoid receptor 1 gene variations increase risk for obesity and modulate body mass index in European populations. Hum. Mol. Genet. 17 , 1916–1921 (2008).
Wang, D. et al. Association of the MC4R V103I polymorphism with obesity: a Chinese case–control study and meta-analysis in 55,195 individuals. Obesity 18 , 573–579 (2010).
Nead, K. T. et al. Contribution of common non-synonymous variants in PCSK1 to body mass index variation and risk of obesity: a systematic review and meta-analysis with evidence from up to 331 175 individuals. Hum. Mol. Genet. 24 , 3582–3594 (2015).
Tonjes, A., Scholz, M., Loeffler, M. & Stumvoll, M. Association of Pro12Ala polymorphism in peroxisome proliferator-activated receptor gamma with pre-diabetic phenotypes: meta-analysis of 57 studies on nondiabetic individuals. Diabetes Care 29 , 2489–2497 (2006).
Rankinen, T. et al. The human obesity gene map: the 2005 update. Obesity 14 , 529–644 (2006).
Frayling, T. M. et al. A common variant in the FTO gene is associated with body mass index and predisposes to childhood and adult obesity. Science 316 , 889–894 (2007). This paper describes the first GWAS (for type 2 diabetes) to identify a locus ( FTO ) robustly associated with BMI .
Scuteri, A. et al. Genome-wide association scan shows genetic variants in the FTO gene are associated with obesity-related traits. PLoS Genet. 3 , e115 (2007).
Buniello, A. et al. The NHGRI-EBI GWAS Catalog of published genome-wide association studies, targeted arrays and summary statistics 2019. Nucleic Acids Res. 47 , D1005–D1012 (2019).
Yengo, L. et al. Meta-analysis of genome-wide association studies for height and body mass index in approximately 700 000 individuals of European ancestry. Hum. Mol. Genet. 27 , 3641–3649 (2018).
Javed, A. et al. Diagnostic performance of body mass index to identify obesity as defined by body adiposity in children and adolescents: a systematic review and meta-analysis. Pediatr. Obes. 10 , 234–244 (2015).
Kilpelainen, T. O. et al. Genetic variation near IRS1 associates with reduced adiposity and an impaired metabolic profile. Nat. Genet. 43 , 753–760 (2011).
Lu, Y. et al. New loci for body fat percentage reveal link between adiposity and cardiometabolic disease risk. Nat. Commun. 7 , 10495 (2016).
Zillikens, M. C. et al. Large meta-analysis of genome-wide association studies identifies five loci for lean body mass. Nat. Commun. 8 , 80 (2017).
Chu, A. Y. et al. Multiethnic genome-wide meta-analysis of ectopic fat depots identifies loci associated with adipocyte development and differentiation. Nat. Genet. 49 , 125–130 (2017).
Kilpelainen, T. O. et al. Genome-wide meta-analysis uncovers novel loci influencing circulating leptin levels. Nat. Commun. 7 , 10494 (2016).
Sun, Q. et al. Genome-wide association study identifies polymorphisms in LEPR as determinants of plasma soluble leptin receptor levels. Hum. Mol. Genet. 19 , 1846–1855 (2010).
Riveros-McKay, F. et al. Genetic architecture of human thinness compared to severe obesity. PLoS Genet. 15 , e1007603 (2019).
Orthofer, M. et al. Identification of ALK in thinness. Cell 181 , 1246–1262.e22 (2020).
Bradfield, J. P. et al. A trans-ancestral meta-analysis of genome-wide association studies reveals loci associated with childhood obesity. Hum. Mol. Genet. 28 , 3327–3338 (2019).
Felix, J. F. et al. Genome-wide association analysis identifies three new susceptibility loci for childhood body mass index. Hum. Mol. Genet. 25 , 389–403 (2016).
Vogelezang, S. et al. Novel loci for childhood body mass index and shared heritability with adult cardiometabolic traits. PLoS Genet. 16 , e1008718 (2020).
Akiyama, M. et al. Genome-wide association study identifies 112 new loci for body mass index in the Japanese population. Nat. Genet. 49 , 1458–1467 (2017).
Ng, M. C. Y. et al. Discovery and fine-mapping of adiposity loci using high density imputation of genome-wide association studies in individuals of African ancestry: African Ancestry Anthropometry Genetics Consortium. PLoS Genet. 13 , e1006719 (2017).
Gurdasani, D. et al. Uganda genome resource enables insights into population history and genomic discovery in Africa. Cell 179 , 984–1002.e36 (2019).
Wojcik, G. L. et al. Genetic analyses of diverse populations improves discovery for complex traits. Nature 570 , 514–518 (2019).
Martin, A. R. et al. Clinical use of current polygenic risk scores may exacerbate health disparities. Nat. Genet. 51 , 584–591 (2019).
Grarup, N. et al. Loss-of-function variants in ADCY3 increase risk of obesity and type 2 diabetes. Nat. Genet. 50 , 172–174 (2018).
Saeed, S. et al. Loss-of-function mutations in ADCY3 cause monogenic severe obesity. Nat. Genet. 50 , 175–179 (2018).
Minster, R. L. et al. A thrifty variant in CREBRF strongly influences body mass index in Samoans. Nat. Genet. 48 , 1049–1054 (2016).
Andersen, M. K. et al. The derived allele of a novel intergenic variant at chromosome 11 associates with lower body mass index and a favorable metabolic phenotype in Greenlanders. PLoS Genet. 16 , e1008544 (2020).
Siljee, J. E. et al. Subcellular localization of MC4R with ADCY3 at neuronal primary cilia underlies a common pathway for genetic predisposition to obesity. Nat. Genet. 50 , 180–185 (2018).
Willer, C. J. et al. Six new loci associated with body mass index highlight a neuronal influence on body weight regulation. Nat. Genet. 41 , 25–34 (2009).
Singh, K. et al. Neural cell adhesion molecule Negr1 deficiency in mouse results in structural brain endophenotypes and behavioral deviations related to psychiatric disorders. Sci. Rep. 9 , 5457 (2019).
Speliotes, E. K. et al. Association analyses of 249,796 individuals reveal 18 new loci associated with body mass index. Nat. Genet. 42 , 937–948 (2010).
Soni, A., Amisten, S., Rorsman, P. & Salehi, A. GPRC5B a putative glutamate-receptor candidate is negative modulator of insulin secretion. Biochem. Biophys. Res. Commun. 441 , 643–648 (2013).
Jarick, I. et al. Novel common copy number variation for early onset extreme obesity on chromosome 11q11 identified by a genome-wide analysis. Hum. Mol. Genet. 20 , 840–852 (2011).
Sainsbury, A. et al. Synergistic effects of Y2 and Y4 receptors on adiposity and bone mass revealed in double knockout mice. Mol. Cell Biol. 23 , 5225–5233 (2003).
Falchi, M. et al. Low copy number of the salivary amylase gene predisposes to obesity. Nat. Genet. 46 , 492–497 (2014).
Meisler, M. H. & Ting, C. N. The remarkable evolutionary history of the human amylase genes. Crit. Rev. Oral Biol. Med. 4 , 503–509 (1993).
Hendricks, A. E. et al. Rare variant analysis of human and rodent obesity genes in individuals with severe childhood obesity. Sci. Rep. 7 , 4394 (2017).
Turcot, V. et al. Protein-altering variants associated with body mass index implicate pathways that control energy intake and expenditure in obesity. Nat. Genet. 50 , 26–41 (2018). This large-scale exome-wide discovery study reports the use of array data to identify rare coding variations associated with BMI .
Emdin, C. A. et al. Analysis of predicted loss-of-function variants in UK Biobank identifies variants protective for disease. Nat. Commun. 9 , 1613 (2018).
Akbari, P. et al. Sequencing of 640,000 exomes identifies GPR75 variants associated with protection from obesity. Science 373 , eabf8683 (2021). This large-scale exome-wide discovery study reports the use of WES data to identify mutations associated with BMI .
Baggio, L. L. & Drucker, D. J. Biology of incretins: GLP-1 and GIP. Gastroenterology 132 , 2131–2157 (2007).
Antolin-Fontes, B. et al. The habenular G-protein-coupled receptor 151 regulates synaptic plasticity and nicotine intake. Proc. Natl Acad. Sci. USA 117 , 5502–5509 (2020).
Winkler, T. W. et al. The influence of age and sex on genetic associations with adult body size and shape: a large-scale genome-wide interaction study. PLoS Genet. 11 , e1005378 (2015).
Graff, M. et al. Genome-wide physical activity interactions in adiposity — a meta-analysis of 200,452 adults. PLoS Genet. 13 , e1006528 (2017).
Smith, C. E. et al. Genome-wide interactions with dairy intake for body mass index in adults of European descent. Mol. Nutr. Food Res. 62 , 1700347 (2018).
Justice, A. E. et al. Genome-wide meta-analysis of 241,258 adults accounting for smoking behaviour identifies novel loci for obesity traits. Nat. Commun. 8 , 14977 (2017).
Qi, Q. et al. Fried food consumption, genetic risk, and body mass index: gene–diet interaction analysis in three US cohort studies. BMJ 348 , g1610 (2014).
Kilpelainen, T. O. et al. Physical activity attenuates the influence of FTO variants on obesity risk: a meta-analysis of 218,166 adults and 19,268 children. PLoS Med. 8 , e1001116 (2011).
Yeo, G. S. H. Genetics of obesity: can an old dog teach us new tricks? Diabetologia 60 , 778–783 (2017).
Locke, A. E. et al. Genetic studies of body mass index yield new insights for obesity biology. Nature 518 , 197–206 (2015). This large-scale GWAS for BMI shows that BMI-associated loci frequently localize in or near genes that act in the brain .
Friedman, J. M. & Halaas, J. L. Leptin and the regulation of body weight in mammals. Nature 395 , 763–770 (1998).
Ahima, R. S. et al. Role of leptin in the neuroendocrine response to fasting. Nature 382 , 250–252 (1996).
Cowley, M. A. et al. Integration of NPY, AGRP, and melanocortin signals in the hypothalamic paraventricular nucleus: evidence of a cellular basis for the adipostat. Neuron 24 , 155–163 (1999).
Bertagna, X. Proopiomelanocortin-derived peptides. Endocrinol. Metab. Clin. North Am. 23 , 467–485 (1994).
Ollmann, M. M. et al. Antagonism of central melanocortin receptors in vitro and in vivo by agouti-related protein. Science 278 , 135–138 (1997).
Butler, A. A. et al. A unique metabolic syndrome causes obesity in the melanocortin-3 receptor-deficient mouse. Endocrinology 141 , 3518–3521 (2000).
Chen, A. S. et al. Inactivation of the mouse melanocortin-3 receptor results in increased fat mass and reduced lean body mass. Nat. Genet. 26 , 97–102 (2000).
Doche, M. E. et al. Human SH2B1 mutations are associated with maladaptive behaviors and obesity. J. Clin. Invest. 122 , 4732–4736 (2012).
Marenne, G. et al. Exome sequencing identifies genes and gene sets contributing to severe childhood obesity, linking PHIP variants to repressed POMC transcription. Cell Metab. 31 , 1107–1119.e12 (2020).
Asai, M. et al. Loss of function of the melanocortin 2 receptor accessory protein 2 is associated with mammalian obesity. Science 341 , 275–278 (2013).
Michaud, J. L. et al. Sim1 haploinsufficiency causes hyperphagia, obesity and reduction of the paraventricular nucleus of the hypothalamus. Hum. Mol. Genet. 10 , 1465–1473 (2001).
Farooqi, I. S. et al. Clinical spectrum of obesity and mutations in the melanocortin 4 receptor gene. N. Engl. J. Med. 348 , 1085–1095 (2003).
Wade, K. H. et al. Loss-of-function mutations in the melanocortin 4 receptor in a UK birth cohort. Nat. Med. 27 , 1088–1096 (2021). This paper establishes the frequency of loss-of-function mutations in MC4R to be 0.3%, much more common than previously appreciated .
van der Klaauw, A. et al. Role of melanocortin signalling in the preference for dietary macronutrients in human beings. Lancet 385 , S12 (2015).
Raffan, E. et al. A deletion in the canine POMC gene is associated with weight and appetite in obesity-prone Labrador retriever dogs. Cell Metab. 23 , 893–900 (2016).
Kim, K. S., Larsen, N., Short, T., Plastow, G. & Rothschild, M. F. A missense variant of the porcine melanocortin-4 receptor (MC4R) gene is associated with fatness, growth, and feed intake traits. Mamm. Genome 11 , 131–135 (2000).
Aspiras, A. C., Rohner, N., Martineau, B., Borowsky, R. L. & Tabin, C. J. Melanocortin 4 receptor mutations contribute to the adaptation of cavefish to nutrient-poor conditions. Proc. Natl Acad. Sci. USA 112 , 9668–9673 (2015).
Pinto, S. et al. Rapid rewiring of arcuate nucleus feeding circuits by leptin. Science 304 , 110–115 (2004).
Davies, A. M. The role of neurotrophins in the developing nervous system. J. Neurobiol. 25 , 1334–1348 (1994).
McAllister, A. K., Katz, L. C. & Lo, D. C. Opposing roles for endogenous BDNF and NT-3 in regulating cortical dendritic growth. Neuron 18 , 767–778 (1997).
Korte, M. et al. Hippocampal long-term potentiation is impaired in mice lacking brain-derived neurotrophic factor. Proc. Natl Acad. Sci. USA 92 , 8856–8860 (1995).
Kernie, S. G., Liebl, D. J. & Parada, L. F. BDNF regulates eating behavior and locomotor activity in mice. EMBO J. 19 , 1290–1300 (2000).
Xu, B. et al. Brain-derived neurotrophic factor regulates energy balance downstream of melanocortin-4 receptor. Nat. Neurosci. 6 , 736–742 (2003).
Xu, B. & Xie, X. Neurotrophic factor control of satiety and body weight. Nat. Rev. Neurosci. 17 , 282–292 (2016).
Rios, M. et al. Conditional deletion of brain-derived neurotrophic factor in the postnatal brain leads to obesity and hyperactivity. Mol. Endocrinol. 15 , 1748–1757 (2001).
Gray, J. et al. Hyperphagia, severe obesity, impaired cognitive function, and hyperactivity associated with functional loss of one copy of the brain-derived neurotrophic factor (BDNF) gene. Diabetes 55 , 3366–3371 (2006).
Yeo, G. S. et al. A de novo mutation affecting human TrkB associated with severe obesity and developmental delay. Nat. Neurosci. 7 , 1187–1189 (2004).
Thorleifsson, G. et al. Genome-wide association yields new sequence variants at seven loci that associate with measures of obesity. Nat. Genet. 41 , 18–24 (2009).
Finucane, H. K. et al. Heritability enrichment of specifically expressed genes identifies disease-relevant tissues and cell types. Nat. Genet. 50 , 621–629 (2018).
Ndiaye, F. K. et al. The expression of genes in top obesity-associated loci is enriched in insula and substantia nigra brain regions involved in addiction and reward. Int. J. Obes. 44 , 539–543 (2020).
Loos, R. J. & Yeo, G. S. The bigger picture of FTO: the first GWAS-identified obesity gene. Nat. Rev. Endocrinol. 10 , 51–61 (2014).
Church, C. et al. Overexpression of Fto leads to increased food intake and results in obesity. Nat. Genet. 42 , 1086–1092 (2010).
Fischer, J. et al. Inactivation of the Fto gene protects from obesity. Nature 458 , 894–898 (2009).
Cheung, M. K., Gulati, P., O’Rahilly, S. & Yeo, G. S. FTO expression is regulated by availability of essential amino acids. Int. J. Obes. 37 , 744–747 (2013).
Gulati, P. et al. Role for the obesity-related FTO gene in the cellular sensing of amino acids. Proc. Natl Acad. Sci. USA 110 , 2557–2562 (2013).
Karra, E. et al. A link between FTO, ghrelin, and impaired brain food-cue responsivity. J. Clin. Invest. 123 , 3539–3551 (2013).
Hess, M. E. et al. The fat mass and obesity associated gene (Fto) regulates activity of the dopaminergic midbrain circuitry. Nat. Neurosci. 16 , 1042–1048 (2013).
Ruud, J. et al. The fat mass and obesity-associated protein (FTO) regulates locomotor responses to novelty via D2R medium spiny neurons. Cell Rep. 27 , 3182–3198.e9 (2019).
Stratigopoulos, G., LeDuc, C. A., Cremona, M. L., Chung, W. K. & Leibel, R. L. Cut-like homeobox 1 (CUX1) regulates expression of the fat mass and obesity-associated and retinitis pigmentosa GTPase regulator-interacting protein-1-like (RPGRIP1L) genes and coordinates leptin receptor signaling. J. Biol. Chem. 286 , 2155–2170 (2011).
Stratigopoulos, G. et al. Hypomorphism for RPGRIP1L, a ciliary gene vicinal to the FTO locus, causes increased adiposity in mice. Cell Metab. 19 , 767–779 (2014).
Stratigopoulos, G. et al. Hypomorphism of Fto and Rpgrip1l causes obesity in mice. J. Clin. Invest. 126 , 1897–1910 (2016).
Smemo, S. et al. Obesity-associated variants within FTO form long-range functional connections with IRX3. Nature 507 , 371–375 (2014).
Claussnitzer, M. et al. FTO obesity variant circuitry and adipocyte browning in humans. N. Engl. J. Med. 373 , 895–907 (2015).
O’Rahilly, S., Coll, A. P. & Yeo, G. S. FTO obesity variant and adipocyte browning in humans. N. Engl. J. Med. 374 , 191 (2016).
PubMed Google Scholar
Sobreira, D. R. et al. Extensive pleiotropism and allelic heterogeneity mediate metabolic effects of IRX3 and IRX5. Science 372 , 1085–1091 (2021).
Almen, M. S. et al. The obesity gene, TMEM18, is of ancient origin, found in majority of neuronal cells in all major brain regions and associated with obesity in severely obese children. BMC Med. Genet. 11 , 58 (2010).
Larder, R. et al. Obesity-associated gene TMEM18 has a role in the central control of appetite and body weight regulation. Proc. Natl Acad. Sci. USA 114 , 9421–9426 (2017). Aside from the efforts at characterizing the FTO locus, this paper establishes a plausible role for yet another GWAS-identified candidate gene, TMEM18 , in energy homeostasis .
Wiemerslage, L. et al. The Drosophila ortholog of TMEM18 regulates insulin and glucagon-like signaling. J. Endocrinol. 229 , 233–243 (2016).
Biederer, T. et al. SynCAM, a synaptic adhesion molecule that drives synapse assembly. Science 297 , 1525–1531 (2002).
Yan, X. et al. Cadm2 regulates body weight and energy homeostasis in mice. Mol. Metab. 8 , 180–188 (2018).
Rathjen, T. et al. Regulation of body weight and energy homeostasis by neuronal cell adhesion molecule 1. Nat. Neurosci. 20 , 1096–1103 (2017).
Wheeler, E. et al. Genome-wide SNP and CNV analysis identifies common and low-frequency variants associated with severe early-onset obesity. Nat. Genet. 45 , 513–517 (2013).
Schafer, M., Brauer, A. U., Savaskan, N. E., Rathjen, F. G. & Brummendorf, T. Neurotractin/kilon promotes neurite outgrowth and is expressed on reactive astrocytes after entorhinal cortex lesion. Mol. Cell. Neurosci. 29 , 580–590 (2005).
Matikainen-Ankney, B. A. & Kravitz, A. V. Persistent effects of obesity: a neuroplasticity hypothesis. Ann. N. Y. Acad. Sci. 1428 , 221–239 (2018).
Lee, A. W. et al. Functional inactivation of the genome-wide association study obesity gene neuronal growth regulator 1 in mice causes a body mass phenotype. PLoS ONE 7 , e41537 (2012).
Joo, Y., Kim, H., Lee, S. & Lee, S. Neuronal growth regulator 1-deficient mice show increased adiposity and decreased muscle mass. Int. J. Obes. 43 , 1769–1782 (2019).
Boender, A. J., van Gestel, M. A., Garner, K. M., Luijendijk, M. C. & Adan, R. A. The obesity-associated gene Negr1 regulates aspects of energy balance in rat hypothalamic areas. Physiol. Rep. 2 , e12083 (2014).
Singh, K. et al. Neuronal growth and behavioral alterations in mice deficient for the psychiatric disease-associated negr1 gene. Front. Mol. Neurosci. 11 , 30 (2018).
Szczurkowska, J. et al. NEGR1 and FGFR2 cooperatively regulate cortical development and core behaviours related to autism disorders in mice. Brain 141 , 2772–2794 (2018).
PubMed PubMed Central Google Scholar
Noh, K. et al. Negr1 controls adult hippocampal neurogenesis and affective behaviors. Mol. Psychiatry 24 , 1189–1205 (2019).
Cano-Gamez, E. & Trynka, G. From GWAS to function: using functional genomics to identify the mechanisms underlying complex diseases. Front. Genet. 11 , 424 (2020).
Loos, R. J. et al. Common variants near MC4R are associated with fat mass, weight and risk of obesity. Nat. Genet. 40 , 768–775 (2008).
Chambers, J. C. et al. Common genetic variation near MC4R is associated with waist circumference and insulin resistance. Nat. Genet. 40 , 716–718 (2008).
Yaghootkar, H. et al. Genetic studies of leptin concentrations implicate leptin in the regulation of early adiposity. Diabetes 69 , 2806–2818 (2020).
Kichaev, G. et al. Leveraging polygenic functional enrichment to improve GWAS power. Am. J. Hum. Genet. 104 , 65–75 (2019).
Pulit, S. L. et al. Meta-analysis of genome-wide association studies for body fat distribution in 694 649 individuals of European ancestry. Hum. Mol. Genet. 28 , 166–174 (2019).
Wang, Y. et al. Melanocortin 4 receptor signals at the neuronal primary cilium to control food intake and body weight. J. Clin. Invest. 131 , e142064 (2021).
Article CAS PubMed Central Google Scholar
Farooqi, I. S. et al. Effects of recombinant leptin therapy in a child with congenital leptin deficiency. N. Engl. J. Med. 341 , 879–884 (1999). This paper is the first description of the treatment of leptin deficiency using leptin replacement therapy .
Farooqi, I. S. & O’Rahilly, S. 20 years of leptin: human disorders of leptin action. J. Endocrinol. 223 , T63–T70 (2014).
Yeo, G. S. H. et al. The melanocortin pathway and energy homeostasis: from discovery to obesity therapy. Mol. Metab. 48 , 101206 (2021).
Kuhnen, P. et al. Proopiomelanocortin deficiency treated with a melanocortin-4 receptor agonist. N. Engl. J. Med. 375 , 240–246 (2016). This paper is the first to demonstrate that the melanocortin pathway is a relevant and, so far, safe therapeutic target for the treatment of at least rare genetic causes of obesity .
Clement, K. et al. MC4R agonism promotes durable weight loss in patients with leptin receptor deficiency. Nat. Med. 24 , 551–555 (2018).
Clement, K. et al. Efficacy and safety of setmelanotide, an MC4R agonist, in individuals with severe obesity due to LEPR or POMC deficiency: single-arm, open-label, multicentre, phase 3 trials. Lancet Diabetes Endocrinol. 8 , 960–970 (2020).
Ayers, K. L. et al. Melanocortin 4 receptor pathway dysfunction in obesity: patient stratification aimed at MC4R agonist treatment. J. Clin. Endocrinol. Metab. 103 , 2601–2612 (2018).
Heymsfield, S. B. et al. Recombinant leptin for weight loss in obese and lean adults: a randomized, controlled, dose-escalation trial. JAMA 282 , 1568–1575 (1999).
Collet, T. H. et al. Evaluation of a melanocortin-4 receptor (MC4R) agonist (setmelanotide) in MC4R deficiency. Mol. Metab. 6 , 1321–1329 (2017).
Khera, A. V. et al. Polygenic prediction of weight and obesity trajectories from birth to adulthood. Cell 177 , 587–596.e9 (2019). This study describes a comprehensive PGS and its association with BMI throughout the life course .
Loos, R. J. F. 15 years of genome-wide association studies and no signs of slowing down. Nat. Commun. 11 , 5900 (2020).
Pearce, L. R. et al. KSR2 mutations are associated with obesity, insulin resistance, and impaired cellular fuel oxidation. Cell 155 , 765–777 (2013).
Greenfield, J. R. et al. Modulation of blood pressure by central melanocortinergic pathways. N. Engl. J. Med. 360 , 44–52 (2009).
Torkamani, A. & Topol, E. Polygenic risk scores expand to obesity. Cell 177 , 518–520 (2019).
Download references
Acknowledgements
R.J.F.L. is supported by funding from Novo Nordisk Foundation (NNF Laureate Award) and the US National Institutes of Health (R01DK110113; R01DK107786; R01HL142302; R01 DK124097). G.S.H.Y. is supported by the Medical Research Council (MRC Metabolic Diseases Unit (MC_UU_00014/1)). The authors thank M. Guindo Martinez for her help with creating data for Fig. 3 and Supplementary Tables 1 and 2.
Author information
Authors and affiliations.
Novo Nordisk Foundation Center for Basic Metabolic Research, University of Copenhagen, Copenhagen, Denmark
Ruth J. F. Loos
Charles Bronfman Institute for Personalized Medicine, Icahn School of Medicine at Mount Sinai, New York, NY, USA
Mindich Child Health and Development Institute, Icahn School of Medicine at Mount Sinai, New York, NY, USA
Department of Environmental Medicine and Public Health, Icahn School of Medicine at Mount Sinai, New York, NY, USA
MRC Metabolic Diseases Unit, University of Cambridge Metabolic Research Laboratories, Wellcome-MRC Institute of Metabolic Science, Addenbrooke’s Hospital, Cambridge, UK
Giles S. H. Yeo
You can also search for this author in PubMed Google Scholar
Contributions
The authors contributed equally to all aspects of the article.
Corresponding authors
Correspondence to Ruth J. F. Loos or Giles S. H. Yeo .
Ethics declarations
Competing interests.
The authors declare no competing interests.
Additional information
Peer review information.
Nature Reviews Genetics thanks D. Meyre, T. Fall and the other, anonymous, reviewer(s) for their contribution to the peer review of this work.

Publisher’s note
Springer Nature remains neutral with regard to jurisdictional claims in published maps and institutional affiliations.
Related links
23andMe: https://research.23andme.com/collaborate/
All of Us: https://allofus.nih.gov/
Biobank Japan: http://jenger.riken.jp/en/
Genetic Investigation for ANthropometric Traits (GIANT) consortium: https://portals.broadinstitute.org/collaboration/giant/index.php/Main_Page
Million Veterans Project: https://www.research.va.gov/mvp/
NCD Risk Factor Collaboration (NCD RisC): https://www.ncdrisc.org/data-visualisations.html
UK Biobank: https://www.ukbiobank.ac.uk/
Supplementary information
Supplementary information.
An environment that promotes weight gain.
A severe, early-onset form of obesity, caused by a single-gene mutation, with little or no influence of the environment.
A common multifactorial form of obesity, resulting from an interaction between the obesogenic environment and hundreds of genetic variants.
An approach used to understand the function of a gene by analysing the consequences of genetically manipulating specific sequences within the gene.
A hypothesis-driven approach to study the effect of a given gene (chosen based on the current understanding of its biology and pathophysiology) on susceptibility to the phenotype under study.
A method that relies on the relatedness of study participants to test whether certain chromosomal regions co-segregate with a disease or trait across generations.
(GWAS). A hypothesis-generating approach that screens whole genomes for associations between genetic variants and a phenotype of interest at much higher resolution than is possible for genome-wide linkage studies, and is thus better able to narrow down the associated locus.
(PGS). A measure used to assess an individual’s genetic susceptibility to disease, calculated by summing the number of disease-increasing alleles, weighted by each variant’s effect size observed in a genome-wide association study.
(AUC ROC ). A metric used to assess the ability of a predictor to discriminate between individuals with and without a disease. The AUC ranges from 0.50 (equal to tossing a coin) to 1.0 (perfect prediction).
Rights and permissions
Reprints and permissions
About this article
Cite this article.
Loos, R.J.F., Yeo, G.S.H. The genetics of obesity: from discovery to biology. Nat Rev Genet 23 , 120–133 (2022). https://doi.org/10.1038/s41576-021-00414-z
Download citation
Accepted : 17 August 2021
Published : 23 September 2021
Issue Date : February 2022
DOI : https://doi.org/10.1038/s41576-021-00414-z
Share this article
Anyone you share the following link with will be able to read this content:
Sorry, a shareable link is not currently available for this article.
Provided by the Springer Nature SharedIt content-sharing initiative
This article is cited by
Genetic effects of sequence-conserved enhancer-like elements on human complex traits.
- Wing Hung Wong
Genome Biology (2024)
A low-calorie meal replacement improves body composition and metabolic parameters in shift workers with overweight and obesity: a randomized, controlled, parallel group trial
- Piumika Sooriyaarachchi
- Ranil Jayawardena
- Neil A. King
Nutrition & Metabolism (2024)
Hyperphagia and impulsivity: use of self-administered Dykens’ and in-house impulsivity questionnaires to characterize eating behaviors in children with severe and early-onset obesity
- Lara Arnouk
- Hélène Chantereau
- Béatrice Dubern
Orphanet Journal of Rare Diseases (2024)
Associations between maternal pre-pregnancy BMI and infant striatal mean diffusivity
- Aylin Rosberg
- Harri Merisaari
- Jetro J. Tuulari
BMC Medicine (2024)
Identifying latent genetic interactions in genome-wide association studies using multiple traits
- Andrew J. Bass
- Shijia Bian
- Michael P. Epstein
Genome Medicine (2024)
Quick links
- Explore articles by subject
- Guide to authors
- Editorial policies
Sign up for the Nature Briefing: Translational Research newsletter — top stories in biotechnology, drug discovery and pharma.


An official website of the United States government
The .gov means it’s official. Federal government websites often end in .gov or .mil. Before sharing sensitive information, make sure you’re on a federal government site.
The site is secure. The https:// ensures that you are connecting to the official website and that any information you provide is encrypted and transmitted securely.
- Publications
- Account settings
- My Bibliography
- Collections
- Citation manager
Save citation to file
Email citation, add to collections.
- Create a new collection
- Add to an existing collection
Add to My Bibliography
Your saved search, create a file for external citation management software, your rss feed.
- Search in PubMed
- Search in NLM Catalog
- Add to Search
The epidemiology of obesity
Affiliations.
- 1 Clinical Nutrition Research Centre (CNRC), Singapore Institute for Clinical Sciences (SICS), Agency for Science, Technology and Research (A*STAR), National University Health System, Singapore.
- 2 Clinical Nutrition Research Centre (CNRC), Singapore Institute for Clinical Sciences (SICS), Agency for Science, Technology and Research (A*STAR), National University Health System, Singapore; Department of Physiology, Yong Loo Lin School of Medicine, National University of Singapore (NUS), Singapore; Department of Nutrition, Exercise and Sports - Obesity Research, University of Copenhagen, Denmark. Electronic address: [email protected].
- PMID: 30253139
- DOI: 10.1016/j.metabol.2018.09.005
Obesity is a complex multifactorial disease. The worldwide prevalence of overweight and obesity has doubled since 1980 to an extent that nearly a third of the world's population is now classified as overweight or obese. Obesity rates have increased in all ages and both sexes irrespective of geographical locality, ethnicity or socioeconomic status, although the prevalence of obesity is generally greater in older persons and women. This trend was similar across regions and countries, although absolute prevalence rates of overweight and obesity varied widely. For some developed countries, the prevalence rates of obesity seem to have levelled off during the past few years. Body mass index (BMI) is typically used to define overweight and obesity in epidemiological studies. However, BMI has low sensitivity and there is a large inter-individual variability in the percent body fat for any given BMI value, partly attributed to age, sex, and ethnicity. For instance, Asians have greater percent body fat than Caucasians for the same BMI. Greater cardiometabolic risk has also been associated with the localization of excess fat in the visceral adipose tissue and ectopic depots (such as muscle and liver), as well as in cases of increased fat to lean mass ratio (e.g. metabolically-obese normal-weight). These data suggest that obesity may be far more common and requires more urgent attention than what large epidemiological studies suggest. Simply relying on BMI to assess its prevalence could hinder future interventions aimed at obesity prevention and control.
Keywords: Adiposity; BMI; Health risk; Prevalence.
Copyright © 2018 Elsevier Inc. All rights reserved.
PubMed Disclaimer
Similar articles
- Body mass index classification misses subjects with increased cardiometabolic risk factors related to elevated adiposity. Gómez-Ambrosi J, Silva C, Galofré JC, Escalada J, Santos S, Millán D, Vila N, Ibañez P, Gil MJ, Valentí V, Rotellar F, Ramírez B, Salvador J, Frühbeck G. Gómez-Ambrosi J, et al. Int J Obes (Lond). 2012 Feb;36(2):286-94. doi: 10.1038/ijo.2011.100. Epub 2011 May 17. Int J Obes (Lond). 2012. PMID: 21587201
- Screening and Interventions for Childhood Overweight [Internet]. Whitlock EP, Williams SB, Gold R, Smith P, Shipman S. Whitlock EP, et al. Rockville (MD): Agency for Healthcare Research and Quality (US); 2005 Jul. Rockville (MD): Agency for Healthcare Research and Quality (US); 2005 Jul. PMID: 20722132 Free Books & Documents. Review.
- Behavioral and Pharmacotherapy Weight Loss Interventions to Prevent Obesity-Related Morbidity and Mortality in Adults: An Updated Systematic Review for the U.S. Preventive Services Task Force [Internet]. LeBlanc EL, Patnode CD, Webber EM, Redmond N, Rushkin M, O’Connor EA. LeBlanc EL, et al. Rockville (MD): Agency for Healthcare Research and Quality (US); 2018 Sep. Report No.: 18-05239-EF-1. Rockville (MD): Agency for Healthcare Research and Quality (US); 2018 Sep. Report No.: 18-05239-EF-1. PMID: 30354042 Free Books & Documents. Review.
- BMI values and other anthropometric and functional measurements as predictors of obesity in a selected group of adolescents. Turconi G, Guarcello M, Maccarini L, Bazzano R, Zaccardo A, Roggi C. Turconi G, et al. Eur J Nutr. 2006 Mar;45(3):136-43. doi: 10.1007/s00394-005-0571-x. Epub 2005 Jul 14. Eur J Nutr. 2006. PMID: 16010452
- Prevalence of underweight, overweight and obesity on the basis of body mass index and body fat percentage in Hungarian schoolchildren: representative survey in metropolitan elementary schools. Antal M, Péter S, Biró L, Nagy K, Regöly-Mérei A, Arató G, Szabó C, Martos E. Antal M, et al. Ann Nutr Metab. 2009;54(3):171-6. doi: 10.1159/000217813. Epub 2009 May 6. Ann Nutr Metab. 2009. PMID: 19420909
- The Link Between Weight-Adjusted-Waist Index and Psoriasis in Adults: A Cross-Sectional Study Based on 2009-2014 and 2003-2006 Data. Jiang Y, Jia M. Jiang Y, et al. Clin Cosmet Investig Dermatol. 2024 Aug 5;17:1763-1772. doi: 10.2147/CCID.S471528. eCollection 2024. Clin Cosmet Investig Dermatol. 2024. PMID: 39132027 Free PMC article.
- Sex-Dependent T Cell Dysregulation in Mice with Diet-Induced Obesity. Brummer C, Singer K, Brand A, Bruss C, Renner K, Herr W, Pukrop T, Dorn C, Hellerbrand C, Matos C, Kreutz M. Brummer C, et al. Int J Mol Sci. 2024 Jul 28;25(15):8234. doi: 10.3390/ijms25158234. Int J Mol Sci. 2024. PMID: 39125804 Free PMC article.
- Molecular Mechanisms behind Obesity and Their Potential Exploitation in Current and Future Therapy. Nicze M, Dec A, Borówka M, Krzyżak D, Bołdys A, Bułdak Ł, Okopień B. Nicze M, et al. Int J Mol Sci. 2024 Jul 27;25(15):8202. doi: 10.3390/ijms25158202. Int J Mol Sci. 2024. PMID: 39125772 Free PMC article. Review.
- Using Advanced Convolutional Neural Network Approaches to Reveal Patient Age, Gender, and Weight Based on Tongue Images. Li X, Li L, Wei J, Zhang P, Turchenko V, Vempala N, Kabakov E, Habib F, Gupta A, Huang H, Lee K. Li X, et al. Biomed Res Int. 2024 Aug 1;2024:5551209. doi: 10.1155/2024/5551209. eCollection 2024. Biomed Res Int. 2024. PMID: 39118805 Free PMC article.
- The potential effect of α7 nicotinic receptors modulation on palatable food-induced dependence-like behaviors. Alameen AA, AlSharari SD, Alshammari MA, Damaj MI, Sari Y. Alameen AA, et al. Saudi Pharm J. 2024 Aug;32(8):102138. doi: 10.1016/j.jsps.2024.102138. Epub 2024 Jul 4. Saudi Pharm J. 2024. PMID: 39109164 Free PMC article.
Publication types
- Search in MeSH
Related information
- Cited in Books
LinkOut - more resources
Full text sources.
- Elsevier Science
Other Literature Sources
- scite Smart Citations
- Genetic Alliance
- MedlinePlus Health Information
Miscellaneous
- NCI CPTAC Assay Portal
- Citation Manager
NCBI Literature Resources
MeSH PMC Bookshelf Disclaimer
The PubMed wordmark and PubMed logo are registered trademarks of the U.S. Department of Health and Human Services (HHS). Unauthorized use of these marks is strictly prohibited.
Is the Obesity Epidemic a Public Health Problem? A Decade of Research on the Economics of Obesity
The world-wide and ongoing rise in obesity has generated enormous popular interest and policy concern in developing countries, where it is rapidly becoming the major public health problem facing such nations. As a consequence, there has been a rapidly growing field of economic analysis of the causes and consequences of this phenomenon. This paper discusses some of the central themes of this decade long research program, aiming at synthesizing the different strands of the literature, and to point to future research that seems particularly productive.
We are thankful to Mary Peate for research assistance. The views expressed herein are those of the author(s) and do not necessarily reflect the views of the National Bureau of Economic Research.
MARC RIS BibTeΧ
Download Citation Data
Published Versions
Tomas J. Philipson & Richard A. Posner, 2008. "Is the Obesity Epidemic a Public Health Problem? A Review of Zoltan J. Acs and Alan Lyles's Obesity, Business and Public Policy," Journal of Economic Literature, American Economic Association, vol. 46(4), pages 974-82, December.
More from NBER
In addition to working papers , the NBER disseminates affiliates’ latest findings through a range of free periodicals — the NBER Reporter , the NBER Digest , the Bulletin on Retirement and Disability , the Bulletin on Health , and the Bulletin on Entrepreneurship — as well as online conference reports , video lectures , and interviews .
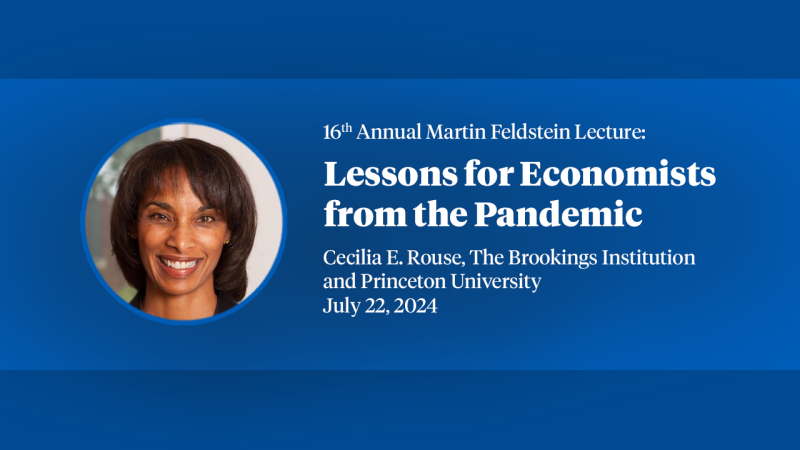
Stay informed: Sign up for eNews
- Facebook (opens in new window)
- X (opens in new window)
- Instagram (opens in new window)
- YouTube (opens in new window)
- LinkedIn (opens in new window)
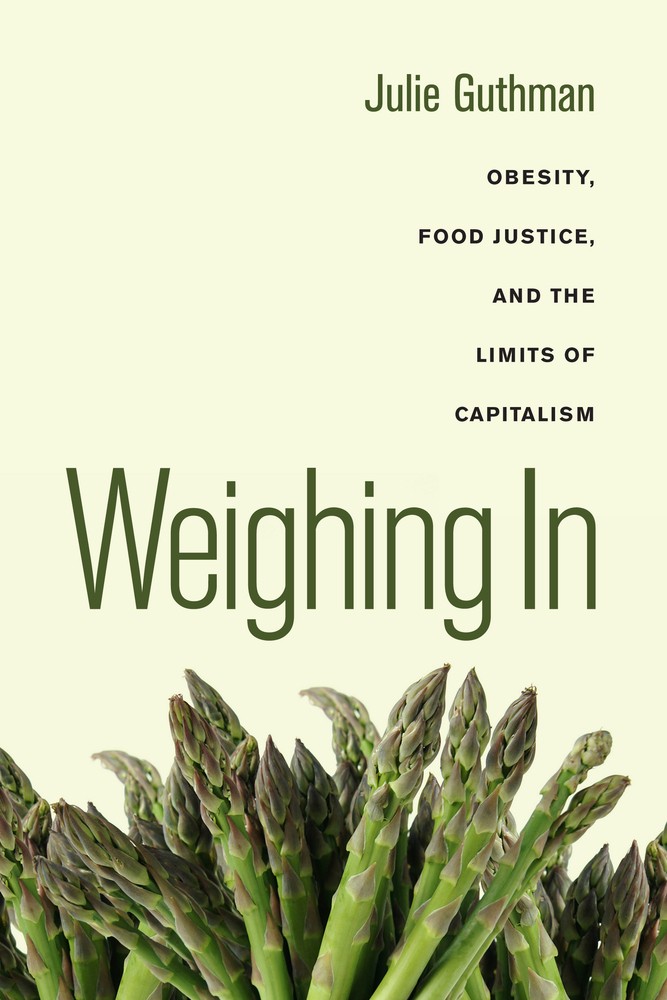
Weighing In
- California Studies in Food and Culture
- Amazon (opens in new window)
- Barnes & Noble (opens in new window)
- Bookshop (opens in new window)
- UC Press (opens in new window)
About the Book
About the author, table of contents.
" Weighing In is an outcome of impressive intellectual, emotional, and physical labor on the part of the author." — Gastronomica
“A rich topography of US obesity that accomplishes the difficult feat of challenging the discursive foundations of the ‘obesity crisis’ while critiquing conventional food capitalism. . . . Weighing In is the book that fat studies and critical geographers of fat have been waiting for.” — Social & Cultural Geography
“If you have any interest in the causes of obesity, our societal response to the ‘obesity epidemic,’ the role of capitalism in our food system, or want to be challenged with a book that goes against everything the media has told you about weight gain—this will be a rewarding read.” — Serious Eats
"Guthman’s Weighing In is a much-needed critical analysis of the dominant discourse surrounding the so-called ‘‘obesity epidemic.’’ — Agriculture and Human Values
“Guthman usefully challenges healthism in obesity research and food movements where consumption eclipses production.” — Sociology of Health & Illness
" Weighing In positions a much-needed, much-expanded food systems perspective on obesity." — American Studies Journal
- ASFS Book Award 2012 , Association for the Study of Food and Society
- James M. Blaut Innovative Publication Award 2012 , Cultural and Political Ecology Specialty Group of the Association of American Ge
- Finalist for the C. Wright Mills Award 2012 , Society for the Study of Social Problems
Interview with the author.

An official website of the United States government
The .gov means it’s official. Federal government websites often end in .gov or .mil. Before sharing sensitive information, make sure you’re on a federal government site.
The site is secure. The https:// ensures that you are connecting to the official website and that any information you provide is encrypted and transmitted securely.
- Publications
- Account settings
Preview improvements coming to the PMC website in October 2024. Learn More or Try it out now .
- Advanced Search
- Journal List
- Glob Pediatr Health
Childhood and Adolescent Obesity in the United States: A Public Health Concern
Adekunle sanyaolu.
1 Federal Ministry of Health, Abuja, Nigeria
Chuku Okorie
2 Essex County College, Newark, NJ, USA
3 Saint James School of Medicine, Anguilla, British West Indies
Jennifer Locke
Saif rehman.
Childhood and adolescent obesity have reached epidemic levels in the United States. Currently, about 17% of US children are presenting with obesity. Obesity can affect all aspects of the children including their psychological as well as cardiovascular health; also, their overall physical health is affected. The association between obesity and other conditions makes it a public health concern for children and adolescents. Due to the increase in the prevalence of obesity among children, a variety of research studies have been conducted to discover what associations and risk factors increase the probability that a child will present with obesity. While a complete picture of all the risk factors associated with obesity remains elusive, the combination of diet, exercise, physiological factors, and psychological factors is important in the control and prevention of childhood obesity; thus, all researchers agree that prevention is the key strategy for controlling the current problem. Primary prevention methods are aimed at educating the child and family, as well as encouraging appropriate diet and exercise from a young age through adulthood, while secondary prevention is targeted at lessening the effect of childhood obesity to prevent the child from continuing the unhealthy habits and obesity into adulthood. A combination of both primary and secondary prevention is necessary to achieve the best results. This review article highlights the health implications including physiological and psychological factors comorbidities, as well as the epidemiology, risk factors, prevention, and control of childhood and adolescent obesity in the United States.
Introduction
Childhood and adolescent obesity have reached epidemic levels in the United States, affecting the lives of millions of people. In the past 3 decades, the prevalence of childhood obesity has more than doubled in children and tripled in adolescents. 1 The latest data from the National Health and Nutrition Examination Survey show that the prevalence of obesity among US children and adolescents was 18.5% in 2015-2016. Overall, the prevalence of obesity among adolescents (12-19 years; 20.6%) and school-aged children (6-11 years; 18.4%) was higher than among preschool-aged children (2-5 years; 13.9%). School-aged boys (20.4%) had a higher prevalence of obesity than preschool-aged boys (14.3%). Adolescent girls (20.9%) had a higher prevalence of obesity than preschool-aged girls (13.5%; Figure 1 ). 1 Moreover, the rates of obesity have been steadily rising from 1999-2000 through 2015-2016 ( Figure 2 ). 1 According to Ahmad et al, 80% of adolescents aged 10 to 14 years, 25% of children younger than the age of 5 years, and 50% of children aged 6 to 9 years with obesity are at risk of remaining adults with obesity. 2

Prevalence of obesity among children and adolescents aged 2 to 19 years, by sex and age: the United States, 2015-2016.

Trends in obesity prevalence among children and adolescents aged 2 to 19 years: the United States, 1999-2000 through 2015-2016.
Obesity can affect all aspects of children and adolescents including but not limited to their psychological health and cardiovascular health and also their overall physical health. 3 The association between obesity and morbid outcomes makes it a public health concern for children and adolescents. 4 Obesity has an enormous impact on both physical and psychological health. Consequently, it is associated with several comorbidity conditions such as hypertension, hyperlipidemia, diabetes, sleep apnea, poor self-esteem, and even serious forms of depression. 5 In addition, children with obesity who were followed-up to adulthood were much more likely to suffer from cardiovascular and digestive diseases. 3 The increase in body fat also exposes the children to increase in the risk of numerous forms of cancers, such as breast, colon, esophageal, kidney, and pancreatic cancers. 6
Due to its public health significance, the increasing trend in childhood obesity needs to be closely monitored. 7 However, these trends have proved to be challenging to quantify and compare. While there are many factors and areas to consider when discussing obesity in children and adolescents, there are a few trends that are evident in recent studies. For example, the prevalence of obesity varies among ethnic groups, age, sex, education levels, and socioeconomic status. A report published by the National Center for Health Statistics using data from the National Health and Nutrition Examination Survey provides the most recent national estimates from 2015 to 2016 on obesity prevalence by sex, age, race, and overall estimates from 1999-2000 through 2015-2016. 1 Prevalence of obesity among non-Hispanic black (22.0%) and Hispanic (25.8%) children and adolescents aged 2 to 19 years was higher than among both non-Hispanic white (14.1%) and non-Hispanic Asian (11.0%) children and adolescents. There were no significant differences in the prevalence of obesity between non-Hispanic white and non-Hispanic Asian children and adolescents or between non-Hispanic black and Hispanic children and adolescents. The pattern among girls was similar to the pattern in all children and adolescents. The prevalence of obesity was 25.1% in non-Hispanic black, 23.6% in Hispanic, 13.5% in non-Hispanic white, and 10.1% in non-Hispanic Asian girls. The pattern among boys was similar to the pattern in all children and adolescents except that Hispanic boys (28.0%) had a higher prevalence of obesity than non-Hispanic black boys (19.0%; Figure 3 ). 1 This review article is aimed at studying the health implications including physical and psychological factors and comorbidities, as well as the epidemiology, risk factors, prevention, and control of childhood and adolescent obesity in the United States.

Prevalence of obesity among children and adolescents aged 2 to 19 years, by sex and race and Hispanic origin: the United States, 2015-2016.
Methodology
We performed a literature search using online electronic databases (PubMed, MedlinePlus, Mendeley, Google Scholar, Research Gate, Global Health, and Scopus) using the keywords “childhood,” “adolescents,” “obesity,” “BMI,” and “overweight.” Articles were retrieved and selected based on relevance to the research question.
Ethical Approval and Informed Consent
Ethics approval and informed consent were not required for this narrative review.
Definition of Childhood Obesity
Defining obesity requires a suitable measurement of body fat and an appropriate cutoff range. 8 Body mass index (BMI) was calculated as weight in kilograms divided by height in meters squared, rounded to 1 decimal place. Obesity in children and adolescents was defined as a BMI of greater than or equal to the age- and sex-specific 95th percentile and overweight with a BMI between the 85th and 95th percentiles of the 2000 Centers for Disease Control and Prevention (CDC) growth charts. 9
However, the use of the BMI percentile according to the age/sex of the CDC growth charts for very high BMIs can result in estimates that differ substantially from those that are observed, 10 , 11 and this constrains the maximum BMI that is attainable at given sex and age. 12 , 13 These limitations have resulted in the classification of severe obesity as a BMI ≥120% of the 95th percentile rather than a percentile greater than the 95th percentile. 11 , 14 A BMI of 120% of the 95th percentile corresponds to a BMI of ~35 among 16 to 18 year olds.
Physiology of Energy Regulation and Obesity
Obesity is a chronic multifactorial disease, characterized by an excessive accumulation of adipose tissue, commonly as a result of excessive food intake and/or low energy expenditure. Obesity can be triggered by genetic, psychological, lifestyle, nutritional, environmental, and hormonal factors. 15
Obesity is found in individuals that are susceptible genetically and involves the biological defense of an elevated body fat mass, the mechanism of which could be explained in part by interactions between brain reward and homeostatic circuits, inflammatory signaling, accumulation of lipid metabolites, or other mechanisms that impair hypothalamic neurons. 16
Normal energy regulation physiology is under tight neurohormonal control. The neurohormonal control is performed in the central nervous system through neuroendocrine connections, in which circulating peripheral hormones, such as leptin and insulin, provide signals to specialized neurons of the hypothalamus reflecting body fat stores and induces appropriate responses to maintain the stability of these stores. The hypothalamic region is where the center of the regulation of hunger and satiety is located. Some of them target the activity of endogenous peptides, such as ghrelin, pancreatic polypeptide, 17 peptide YY, and neuropeptide Y, 18 as well as their receptors.
The physiology of energy regulation may result in obesity in susceptible people when it goes awry from genetic and environmental modulators. There is strong evidence of the majority of obesity cases that are associated with central resistance to both leptin and insulin actions. 19 , 20 The environmental modulators equally play critical roles in obesity. Changes in the circadian clock are associated with temporal alterations in feeding behavior and increased weight gain. 21 Stress interferes with cognitive processes such as executive function and self-regulation. Second, stress can affect behavior by inducing overeating and consumption of foods that are high in calories, fat, or sugar; by decreasing physical activity; and by shortening sleep. Third, stress triggers physiological changes in the hypothalamic-pituitary-adrenal axis, reward processing in the brain, and possibly the gut microbiome. Finally, stress can stimulate the production of biochemical hormones and peptides such as leptin, ghrelin, and neuropeptide Y. 17
The lateral hypothalamus (LH) plays a fundamental role in regulating feeding and reward-related behaviors; however, the contributions of neuronal subpopulations in the LH are yet to be identified thoroughly. 22 The LH has also been associated with other aspects of body weight regulation, such as physical activity and thermogenesis. 23 The LH contains a heterogeneous assembly of neuronal cell populations, in which γ-aminobutyric acid (GABA) neurons predominate. 23 LH GABA neurons are known to mediate multiple behaviors important for body weight regulation, thus altering energy expenditure. 23
Etiology and Risk Factors
Excess body fat is a major health concern in childhood and adolescent populations. The dramatic increase in childhood obesity foreshadows the serious health consequences of their adult life. As obesity begins from childhood and spans through adult life, it becomes increasingly more difficult to treat successfully. Being able to identify the risk factors and potential causes of childhood obesity is one of the best strategies for preventing the epidemic. 24
According to the Morbidity and Mortality Weekly Report released in 2011, there is an acceptance that there is no single cause of childhood obesity and that energy imbalance is just a part of the numerous factors. 25 Many children have a discrepancy between what is taken in and what is expended. 26 For example, children with obesity consume approximately 1000 calories more than what is necessary for their body to function healthily and to be able to participate in regular physical activities. Over 10 years, there will be an excess of 57 pounds of unnecessary weight. With excessive caloric intake, as well as sedentary lifestyles, childhood obesity will continue to rise if no changes are implemented. Adding daily physical activity, better sleep patterns, as well as dietary changes can help decrease the number of excess calories and help with obesity-related problems in the future.
Also, during childhood, excess fat accumulates when the increase in caloric intake exceeds the total energy expenditure. 26 Furthermore, children living in the United States today compared with children living in the 1900s are participating in more than 6 hours per day activities on social media. This includes but is not limited to traditional television, video gaming, and blogging/Facebook activities. An additional economic rationalization for the increase in childhood obesity is technology. In other words, Americans can now eat more in less time.
In a study, Cutler et al found that an increase in consumption of food tends to be related to technology innovation in food production and transportation. Technology has thus made it increasingly possible for firms to mass prepare food and ship to consumers for ready consumption, thereby taking advantage of scale economies in food preparation. The result of this change has been a significant reduction in the time costs for food production. These lower time costs have led to increased food consumption and, ultimately, increased weights. 27 Eliminating the time cost of food preparation disproportionately increases consumption for hyperbolic discounters because time delay is a particularly important mechanism for discouraging those individuals from consuming. 27 Society today prefers immediate satisfaction with regard to food and convenience over the long-term goals of living a long, healthy life. The availability of high-caloric, less-expensive food coupled with the extensive advertisement and easy accessibility of these foods has contributed immensely to the rising trend of obesity. 28 For example, there have been reductions in the price of McDonalds and Coca-Cola (5.44% and 34.89%, respectively) between 1990 and 2007, while there was about a 17% increase in the price of fruits and vegetables between 1997 and 2003. 29
Likewise, only 16% of children walk or bike to school today as compared with 42% in the late 1960s. However, the distance, convenience, weather, scanty sidewalks, and anxiety about crimes against children could all contribute to this difference. Furthermore, with elementary, middle, and high school combined, only 13.8% of these schools provide adequate daily physical education classes for at least 4 hours a week. 30
Some other potential risk factors have been reported through research studies that involve issues that affect the child in utero and childhood. Table 1 represents potential risk factors and confounders of childhood obesity. 31
Potential Risk Factors of Childhood Obesity.
Family characteristics | Parent’s BMI during pregnancy |
Number of siblings of the child at 18 months | |
The ethnicity of the child | |
Age of the mother at delivery | |
Childhood lifestyle | Time spent watching TV |
Time in the car per day (weekdays/weekend) | |
Duration of night sleep | |
Dietary pattern | |
Infant feeding | Breast feeding/formula feeding |
Age of introduction to solid foods | |
Intrauterine and perinatal factors | Birthweight |
Sex | |
Maternal parity | |
Maternal smoking during pregnancy (28-32 weeks) | |
Season of birth (winter, summer, fall, spring) | |
Number of fetuses | |
Other | Maternal social class (SES) |
Maternal education | |
Energy intake of the child |
Abbreviations: BMI, body mass index; SES, socioeconomic status.
Catalano et al argues that maternal BMI before conception, independent of maternal glucose status or birth weight, is a strong predictor of childhood obesity. 32 Infants at the highest quarter for weight at 8 and 18 months are more likely to become children with obesity at age 7, than children in the lower quarters. Certain behaviors have been linked to childhood obesity and overweight; these are a lack of physical activity and unhealthy eating patterns (eating more food away from home, drinking more sugar-sweetened drinks, and snacking more frequently), resulting in excess energy intake. 22 , 31 In addition, when one parent presents with obesity, there is an increased potential for the child to become obese over the years. Naturally, the risk is higher for the children when both parents present with obesity. Furthermore, a study that followed children over time observed that children who got less sleep <10.5 hours at age 3 were 45% more likely to be children with obesity at the age of 7, than children who got greater than 12 hours of sleep during their first 3 years of life. 33 , 34
While all the above-mentioned factors are informative, there is still the need for further research concerning childhood and adolescent obesity and obesity in general. Risk factors for obesity in childhood are still somewhat uncertain, and evidence-based research for preventative strategies is lacking. Moreover, effective action to prevent the childhood obesity epidemic requires evidence-based on early life risk factors, and this evidence, unfortunately, is still incomplete. Furthermore, a research study has attempted to capture the complete picture of childhood obesity early life course risk factors. In the study, they identified that parental BMI and gestational weight gain among other factors should be considered in prevention programs. 35
Health Effects of Childhood Obesity
Childhood obesity is known to have a significant impact on both physical and psychological health. Sahoo et al stated that “childhood obesity can profoundly affect children’s physical health, social and emotional well-being, as well as self-esteem.” They associated poor academic performance and a lower quality of life experienced by the child with childhood obesity. They also stated that “metabolic, cardiovascular, orthopedic, neurological, hepatic, pulmonary, and menstrual disorders among others are consequences of childhood obesity.” 36 There are many health consequences of childhood obesity, and three of the more common ones are sleep apnea, diabetes, and cardiovascular diseases. 36
Psychological Consequences of Obesity
Several studies related to childhood and adolescent obesity have focused primarily on physiological consequences. Other studies have been conducted regarding the association between psychiatric disorders and obesity; these have resulted in conflict due to obesity being found to be an insignificant factor for psychopathology. However, a comparative study by Britz et al found that high rates of mood, anxiety, somatoform, and eating disorders were detected among children with obesity. The study also observed that most psychiatric disorders began after the onset of obesity. In this large population-based study, it was found that a staggering 60% of females and 35% of males reported that they have engaged in binge eating and expressed a lack of control over their diet. 37
Goldfield et al conducted a study among 1400 adolescents with obesity, overweight, and normal weight in grades 7 to 12. Their BMIs, as determined by the International Obesity Task Force, were the criteria used to define each group. Each participant completed a questionnaire on body images, eating behaviors, and moods. Adolescents with obesity reported significantly higher body dissatisfaction, social isolation, depression symptoms, anhedonia, and negative self-esteem than those of normal weight. 38 There is widespread stigmatization of people with obesity that causes harm rather than the intention to motivate people to lose weight. Stigma contributes to behaviors such as binge eating, social isolation, avoidance of health care services, decreased physical activity, and increased weight gain, which worsens obesity and creates additional barriers to healthy behavior change. 39 Weight-based bullying in youth is considered a common, serious problem in many countries. 40 In a study conducted by O’Brien et al, to test whether the association between weight stigma experiences and disordered eating behaviors, that is, emotional eating, uncontrolled eating, and loss-of-control eating, are mediated by weight bias internalization and psychological distress among 634 undergraduate university students, and results of statistical analyses showed that weight stigma was significantly associated with all measures of disordered eating, and with weight bias internalization and psychological distress. 41
Asthma and Obesity
There is mounting evidence that childhood obesity is a risk factor for the development of asthma. 42 A research study was conducted by Belamarich et al to investigate 1322 children aged 4 to 9 years with asthma. Obesity, as defined by the CDC, is the BMI, with weight and height being greater than the 95th percentile. This was the criteria used to identify the 249 children with obesity, while the BMI between the 5th and 95th percentile identified the children who were not obese. After a baseline assessment was done, the 9-month study found that the children with obesity had a higher number of days of wheezing over 2 weeks (4.0 vs 3.4) and as well had more unscheduled emergency hospital visits (39% vs 31%). 42
Obesity directly correlates with the severity of asthma, as well as poor response to corticosteroids. 43 In fact, children with obesity who also have a history of asthma are more challenging to control and linked to worse quality of life. 44 A prospective trial found that weight loss in patients with obesity and a history of asthma can significantly aid them to control the asthma attacks. 43
Chronic Inflammation and Childhood Obesity
Lumeng and Saltiel reported that obesity in children affects multiple organ systems and predisposes them to diseases. The effect of obesity on the tissue can manifest in the development of insulin-resistant type 2 diabetes, the risk of cancer, and pulmonary diseases. 45
The inflammatory response to obesity triggers pathogens, systematic increases in circulatory inflammatory cytokines, and acute-phase reactants (eg, C-reactive proteins), which inflames the tissues. This is often caused by the activation of tissue leukocytes. Chronic inflammation in children with obesity can induce meta-inflammation that is unique when compared with other inflammatory paradigms (eg, infection, autoimmune diseases). 45 Researchers have reported that children with obesity are at risk of lifelong meta-inflammation. In these children, the inflammatory markers are elevated as early as in the third year of life. 45 , 46 This has been linked to heart disease later in life. 19 The long-term consequences of such findings can cause cumulative vascular damage that correlates with the increased weight status. 47
The short-term and long-term effects of obesity on the health of children is a significant concern because of the negative psychological and health consequences. 46 The potential negative psychological outcomes are depressive symptoms, poor body image, low self-esteem, a risk for eating disorders, and behavior and learning problems. Additional negative health consequences include insulin resistance, type 2 diabetes, asthma, hypertension, high total, and low-density lipoprotein cholesterol and triglyceride levels in the blood, low high-density lipoprotein cholesterol levels in the blood, sleep apnea, early puberty, orthopedic problems, and nonalcoholic steatohepatitis 46 , 47 ( Figure 4 ). Children with obesity are more likely to become adults with obesity, thus increasing their risk for several diseases before they even reach their teen years. 48

Comorbidities and potential health consequences of childhood obesity. 47
Prevention and Control
There are two primary components to the prevention and control of childhood obesity.
The first is to educate parents on proper nutritional requirements for their children and the second is to implement the learned information. Educating parents on proper nutrition and dietary caloric intake requirements for their children is at the forefront for the prevention of obesity; however, the way the information is disseminated may affect the usefulness of the information. For example, one of the main limitations to the education of parents about childhood obesity is that typically written information is used as the conduit to health information and disease prevention. 49 The Growing Right Onto Wellness (GROW) trial used a systematic assessment of patient education material that was used for the prevention of childhood obesity in the low health literate population. 49 Results suggest that the average readability is of grade 6 level (SMOG [Simple Measure of Gobbledygook] Index 5.63 ± 0.76 and Fry graph 6.0 ± 0.85) and that adjustment of education material must be done for low health literate populations to adequately comprehend educational material and maintain motivation on the prevention of childhood obesity. 49 A similar study was conducted to further support this improvement when using color-coordinated diagrams to help parents visualize instead of trying to comprehend with numbers and words. It proved to be successful as parents were able to see where they were going wrong and make the necessary changes in their children’s diet. 49
Similarly, the National Institute of Child Health and Human Development Study of Early Child Care and Youth Development conducted a study on 744 adolescents and parents, and analyzed data to determine if parental (maternal and paternal, individually) reactions to children’s behavior was related to childhood obesity. 50 The study concluded that informing parents that their attitude toward their children’s behaviors will play a prominent role in preventing childhood obesity. 50 Parental education on nutrition, health, and the involvement of politicians, physicians, and school personnel are key for the prevention of childhood obesity. However, community and educational institutions have begun legislating and incorporating programs such as providing healthy foods at schools and also health information sessions directed toward young individuals, aimed at preventing childhood obesity in the United States and Canada. 51
Another effective prevention measure against childhood obesity is the awareness of parents on the meal and snack portion sizes. In a systematic review conducted on the effects of portion size manipulation with children and portion education/training interventions on dietary intake with parents, it was determined that the ability of adults to accurately estimate portion size improved following education/training. 52 Education of parents and children on diet requirements has its limitations in that the information must be easy to understand and be easily accessible in order to be practical. Making the available education materials easier to understand from just tables and numbers to more relatable aspects such as colors or figures, parents were able to visualize the changes they need to make whether it is with regard to portion sizes or even seeing how much childhood obesity is present in their family. Although much of the literature provided to parents is targeted to help those with lower numeracy skills, many parents benefited from the information being comparative from right/wrong and good/bad with regard to dieting. 49
The study recommended that proper educational materials, including useful and understandable literature, be used to control meal portion sizes and to help parents identify when children are at risk of obesity. Similarly, healthy eating practices should be taught by schools as a mandatory and essential method in the prevention of childhood obesity. 52
The implementation of healthy eating practices and adequate exercise regimes are essential in the prevention and control of childhood obesity. For example, information from systematic reviews, randomized controlled trials, and well-designed observational studies indicate that evidence-based prevention and control of childhood obesity can be accomplished with the collaboration of community/school, primary health care, and home-based/family-based interventions that involve both physical activity and dietary component. 53 In particular, the control of children with obesity is of significant value, as is the prevention of obesity. Two randomized control trials of 182 families were conducted from November 2005 to September 2007, and they studied the efficacy of US pediatric obesity treatment guidelines in children aged 4 to 9 years with a standardized BMI (ZBMI) greater than the 85 percentile. 54 Briefly, Trial 1 studied the impact on ZBMI by reducing snack foods and sugar-sweetened beverages and increasing fruits, vegetables, and low-fat dairy. 54 Trial 2 studied the impact on ZBMI by decreasing sugar-sweetened beverages and increasing physical activity and increasing low-fat milk consumption and reducing television watching. In Trial 1, the resulting ZBMI reduced within 6 months, and this was maintained through to the 12th month (ΔZBMI 0-12 months = −0.12 ± 0.22). 53 In Trial 2, the resulting ZBMI reduced within 6 months and continued to improve till the 12 months (ΔZBMI 0-12 months = −0.16 ± 0.31). 50
A similar cluster-randomized trial in England studied the effects of the reduction of carbonated beverages on the number of children with obesity in 29 classes (644 children). 51 Results indicate that a decrease of 0.6 glasses of carbonated drinks (250 mL) over three days per week decreased the number of children with obesity by 0.2%, while the control group increased by 7.5% (mean difference = 7.7%, 2.2% to 13.1%) at 12 months. However, diet control is only one component of the control and prevention of childhood obesity, while adequate exercise is another. 55
A systematic review and meta-analyses of the impact of diet and exercise programs (single or combined) was done on their effects on metabolic risk reduction in the pediatric population. 56 Analyses indicated that the addition of exercise to dietary intervention led to greater improvements in the levels of high-density lipoprotein cholesterol (3.86 mg/dL; 95% confidence interval [CI] = 2.70 to 4.63), fasting glucose (−2.16 mg/dL; 95% CI = −3.78 to −0.72), and fasting insulin (−2.75 µIU/mL; 95% CI = −4.50 to −1.00) over 6 months. 56 Diet and exercise are both important factors in the control and prevention of childhood obesity. It is our recommendation that parents and community (teachers and doctors) should be involved in identifying children at risk based on their BMI and participate in implementing practices such as good diet control through the reduction of sugary drinks, fatty foods, and also encouraging safe exercise programs to prevent and control childhood obesity in the society. 56
While all of the previous data express the more obvious prevention methods with regard to childhood obesity, it is imperative to note that ensuring that the whole family is involved in the intervention will yield the greatest results. 2 All current studies indicate that families must be included in childhood treatment of obesity. However, for the success of the child’s weight loss program, it is vital that the parents understand that the causes of obesity are often a mixture of four factors: genetic causes, parental habits, overeating, and poor exercise habits. Thus, instilling some responsibility on the parents and informing them that controlled food preparation, diet control, and family participation in physical activities will all assist in the treatment and control of obesity in their children. 2
Childhood obesity has increased significantly in recent decades and has quickly become a public health crisis in the United States and all over the world. Its increase in prevalence has provoked widespread research efforts to identify the factors that contributed to these changes. 57 Obesity starts with an imbalance between caloric intake and caloric expenditure. 58 Children with obesity are at greater risk of adult obesity; therefore, if we can educate and improve the health habits of families even before they start having children, this can help reduce the increasing rate of childhood obesity in the United States. Parents and caregivers with proper education on the causes and consequences of childhood obesity can help prevent childhood obesity by providing healthy meals and snacks, daily physical activity, and nutrition education to their family members. 59 Families need to take the approach of not adapting to their family being on a diet but more of a healthy lifestyle. A family’s home environment can influence children at a young age; therefore, making changes starting in the household early can educate and influence them to grow up healthy. Although prevention programs may be more expensive in the short term, the long-term benefits acquired through prevention are much more likely to save an even greater amount of health care costs. Not only will the children have a better childhood and self-esteem, but prevention programs can also decrease the incidence of cardiovascular diseases, diabetes, stroke, and possibly cancers in adulthood. 60 The overall need to decrease the obesity rate will help children and their families in the generations to come by building a healthy lifestyle and environment. In order to tackle the climbing obesity rate, overall health and lifestyle needs to be a priority as they balance one with the other. 49 While effective interventions to thwart childhood obesity still remain elusive, the sustainability of the interventions already in place will enable children and their families to adopt these important health behaviors as lifelong practices and improve their health. 58
Treatment of Obesity and the Physiology of Energy Regulation
As discussed previously, a variety of mechanisms participate in weight regulation and the development of obesity in children, including genetics, developmental influences (“metabolic programming” or epigenetics), individual and family health behaviors, and environmental factors. Among these potential mechanisms, only environmental factors are potentially modifiable during childhood and adolescence.
Unfortunately, despite intensive lifestyle modifications and support for healthy practices within the children’s environment, some children will continue to struggle with extreme excess weight and associated comorbidities. 61 , 62 Therefore, a combination of pharmacotherapy and lifestyle modification can be considered. 61 Overweight children should not be treated with medications unless significant, severe comorbidities persist despite lifestyle modification. The use of pharmacotherapy should also be considered in overweight children with a strong family history of type 2 diabetes or cardiovascular risk factors. Constant bidirectional communication between the brain and the gastrointestinal tract, as well as the brain and other relevant tissues (ie, adipose tissue, pancreas, and liver), ensures that the brain constantly perceives and responds accordingly to the energy status/needs of the body. This elegant biological system is subject to disruption by a toxic obesogenic environment, leading to syndromes such as leptin and insulin resistance, and ultimately further exposing individuals who are obese to further weight gain and type 2 diabetes mellitus. Currently, the only Food and Drug Administration–approved prescription drug indicated for the treatment of pediatric obesity is orlistat (Xenical; Genentech USA, Inc, South San Francisco, CA). 63 Orlistat works by inhibiting gastric and pancreatic lipases, the enzymes that break down triglycerides in the intestine. Moreover, imaging studies in humans are beginning to examine the influence that higher- order/hedonic brain regions have on homeostatic areas, as well as their responsiveness to homeostatic peripheral signals. With a greater understanding of these mechanisms, the field moves closer to understanding and eventually treating the casualties of obesity.
The number of children with obesity in the United States has increased substantially over the years; due to its public health significance, the increasing trends need to be closely monitored. While a complete picture of all the risk factors associated with obesity remains elusive, many of the studies agreed that prevention is the key strategy for controlling the current problem. Since the combination of diet, exercise, and physiological and psychological factors are all important factors in the control and prevention of childhood obesity, primary prevention methods should be aimed at educating the child and family and encouraging appropriate diet and exercise from a young age through adulthood while secondary prevention should be targeted at lessening the effect of childhood obesity by preventing the child from continuing unhealthy habits and obesity into adulthood. A combination of primary and secondary prevention is necessary to achieve the best results. Thus, a combined implementation of both types of preventions can significantly help lower the current prevalence of childhood and adolescent obesity in the United States. Failure to take appropriate actions could lead to serious public health consequences.
Author Contributions: AS: Contributed to conception and design; drafted manuscript; gave final approval; agrees to be accountable for all aspects of work ensuring integrity and accuracy.
XQ: Contributed to the acquisition, analysis, and interpretation.
JL: Contributed to the acquisition, analysis, and interpretation.
SR: Contributed to the acquisition, analysis, and interpretation.
Declaration of Conflicting Interests: The author(s) declared no potential conflicts of interest with respect to the research, authorship, and/or publication of this article.
Funding: The author(s) received no financial support for the research, authorship, and/or publication of this article.


IMAGES
COMMENTS
Obesity has become a global epidemic and is one of today's most public health problems worldwide. Obesity poses a major risk for a variety of serious diseases including diabetes mellitus, non-alcoholic liver disease (NAFLD), cardiovascular disease, hypertension and stroke, and certain forms of cancer (Bluher, 2019).Obesity is mainly caused by imbalanced energy intake and expenditure due to a ...
The obesity epidemic emerged in Westernized countries during the 1980s [ 3, 4 ]. Our best evidence is that this major event started a few years earlier in the USA, namely in 1976-1980 [ 5, 6 ]. Obesity is defined as a BMI ≥ 30. There was only a small rise (approximately 0.5%) in the prevalence of obesity among American adults in the years ...
1. Introduction. Obesity is a chronic disease that is increasing in prevalence and is now considered to be a global epidemic. Epidemiologic studies have revealed an association between high body mass index (BMI) and an extensive range of chronic diseases such as Non Alcoholic Fatty Liver (NAFL), cardiovascular disease , , diabetes mellitus , several malignancies , , musculoskeletal diseases ...
In the United States, overweight and obesity are chronic diseases that contribute to excess morbidity and mortality. Despite public health efforts, these disorders are on the rise, and their consequences are burgeoning. 1 The Centers for Disease Control and Prevention report that during 2017 to 2018, the prevalence of obesity in the United States was 42.4%, which was increased from the ...
Obesity prevalence among children is >30% in the Cook Islands, Nauru and Palau, with a notable increase over the past few decades. Worldwide prevalence of obesity increased at an alarming rate in ...
The findings from our approach suggest with high predictive accuracy that by 2030 nearly 1 in 2 adults will have obesity (48.9%; 95% confidence interval [CI], 47.7 to 50.1), and the prevalence ...
Almost 20 years ago, the World Health Organization (WHO) declared the problem of rising levels of obesity a 'global epidemic', 1 yet the prevalence of overweight (body mass index (BMI; a ratio of weight to height commonly used to categorise weight status) ⩾ 25 kg/m 2) and obesity (BMI ⩾ 30 kg/m 2) has continued to rise. 2,3 In 2016, more than 1.9 billion adults (39% of the world's ...
The present study conducted a systematic literature review to examine obesity research and machine learning techniques for the prevention and treatment of obesity from 2010 to 2020. Accordingly, 93 papers are identified from the review articles as primary studies from an initial pool of over 700 papers addressing obesity.
Obesity (body mass index ≥ 30 kg/m 2) is a growing public health crisis across the world. The available data suggest that the global burden of obesity has more than tripled since 1975 ( 1 ). It is particularly high in some first world countries. However, recent data show that obesity is spreading very fast in low-and middle-income countries ...
This paper provides a comprehensive overview of the obesity epidemic, examining its causes, consequences, and potential solutions. It explores the complex interplay of genetic, environmental, and ...
Background. There has been a significant global increase in obesity rate during the last 50 years. Obesity is defined as when a person has a body mass index [BMI (kg/m 2), dividing a person's weight by the square of their height] greater than or equal to 30, overweight is defined as a BMI of 25.0-29.9.Being overweight or obesity is linked with more deaths than being underweight and is a more ...
This article is part of the PLOS Biology 20th anniversary collection. Obesity is a multifaceted disorder, affecting individuals across their life span, with increased prevalence in persons from underrepresented groups. The complexity of obesity is underscored by the multiple hypotheses proposed to pinpoint its seminal mechanisms, such as the ...
2. Classification of Body Weight in Adults. The current most widely used criteria for classifying obesity is the body mass index (BMI; body weight in kilograms, divided by height in meters squared, Table 1), which ranges from underweight or wasting (<18.5 kg/m 2) to severe or morbid obesity (≥40 kg/m 2).In both clinical and research settings, waist circumference, a measure of abdominal ...
The prevalence of obesity has tripled over the past four decades, imposing an enormous burden on people's health. Polygenic (or common) obesity and rare, severe, early-onset monogenic obesity ...
The combined burden of underweight and obesity has increased in most countries, driven by an increase in obesity, while underweight and thinness remain prevalent in south Asia and parts of Africa. A healthy nutrition transition that enhances access to nutritious foods is needed to address the remaining burden of underweight while curbing and reversing the increase in obesity.
The emerging obesity epidemic and accompanying health consequences led The Obesity Society (TOS) in 2008 to publish a position paper defining obesity as a disease. Since then, new information has emerged on the underlying mechanisms leading to excess adiposity and the associated structural, cardiometabolic, and functional disturbances.
Overweight / epidemiology. Prevalence. Obesity is a complex multifactorial disease. The worldwide prevalence of overweight and obesity has doubled since 1980 to an extent that nearly a third of the world's population is now classified as overweight or obese. Obesity rates have increased in all ages and both sexes irrespective of geograph ….
The epidemic of obesity has grown largely unchecked over the past several decades, arguably making obesity America's most pressing public health issue. Approximately 40% of American adults currently have obesity (defined as a body mass index ≥30 kg/m 2; Hales et al., 2018).Another third of the population of the United States is overweight, leaving these individuals at risk for the ...
The obesity epidemic. The World Health Organization (WHO) defines overweight and obesity as abnormal or excessive fat accumulation that presents a risk to health (WHO, 2016a).A body mass index (BMI) ≥25 kg/m 2 is generally considered overweight, while obesity is considered to be a BMI ≥ 30 kg/m 2.It is well known that obesity and overweight are a growing problem globally with high rates in ...
DOI: 10.1056/NEJMe1514957. The prevalence of severe obesity in the United States has increased dramatically, not only among adults but also among children. The increase in childhood severe obesity ...
As a consequence, there has been a rapidly growing field of economic analysis of the causes and consequences of this phenomenon. This paper discusses some of the central themes of this decade long research program, aiming at synthesizing the different strands of the literature, and to point to future research that seems particularly productive.
Both research groups described how obesity prevalence has increased in the last few decades. ... This study is one of this group's most recent papers to document US obesity prevalence during the period between 2005 - 2014. ... Baker JL, Sørensen TIA: The levelling off of the obesity epidemic since the year 1999--a review of evidence and ...
Weighing In takes on the "obesity epidemic," challenging many widely held assumptions about its causes and consequences. Julie Guthman examines fatness and its relationship to health outcomes to ask if our efforts to prevent "obesity" are sensible, efficacious, or ethical. ... Guthman gives us the research behind the questions we should ...
Introduction. Childhood and adolescent obesity have reached epidemic levels in the United States, affecting the lives of millions of people. In the past 3 decades, the prevalence of childhood obesity has more than doubled in children and tripled in adolescents. 1 The latest data from the National Health and Nutrition Examination Survey show that the prevalence of obesity among US children and ...