International Journal of Phytomedicine and Phytotherapy
- Original contribution
- Open access
- Published: 09 July 2020

Quantitative phytochemical analysis and antimicrobial activities of fresh and dry ethanol extracts of Citrus sinensis (L.) Osbeck (sweet Orange) peels
- Ehigbai I. Oikeh ORCID: orcid.org/0000-0002-4931-9085 1 , 2 ,
- Faith E. Oviasogie 3 &
- Ehimwenma S. Omoregie 1
Clinical Phytoscience volume 6 , Article number: 46 ( 2020 ) Cite this article
15k Accesses
36 Citations
Metrics details
Citrus sinensis is one of the most abundant citrus species consumed. Orange peels are a waste by-product of the fruit and may potentially contain useful phytonutrients with biological relevance.
Fresh and dry peels of sweet orange were subjected to Soxhlet extraction and then concentrated using a rotary evaporator. Total phenolic, flavonoid and tannin content were determined using standard methods. Antimicrobial activities against five (5) bacterial strains ( Staphylococcus aureus, Enterococcus faecalis, Pseudomonas aeruginosa, Escherichia coli and Salmonella typhimurium ) and three (3) fungal strains ( Candida albicans, Aspergillus niger and Penicillium notatum ) was carried out by observing the zone of inhibition using disc diffusion method.
The total phenolic, flavonoid and tannin content was higher in the fresh peel extract compared to the dry peel extract. Antimicrobial activities revealed that the fresh peel extract had better antibacterial activities against all bacterial strains and one fungal strain studied compared to the dry peel extract. Growth of Aspergillus niger and Penicillium notatum were however better inhibited by the dry peel extract than the fresh peel extract.
This study investigated the phenolic content and antimicrobial activities of fresh and dry Citrus sinensis peel extracts. The results from the study conclude that the fresh Citrus sinensis peel extract contains more phenolics and possesses better antimicrobial activities against the studied microbial strains compared to the dry peel extract. The findings in this study suggest that drying plant parts before extraction for phytonutrients may lead to loss of active components.
Citrus sinensis (L.) Osbeck (sweet orange) is the world’s most widely grown and commercialized citrus specie. The fruit of C. sinensis is mostly recognized for its vitamin C content and is also an important source of other phytochemicals such as phenolics and carotenoids which are reputed to have health benefits [ 1 , 2 , 3 ]. The sweet orange fruit is usually eaten whole or processed into juice after the peeling of the external rind (flavedo). This peeling process leads to the generation of substantial wastes [ 4 , 5 , 6 , 7 ].
Traditional medicine is an established practice in many parts of the world. The use of herbs is well documented and is the oldest approach for healing known [ 8 , 9 ]. In Nigeria, a huge percentage of the populace depend on herbal medicine for treating different ailments [ 10 ]. Studies into the discovery of plant parts with medicinal properties often make use of dried plant parts that are subjected to solvent extraction of bioactive components. This practice often doesn’t take into recognition the presence of volatile compounds that may be lost during the drying process.
Microbial drug resistance is a problem of current world interest [ 11 , 12 ]. The emergence and spread of multidrug-resistance (MDR) microbial strains presents a severe challenge to global public health. Research is increasingly turning towards the use of herbal products as new leads towards the development of better drugs against microbial strains [ 13 ].
We have reported in earlier results the presence of phytochemicals as well as evaluated the antioxidant activities of the peels (flavedo), seeds and albedo of dry C. sinensis fruits [ 1 , 7 ]. Those previous studies evaluated extracts obtained from shade-dried materials. This study therefore compares the phenolic content of fresh and dry C. sinensis peel extracts and evaluates the antibacterial and antifungal activities of these extracts.
Materials and methods
Raw materials.
Oranges were purchased from New Benin Market in Benin City, Nigeria. Oranges were obtained same day they were plucked from local farm trees not under any pesticide treatment. The fruits were washed with distilled water and the peels removed with the aid of a sharp knife. Outer peel removal was carried out to ensure that the flavedo was not harvested alongside the albedo. The flavedo were divided into two equal groups. One group was air-dried in a shade at 30–33 °C for seven days and then pulverized. The other group was pulverized immediately after peeling the oranges and then subjected to extraction. Pulverization was carried out using a sterile mortar and pestle till fine granular or powdery consistency was obtained.
Preparation of plant extract
The pulverized samples were subjected to Soxhlet extraction for a period of 12 h with 500 mL of ethanol and then concentrated using a rotary evaporator at reduced pressure. The extracts were stored in the refrigerator till required for use.
Determination of Total phenolic content
Total phenolic content was determined according to Folin-Ciocalteau reagent method of Cicco [ 14 ]. Concentrations of 0.2, 0.4, 0.6, 0.8, and 1 mg/mL of gallic acid were prepared in methanol. Extracts were also prepared in methanol to obtain concentrations of 1 mg/mL. Then 4.5 mL of distilled water was added to 0.5 mL of the extract and mixed with 0.5 mL of a ten-fold diluted Folin- Ciocalteau reagent. Subsequently, 5 mL of 7% sodium carbonate and 2 mL of distilled water were added. The mixture was allowed to stand for 90 min at room temperature before the absorbance was read at 760 nm. All determinations were performed in triplicates with gallic acid utilized as the positive control. The total phenolic content was expressed as gallic acid equivalent (GAE).
Determination of Total flavonoid content
Total flavonoid contents were estimated using the method described by Ebrahimzadeh et al , [ 15 ]. Extracts (0.5 mL of 1 mg/mL) were mixed with 1.5 mL of methanol. To this mixture, 0.1 mL of 10% aluminium chloride was added, followed by 0.1 mL of 1 M potassium acetate and 2.8 mL of distilled water. The mixture was incubated at room temperature for 30 min. The absorbance was measured by a spectrophotometer at 420 nm. The results were expressed as milligrams quercetin equivalents (QE) per gram of extract (mg QE/g extract).
Determination of Total tannin content
The total tannin content was determined by modified method of Polsheltiwar et al. , [ 16 ]. To 0.1 mL of 1 mg/mL sample extracts was added 0.5 mL of Folin-Denis reagent followed by 1 mL of Na 2 CO 3 (0.5% W / V ) solution and distilled water up to 5 mL. The absorbance was measured at 755 nm within 30 min of reaction against blank. The total tannin in the extract was expressed as the equivalent to tannin acid.
Antimicrobial assay
Test microorganisms.
Eight (8) microorganisms were used in this study - Five bacterial strains and three fungal strains. Two were gram positive ( Staphylococcus aureus and Enterococcus faecalis ) while the other three were gram negative ( Pseudomonas aeruginosa, Escherichia coli and Salmonella typhimurium ). The three fungal strains used are Candida albicans, Aspergillus niger and Penicillium notatum. All microorganisms were clinical isolates obtained from Lahor Research and Diagnostic Laboratories, Benin City, Nigeria. The identities of the test organisms were confirmed to the specie levels using standard biochemical and morphological procedures.
Antimicrobial susceptibility assay
Test organisms and 2 control strains ( S. aureus ATCC 25923 and E. coli ATCC 25922) were sub-cultured onto fresh suitable broth medium. Broth cultures were then incubated at 37 °C till the turbidity of 0.5 McFarland’s standard (1.5 × 10 8 CFU/mL). Mueller-Hinton agar was used as bacterial medium and Sabouraud agar as fungal medium. All were incubated appropriately as specified for each test organism. The turbidity of the actively growing broth culture was adjusted with sterile saline to obtain 0.5 McFarland’s standard turbidity. One milliliter of the suspension was then used to flood the surface of solid Mueller-Hinton agar plates and drained dry. Wells of 5 mm in diameter and about 2 cm apart were punched in the culture media with sterile cork borer. The extracts (0.2 mL) were thereafter used to fill the boreholes. Each plate was kept in the refrigerator at 4 °C for 1 h before incubating at 37 °C for 24 h (bacteria) and 72 h (fungi). Zones of inhibition around the wells, measured in millimeters, were used as positive bioactivity. All experiments were carried out in triplicates.
Minimum inhibitory concentration (MIC)
The organisms that showed susceptibility to the different solvent extracts were introduced into the broths containing different concentrations of each extract (Serial dilutions of the extracts corresponding to 200 μg/mL, 100 μg/mL, 50 μg/mL, 25 μg/mL and 12.5 μg/mL). The tubes were thereafter incubated for 24 h at 37 °C. The MIC was taken as the lowest concentration of the extracts that did not permit any visible growth.
Minimum bactericidal concentration (MBC) and minimum fungicidal concentration (MFC)
The tubes that showed no turbidity in the MIC test were taken and a loop-full from each tube was streaked on Mueller Hinton agar. The plates were incubated for 24 h at 37 °C and the absence of growth was observed. The concentration of the extracts that showed no growth was recorded as the MBC / MFC.
Statistical analysis
The data were expressed as mean ± SEM of three replicates. The data were subjected to one-way analysis of variance (ANOVA), and differences between means were determined by Duncan’s multiple range test using the Statistical Analysis System (SPSS Statistics 17.0) where applicable. P values ≤0.05 were regarded as significant.
The fresh peel extract (FPE) was observed to contain significantly higher (p < 0.05) phenolics than the dry peel extract (DPE). Total phenol was 27.14 ± 0.23 mg GAE/g of extract and 3.64 ± 0.09 mg GAE/g of extract for FPE and DPE, respectively (Fig. 1a ). FPE was estimated to contain a flavonoid content of 86.82 ± 1.82 mg QE/g of extract while DPE was estimated to contain 59.94 ± 0.06 mg QE/g of extract (Fig. 1b ). Figure 1c shows the estimation of tannic content. Total tannins estimated were 28.50 ± 6.80 mg TE/g of extract for FPE compared to 8.00 ± 0.33 mg TE/g of extract in the DPE.
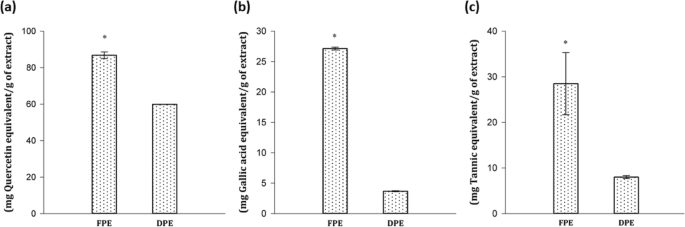
(a) Total phenolic, (b) flavonoid and (c) tannin content of fresh and dry Citrus sinensis peel extracts. Values are mean ± SEM (n = 3). * Significantly different from the other group at p < 0.05. FPE = Fresh peel extract, DPE = Dry peel extract
The results show that both the FPE and DPE of C. sinensis possess varying degrees of antimicrobial activities against the test bacterial and fungal strains (Tables 1 and 2 ). The FPE produced the widest zone of inhibition (ZOI) of 20 mm against E. faecalis. This was followed by S. aureus and P. aeruginosa with 14 mm ZOI and E. coli with 13 mm ZOI (Table 1 ). The FPE produced a 6 mm ZOI for S. typhimurium., the lowest observed for the bacterial strains studied. The DPE produced generally smaller zones of inhibition against the bacterial strains with a 12 mm zone of inhibition observed for E. faecalis and 10 mm for S. typhimurium. The DPE produced 4 mm, 6 mm and 8 mm zones of inhibition, respectively against S. aureus, P. aeruginosa and E. coli .
Table 2 shows that the FPE was most effective against C. albicans , producing an 18 mm ZOI while the DPE was most effective against P. notatum. with an observed ZOI of 10 mm. Two (2) mm zones of inhibition were observed for the FPE against A. niger and P. notatum. while 2 and 4 mm respectively for C. albicans and A. niger when exposed to the DPE.
Table 3 shows that the minimum inhibitory concentrations (MIC) ranged from 12.5 to 100 μg/mL. The lowest MIC value (12.5 μg/mL) was observed for the FPE against S. aureus, E. faecalis and P. aeruginosa. MIC values were higher for the DPE against the same microbial strains. DPE had MIC value of 50 μg/mL against P. aeruginosa and S. typhimurium. MBC values were generally higher than the MIC values obtained ranging from 25 μg/mL for the FPE against S. aureus, E. faecalis and P. aeruginosa to 200 μg/mL for DPE against S. aureus, E. faecalis and E. coli.
MIC and MFC against three fungal strains studied are shown in Table 4 . The DPE had lower MIC and MFC values against A. niger (50 μg/mL and 100 μg/mL respectively) compared to the FPE (100 μg/mL and 200 μg/mL for MIC and MFC respectively) Similar MIC and MFC (100 μg/mL and 200 μg/mL respectively) were observed for both FPE and DPE against P. notatum.
Values are mean of 3 biological replicates to the nearest mm.
MIC = Minimum inhibitory concentration; MBC = Minimum bactericidal concentration.
MIC = Minimum inhibitory concentration; MFC = Minimum fungicidal concentration.
The increasing existence of microbial resistance to drugs has made the search for new antimicrobial drugs an important and ongoing one. A current approach is to screen medicinal plants for novel antimicrobial principles. Bioactive natural products from plants have proven to be very useful in the drug design and discovery process [ 17 ].
Secondary metabolites in citrus plants have been identified as therapeutic agents in the management of several diseases. Phytochemical analysis of Citrus sinensis has revealed the presence of carbohydrates, flavonoids, glycosides, coumarin glycosides, volatile oils, organic acids, fats and fixed oils [ 7 , 18 ]. Tannins, flavonoids, saponins, phenolic compounds and essential oils are believed to be the phytochemicals responsible for the antimicrobial effects of plants [ 13 ]. Flavonoids have been linked to several biological activities including antibacterial, antioxidant and inflammatory activities. They are also known to possess the capacity to modulate enzymatic activities and inhibit cell proliferation. In plants, they are known to play a defensive role against invading pathogens [ 17 , 19 , 20 ]. Tannins form complexes with proline-rich proteins that inhibit cell protein synthesis. Synergistic action of tannins, flavonoids, alkaloids and saponins are known to inhibit the growth of pathogens [ 21 ].
The results of this study revealed that the fresh C. sinensis peel extract had significantly higher total phenol, total flavonoid and total tannin content than the dry peel extract. This may be due to loss of volatile compounds in the fresh peels during the drying process. This finding challenges the widespread practice of drying natural plant parts before solvent extraction of bioactive components from them. The drying process may lead to loss of potent compounds that may contribute to therapeutic/pharmacological activity of the plant material.
Our results agree with the findings of El-Desoukey et al [ 22 ] and Baba et al [ 23 ] who also investigated antimicrobial activities of C. sinensis peel extracts. Those papers however examined fewer organisms. The present study suggests that the fresh C. sinensis peel extract may have potent activity against microorganisms as a result of the high levels of phenolics, flavonoids and tannins present. Synergistic action of these groups of phytochemicals may be responsible for the antimicrobial effects observed in this study. On the other hand, the dry C. sinensis peel extract was observed to contain lower total phenolic, flavonoids and tannin content. This may explain why this extract is not as effective as an antimicrobial agent compared to the fresh peel extract. The antimicrobial effects observed in the dry peel extract may therefore be ascribed majorly to its flavonoid content.
Aromatic phenolic compounds in plants are well known to possess wide spectra of antimicrobial activity. These compounds are synthesized in plants dealing with a microbial infection. It has been suggested that their activity may be due to their ability to form complexes with extracellular and soluble proteins as well as bacterial cell walls [ 18 ]. This may therefore explain further, the better antibacterial effect of the fresh peel extract when compared to the dry peel extract.
Candida spp. are among the most frequently isolated microorganisms in clinical microbiology laboratories. Their relevance hinges on their ability to cause opportunistic and hospital-acquired infections [ 24 ]. In this study, the antifungal analysis shows that the fungal strains were not as susceptible to the C. sinensis peel extracts compared to the bacterial strains. The fresh peel extract was however effective in inhibiting the growth of C. albicans (ZOI 18 mm). The fresh peel extract may therefore hold promise as an antifungal agent for the management of candidiasis.
A surprising result was the greater zones of inhibition against the growth of A. niger and P. notatum by DPE compared to the FPE. It was expected that the FPE would have better antifungal activity compared to the DPE because of the greater phytochemicals in the FPE. The reason for this observation is not well understood. Further investigation utilizing a more diverse range of fungal organisms may however provide more insight as to whether DPE has better antifungal activities compared to FPE.
This preliminary study forms the basis for further research into the identification of the antibacterial compounds present in the peels of C. sinensis . This further emphasizes the waste-to-wealth potential of sweet orange wastes. The results in this study show that fresh C. sinensis peel extract contains more phenolics and possesses better antimicrobial activities against the microorganisms studied compared to the dry peel extract. Our findings also suggest that drying of plant materials prior to extraction may not always be better as certain active pharmacological compounds may be lost during this process.
Availability of data and materials
All data pertaining to this study have been included in this article.
Oikeh EI, Oriakhi K, Omoregie ES. Phenolic content and in vitro antioxidant activities of sweet Orange ( Citrus sinensis L.) fruit wastes. Archives of Basic and Applied Medicine. 2014;2(2):119–26.
Google Scholar
Liu Y, Heying E, Tanumihardjo SA. History, global distribution, and nutritional importance of Citrus fruits. Compr Rev Food Sci Food Saf. 2012;11(6):530–45.
Article CAS Google Scholar
Martínez-Cuenca MR, Primo-Capella A, Forner-Giner MA. Influence of rootstock on Citrus tree growth: effects on photosynthesis and carbohydrate distribution, plant size, yield, fruit quality, and dwarfing genotypes. 2016. InTech. .
Mandalari G, Bennett RN, Bisignano G, Saija A, Dugo G, et al. Characterization of flavonoids and pectin from bergamot ( Citrus bergamia Risso) peel, a major byproduct of essential oil extraction. Journal of Agriculture and Food Chemistry. 2006;54:197–203.
Hegazy AE, Ibrahium MI. Antioxidant activities of Orange Peel extracts. World Appl Sci J. 2012;18(5):684–8.
CAS Google Scholar
Putnik P, Bursać Kovačević D, Režek Jambrak A, Barba FJ, Cravotto G, et al . Innovative "Green" and Novel Strategies for the Extraction of Bioactive Added Value Compounds from Citrus Wastes-A Review. Molecules (Basel, Switzerland) , 2017;22(5), 680.
Oikeh EI, Oriakhi K, Omoregie ES. Proximate analysis and phytochemical screening of Citrus sinensis fruit wastes. The Bioscientist. 2013;1(2):164–70.
Ekor M. The growing use of herbal medicines: issues relating to adverse reactions and challenges in monitoring safety. Front Pharmacol. 2014;4:177.
Article Google Scholar
Yuan H, Ma Q, Ye L, Piao G. The traditional medicine and modern medicine from natural products. Molecules. 2016;21(5):559.
Onyeagba RA, Ugbogu OC, Okeke CU, Iroakasi O. Studies on the antimicrobial effects of garlic ( Allium sativum Linn), ginger ( Zingiber officinale roscoe) and lime ( Citrus aurantifolia Linn). Afr J Biotechnol. 2004;3(10):552–4.
Ayukekbong JA, Ntemgwa M, Atabe AN. The threat of antimicrobial resistance in developing countries: causes and control strategies. Antimicrob Resist Infect Control. 2017;6:47.
Aslam B, Wang W, Arshad MI, Khurshid M, Muzammil S, Rasool MH, Nisar MA, Alvi RF, Aslam MA, Qamar MU, Salamat MKF, Baloch Z. Antibiotic resistance: a rundown of a global crisis. Infect Drug Resist. 2018;10(11):1645–58.
Rahman S, Parvez AK, Islam R, Khan MH. Antibacterial activity of natural spices on multiple drug resistant Escherichia coli isolated from drinking water. Bangladesh Annals of Clinical Microbiology and Antimicrobials. 2011;10:10.
Cicco N, Lanorte MT, Paraggio M, Viggiano M, Lattanzio V. A reproducible, rapid and inexpensive Folin-Ciocalteu micro method in determining phenolics of plant methanol extracts. Microchem J. 2009;91:107–10.
Ebrahimzadeh MA, Pourmorad F, Bekhradnia AR. Iron chelating activity, phenol and flavonoid content of some medicinal plants from Iran. Afr J Biotechnol. 2008;7(18):3188–92.
Polshettiwar SA, Ganjiwale RO, Wadher SJ, Yeole PG. Spectrophotometric estimation of total tannins in some Ayurvedic eye drops. Indian J Pharm Sci. 2007;69:574–6.
Hafidh RR, Abdulamir AS, Vern LS, Bakar FA, Abas F, Jahanshiri F, Sekawi Z. Inhibition of growth of highly resistant bacterial and fungal pathogens by a natural product. The Open Microbiology Journal. 2011;5:96–106.
Dhiman A, Nanda A, Ahmad S, Narasimhan B. In vitro antimicrobial status of methanolic extract of Citrus sinensis Linn. Fruit peel. Chronicles of Young Scientists. 2012;3:204–8.
Dhanavade MJ, Jalkute CB, Ghosh JS, Sonawane KD. Study antimicrobial activity of lemon ( Citrus lemon L.) Peel extract. Br J Pharmacol Toxicol. 2011;2(3):119–22.
Gorniak I, Bartoszewski R, Kroliczewski J. Comprehensive review of antimicrobial activities of plant flavonoids. Phytochem Rev. 2019;18:241–72.
Nwankwo IU, Onwuakor CE, Aninweze ON. Antibacterial activity of Ethanolic extracts of Citrus sinensis peels on Staphylococcus aureus , Escherichia coli and Pseudomonas aeruginosa isolated from wound infections. International Journal of Advances in Pharmacy, Biology and Chemistry. 2014;3(4):941–7.
El-Desoukey RMA, Saleh ASB, Alhowamil HF. The phytochemical and antimicrobial effect of Citrus sinensis (Orange) Peel powder extracts on some animal pathogens as eco-friendly. EC Microbiology. 2018;14(6):312–8.
Baba J, Mohammed SB, Ya'aba Y, Umaru FI. Antibacterial activity of sweet Orange Citrus sinensis on some clinical Bacteria species isolated from wounds. Journal of Family Medicine and Community Health. 2018;5(4):1154.
Aboellil AH, Al-Tuwaijri MMY. Effect of some alternative medicine and biological factors on Candida albicans in Saudi Arabia. Journal of Yeast and Fungal Research. 2010;1(6):100–7.
Download references
Acknowledgements
The authors acknowledge and appreciate the support and resources made available at Lahor Research Laboratory and Medical Centre, Benin-City.
Authors declare that they did not receive any fund for this work.
Author information
Authors and affiliations.
Department of Biochemistry, Faculty of Life Sciences, University of Benin, PMB 1154, Benin City, Nigeria
Ehigbai I. Oikeh & Ehimwenma S. Omoregie
Department of Chemistry, College of Arts and Sciences, University of Kentucky, Lexington, USA
Ehigbai I. Oikeh
Department of Microbiology, Faculty of Life Sciences, University of Benin, PMB 1154, Benin City, Nigeria
Faith E. Oviasogie
You can also search for this author in PubMed Google Scholar
Contributions
All authors contributed equally. All authors read and approved the final manuscript.
Corresponding author
Correspondence to Ehigbai I. Oikeh .
Ethics declarations
Ethics approval.
Not applicable.
Consent for publication
Competing interests.
All authors declare that they have no competing interests.
Additional information
Publisher’s note.
Springer Nature remains neutral with regard to jurisdictional claims in published maps and institutional affiliations.
Rights and permissions
Open Access This article is licensed under a Creative Commons Attribution 4.0 International License, which permits use, sharing, adaptation, distribution and reproduction in any medium or format, as long as you give appropriate credit to the original author(s) and the source, provide a link to the Creative Commons licence, and indicate if changes were made. The images or other third party material in this article are included in the article's Creative Commons licence, unless indicated otherwise in a credit line to the material. If material is not included in the article's Creative Commons licence and your intended use is not permitted by statutory regulation or exceeds the permitted use, you will need to obtain permission directly from the copyright holder. To view a copy of this licence, visit http://creativecommons.org/licenses/by/4.0/ .
Reprints and permissions
About this article
Cite this article.
Oikeh, E.I., Oviasogie, F.E. & Omoregie, E.S. Quantitative phytochemical analysis and antimicrobial activities of fresh and dry ethanol extracts of Citrus sinensis (L.) Osbeck (sweet Orange) peels. Clin Phytosci 6 , 46 (2020). https://doi.org/10.1186/s40816-020-00193-w
Download citation
Received : 01 February 2020
Accepted : 03 July 2020
Published : 09 July 2020
DOI : https://doi.org/10.1186/s40816-020-00193-w
Share this article
Anyone you share the following link with will be able to read this content:
Sorry, a shareable link is not currently available for this article.
Provided by the Springer Nature SharedIt content-sharing initiative
- Citrus sinensis
- Orange peel
- Antimicrobial
- Phytonutrients
Information
- Author Services
Initiatives
You are accessing a machine-readable page. In order to be human-readable, please install an RSS reader.
All articles published by MDPI are made immediately available worldwide under an open access license. No special permission is required to reuse all or part of the article published by MDPI, including figures and tables. For articles published under an open access Creative Common CC BY license, any part of the article may be reused without permission provided that the original article is clearly cited. For more information, please refer to https://www.mdpi.com/openaccess .
Feature papers represent the most advanced research with significant potential for high impact in the field. A Feature Paper should be a substantial original Article that involves several techniques or approaches, provides an outlook for future research directions and describes possible research applications.
Feature papers are submitted upon individual invitation or recommendation by the scientific editors and must receive positive feedback from the reviewers.
Editor’s Choice articles are based on recommendations by the scientific editors of MDPI journals from around the world. Editors select a small number of articles recently published in the journal that they believe will be particularly interesting to readers, or important in the respective research area. The aim is to provide a snapshot of some of the most exciting work published in the various research areas of the journal.
Original Submission Date Received: .
- Active Journals
- Find a Journal
- Proceedings Series
- For Authors
- For Reviewers
- For Editors
- For Librarians
- For Publishers
- For Societies
- For Conference Organizers
- Open Access Policy
- Institutional Open Access Program
- Special Issues Guidelines
- Editorial Process
- Research and Publication Ethics
- Article Processing Charges
- Testimonials
- Preprints.org
- SciProfiles
- Encyclopedia
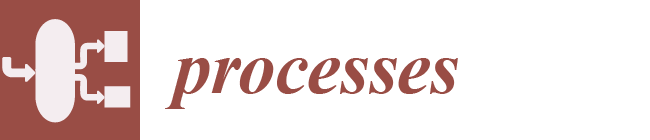
Article Menu

- Subscribe SciFeed
- Recommended Articles
- Google Scholar
- on Google Scholar
- Table of Contents
Find support for a specific problem in the support section of our website.
Please let us know what you think of our products and services.
Visit our dedicated information section to learn more about MDPI.
JSmol Viewer
Chemical and biological characterisation of orange ( citrus sinensis ) peel extracts obtained by subcritical water.
1. Introduction
2. materials and methods, 2.1. plant material, 2.2. chemicals and reagents, 2.3. subcritical water extraction, 2.4. total extraction yield, 2.5. total phenolics content (tpc), 2.6. total flavonoids content (tfc), 2.7. total antioxidant capacity, 2.8. dpph radical scavenging activity, 2.9. total carbohydrate content, 2.10. pectin content, 2.11. dietary fibre content, 2.12. phytochemical screening assay, 2.12.1. free flavonoids, 2.12.2. anthocyanins, 2.12.3. total tannins, 2.12.4. gallic tannins, 2.12.5. reducing sugars (fehling’s test), 2.12.6. cardiac glycosides, 2.12.7. alkaloids, 2.12.8. coumarins, 2.12.9. saponosides, 2.12.10. o -heterosides, 2.12.11. c -heterosides, 2.13. statistical analysis, 3.1. swe samples, 3.2. total extraction yield and polyphenol content in orange peel extracts, 3.3. antioxidant capacity and dpph radical scavenging activity of orange peel extracts, 3.4. carbohydrates in orange peel extracts, 3.5. phytochemical screening assay, 4. discussion, 5. conclusions, author contributions, data availability statement, acknowledgments, conflicts of interest.
- Cui, J.; Ren, W.; Zhao, C.; Gao, W.; Tian, G.; Bao, Y.; Lian, Y.; Zheng, J. The structure-property relationships of acid- and alkali-extracted grapefruit peel pectines. Carbohyd. Polym. 2020 , 229 , 115524. [ Google Scholar ] [ CrossRef ] [ PubMed ]
- Food and Agriculture Organization. Citrus Fruit Fresh and Processed ; Statistical Bulletin 2016; Food and Agriculture Organization of the United Nations: Rome, Italy, 2017; Available online: http://www.fao.org/3/a-i8092e.pdf (accessed on 19 February 2023).
- Singh, B.; Pal Singh, J.; Kaur, A.; Singh, N. Phenolic composition, antioxidant potential and health benefits of citrus peel. Food Res. Int. 2020 , 132 , 109114. [ Google Scholar ] [ CrossRef ] [ PubMed ]
- Negro, V.; Mancini, G.; Ruggeri, B.; Fino, D. Citrus waste as feedstock for biobased products recovery: Review on limonene case study and energy valorisation. Bioresour. Technol. 2016 , 214 , 806–815. [ Google Scholar ] [ CrossRef ] [ PubMed ]
- Sharma, K.; Mahato, N.; Hwan Cho, M.; Rok Lee, Y. Converting citrus wastes into value-added products: Economic and environmentaly friendly approaches. Nutrition 2017 , 34 , 29–46. [ Google Scholar ] [ CrossRef ]
- Senit, J.J.; Velasco, D.; Manrique, A.G.; Sanchez-Barba, M.; Toledo, J.M.; Santos, V.E.; Garcia-Ochoa, F.; Yustos, P.; Ladero, M. Orange peel waste upstream integrated processing to terpenes, phenolics, pectin and monosaccharides: Optimization approaches. Ind. Crop. Prod. 2019 , 134 , 370–381. [ Google Scholar ] [ CrossRef ]
- Satari, B.; Karimi, K. Citrus processing wastes: Environmental impacts, recent advances, and future perspectives in total valorisation. Resour. Conserv. Recycl. 2018 , 129 , 153–167. [ Google Scholar ] [ CrossRef ]
- Mandalari, G.; Bennett, R.N.; Bisignano, G.; Saija, A.; Dugo, G.; Lo Curto, R.B.; Waldron, K.W. Characterization of flavonoids and pectins from bergamot ( Citrus bergamia Risso) peel, a major byproduct of essential oil extraction. J. Agr. Food Chem. 2006 , 54 , 197–203. [ Google Scholar ] [ CrossRef ]
- Liu, N.; Li, X.; Zhao, P.; Zhang, X.; Qiao, O.; Huang, L.; Guo, L.; Gao, W. A review of chemical constituents and health-promoting effects of citrus peels. Food Chem. 2021 , 365 , 130585. [ Google Scholar ] [ CrossRef ]
- Alvarez, J.; Hooshdaran, B.; Cortazar, M.; Amutio, M.; Lopez, G.; Freire, F.B.; Haghshenasfard, M.; Hosseini, S.H.; Olazar, M. Valorization of citrus wastes by fast pyrolysis in a conical spouted bed reactor. Fuel 2018 , 224 , 111–120. [ Google Scholar ] [ CrossRef ]
- Negro, V.; Ruggeri, B.; Fino, D.; Tonini, D. Life cycle assessment of orange peel waste management. Resour. Conserv. Recycl. 2017 , 127 , 148–158. [ Google Scholar ] [ CrossRef ]
- Ben Hsouna, A.; Sadaka, C.; Generalić Mekinić, I.; Garzoli, S.; Švarc-Gajić, J.; Rodrigues, F.; Morais, S.; Moreira, M.M.; Ferreira, E.; Spigno, G.; et al. The chemical variability, nutraceutical value, and food-industry and cosmetic applications of citrus plants: A critical Review. Antioxidants 2023 , 12 , 481. [ Google Scholar ] [ CrossRef ]
- Ciriminna, R.; Fidalgo, A.; Delisi, R.; Tamburino, A.; Carnaroglio, D.; Cravotto, G.; Ilharco, L.M.; Pagliaro, M. Controlling the degree of esterification of citrus pectin for demanding application by selection of the source. ACS Omega 2017 , 2 , 7991–7995. [ Google Scholar ] [ CrossRef ]
- Zhang, J.; Wen, C.; Zhang, H.; Duan, Y.; Ma, H. Recent advances in the extraction of bioactive compounds with subcritical water: A review. Trends Food Sci. Techol. 2020 , 95 , 183–195. [ Google Scholar ] [ CrossRef ]
- Lachos-Perez, D.; Baseggio, A.M.; Mayanga-Torres, P.C.; Maróstica Junior, M.R.; Rostagno, M.A.; Martínez, J.; Forster-Carneiro, T. Subcritical water extraction of flavanones from defatted orange peel. J. Supercrit. Fluids 2018 , 138 , 7–16. [ Google Scholar ] [ CrossRef ]
- Lachos-Perez, D.; Baseggio, A.M.; Torres- Mayanga, P.C.; Ávila, P.F.; Tompsett, G.A.; Marostica, M.; Goldbeck, R.; Timko, M.T.; Rostagno, M.; Martinez, J.; et al. Sequential subcritical water process applied to orange peel for the recovery flavanones and sugars. J. Supercrit. Fluids 2020 , 160 , 104789. [ Google Scholar ] [ CrossRef ]
- Švarc-Gajić, J.; Cvetanović, A.; Segura-Carretero, A.; Linares, I.B.; Mašković, P. Characterisation of ginger extracts obtained by subcritical water. J. Supercrit. Fluids 2017 , 123 , 92–100. [ Google Scholar ] [ CrossRef ]
- Li, H.-B.; Cheng, K.-W.; Wong, C.-C.; Fan, K.-W.; Chen, F.; Jiang, Y. Evaluation of antioxidant capacity and total phenolic content of different fractions of selected microalgae. Food Chem. 2007 , 102 , 771–776. [ Google Scholar ] [ CrossRef ]
- Benmerzoug, A.; Švarc-Gajić, J.; Nastić, N.; Guettaf, S.; Harzallah, D. Subcritical water extraction of polyphenols from endemic Algerian plants with medicinal properties. APTEFF Acta Period. Technol. 2020 , 51 , 191–206. [ Google Scholar ] [ CrossRef ]
- Prieto, P.; Pineda, M.; Aguilar, M. Spectrophotometric quantitation of antioxidant capacity through the formation of a phosphomolybdenum complex: Specific application to the determination of vitamin E. Anal. Biochem. 1999 , 269 , 337–341. [ Google Scholar ] [ CrossRef ]
- Moreira, M.M.; Barroso, M.F.; Porto, J.V.; Ramalhosa, M.J.; Švarc-Gajić, J.; Estevinho, L.; Morais, S.; Delure-Matos, C. Potential of Portuguese vine shoot wastes as natural resources of bioactive compounds. Sci. Total Environ. 2018 , 634 , 831–842. [ Google Scholar ] [ CrossRef ] [ Green Version ]
- Benvenutti, L.; Ferreira Zielinski, A.A.; Salvador Ferreira, S.R. Subcritical water extraction (SWE) modified by deep eutectic solvent (DES) for pectin recovery from a Brazilian berry by-product. J. Supercrit. Fluids 2022 , 189 , 105729. [ Google Scholar ] [ CrossRef ]
- Bezus, B.; Esquivel, J.C.C.; Cavalitto, S.; Cavello, I. Pectin extraction from lime pomace by cold-active polygalacturonase-assisted method. Int. J. Biol. Macromol. 2022 , 209 , 290–298. [ Google Scholar ] [ CrossRef ] [ PubMed ]
- AOAC—Association of Official Analytical Chemists. International, Official Methods of Analysis of the Assosiation of Official Analytical Chemists International , 17th ed.; Association of Official Analytical Communities: Gaithersburg, MD, USA, 2000. [ Google Scholar ]
- Redhouane, B.; Khaled, B.; Hadjira, B.; Imene, I.; Abderrezak, K.; Fethia, F.; Švarc-Gajić, J. Evaluation of some biological activities of phenolic compounds obtained from two Algerian medicinal plants: Mentha rotundifolia and Satureja calamintha . APTEFF Acta Period. Technol 2020 , 51 , 87–102. [ Google Scholar ] [ CrossRef ]
- Shehata, M.G.; Awad, T.S.; Asker, D.; El Sohaimy, S.A.; Abd El-Aziz, N.M.; Youssef, M.M. Antioxidant and antimicrobial activities and UPLC-ESI-MS/MS polyphenolic profile of sweet orange peel extracts. Curr. Res. Food Sci. 2021 , 4 , 326–335. [ Google Scholar ] [ CrossRef ]
- Tomás-Navaro, M.; Vallejo, F.; Tomás-Barberán, F.A. Bioavailability and metabolism of citrus fruit beverage flavanones in humans. In Polyphenols in Human Health and Disease ; Watson, R.R., Preedy, V.R., Zibadi, S., Eds.; Elesvier: Amsterdam, The Netherlands, 2014; Volume 1, pp. 537–551. [ Google Scholar ]
- Pontifex, M.G.; Malik, M.; Connell, E.; Muller, M.; Vauzour, D. Citrus polyphenols in brain health and disease: Current perspectives. Front. Neurosci. 2021 , 15 , 640648. [ Google Scholar ] [ CrossRef ]
- Cory, H.; Passarelli, S.; Szeto, J.; Tamez, M.; Mattei, J. The role of polyphenols in human health and food systems: A mini-review. Front. Nutr. 2018 , 5 , 87. [ Google Scholar ] [ CrossRef ] [ Green Version ]
- Larrauri, J.A.; Rupérez, P.; Saura-Calixto, F. Effect of drying temperature on the stability of polyphenols and antioxidant activity of red grape pomace peels. J. Agric. Food Chem. 1997 , 45 , 1390–1393. [ Google Scholar ] [ CrossRef ]
- Antony, A.; Farid, M. Effect of temperatures on polyphenols during extraction. Appl. Sci. 2022 , 12 , 2107. [ Google Scholar ] [ CrossRef ]
- Palma, M.; Piñeiro, Z.; Barroso, C.G. Stability of phenolic compounds during extraction with superheated solvents. J. Chromatogr. A 2001 , 921 , 169–174. [ Google Scholar ] [ CrossRef ]
- Vergara-Salinas, J.R.; Pérez-Jiménez, J.; Torres, J.L.; Agosin, E.; Pérez-Correa, J.R. Effects of temperature and time on polyphenolic content and antioxidant activity in the pressurized hot water extraction of deodorized thyme ( Thymus vulgaris ). J. Agric. Food Chem. 2012 , 60 , 10920–10929. [ Google Scholar ] [ CrossRef ]
- Álvarez-Casas, M.; García-Jares, C.; Llompart, M.; Lores, M. Effect of experimental parameters in the pressurized solvent extractionof polyphenolic compounds from white grape marc. Food Chem. 2014 , 157 , 524–532. [ Google Scholar ] [ CrossRef ]
- Omboa, O.S.; Obafaye, R.O.; Salawu, S.O.; Boligon, A.A.; Athayde, M.L. HPLC-DAD phenolic caracterization and antioxidant activities of ripe and unripe sweet orange peels. Antioxidants 2015 , 4 , 498–512. [ Google Scholar ] [ CrossRef ] [ Green Version ]
- Nayak, B.; Dahmoune, F.; Moussi, K.; Remini, H.; Dairi, S.; Aoun, O.; Khodir, M. Comparison of microwave, ultrasound and accelerated-assisted solvent extraction for recovery of polyphenols from Citrus sinensis peels. Food Chem. 2015 , 187 , 507–516. [ Google Scholar ] [ CrossRef ]
- Lee, G.J.; Lee, S.Y.; Kang, N.-G.; Jin, M.H. A multi-faceted comparison of phytochemicals in seven citrus peels and improvement of chemical composition and antioxidant activity by steaming. LWT Food Sci. Technol. 2022 , 160 , 113297. [ Google Scholar ] [ CrossRef ]
- Selahvarzi, A.; Ramezan, Y.; Sanjabi, M.R.; Namdar, B.; Akbarmivehie, M.; Mirsaeedghazi, H.; Azarikia, F. Optimization of ultrasonic-assisted extraction of phenolic compounds from pomegranate and orange peels and their antioxidant activity in a functional drink. Food Biosci. 2022 , 49 , 101918. [ Google Scholar ] [ CrossRef ]
- Gómez-Urios, C.; Viñas-Ospino, A.; Puchades-Colera, P.; Blesa, J.; López-Malo, D.; Frígola, A.; Esteve, M.J. Choline chloride-based natural deep eutectic solvents for the extraction and stability of phenolic compounds, ascorbic acid, and antioxidant capacity from Citrus sinensis peel. LWT Food Sci. Technol. 2023 , 177 , 114595. [ Google Scholar ] [ CrossRef ]
- Makni, M.; Jemai, R.; Kriaa, W.; Chtourou, Y.; Fetovi, H. Citrus limon from Tunisia: Phytochemical and physicochemical properties and biological activities. BioMed Res. Int. 2018 , 2018 , 6251546. [ Google Scholar ] [ CrossRef ] [ Green Version ]
- Rizaldy, D.; Insanu, M.; Sabila, N.; Haniffadly, A.; Zahra, A.A.; Pratiwi, S.N.E.; Mudrika, S.N.; Hartati, R.; Fidrianny, I. Lemon ( Citrus limon L.): Antioxidative activity and its marker compound. Biointerface Res. Appl. Chem. 2023 , 13 , 21. [ Google Scholar ]
- Wang, F.; Chen, L.; Chen, H.; Chen, S.; Liu, Y. Analysis of flavonoid methabolites in citrus peels ( Citrus reticulata “Dahongpao”) using UPLC-ESI-MS/MS. Molecules 2019 , 24 , 2680. [ Google Scholar ] [ CrossRef ] [ Green Version ]
- Castro-Vazquez, L.; Alañón, M.E.; Rodríguez-Robledo, V.; Pérez-Coello, M.S.; Hermosín-Gutierrez, I.; Díaz-Maroto, M.C.; Jordán, J.; Galindo, M.F.; Arroyo-Jiménez, M.d.M. Bioactive flavonoids, antioxidant behaviour, and cytoprotective effects of dried grapefruit peels ( Citrus paradisi Macf.). Oxid. Med. Cell. Longev. 2016 , 2016 , 8915729. [ Google Scholar ] [ CrossRef ] [ Green Version ]
- Hassan, M.A.M.; Hussein, S.M. Chemical and technological studies on pink grapefruit ( Citrus paradise L.) peels. 1-Effect of storage conditions on gross chemical composition, phytochemical components and oil stability of pink grapefruit peels. World J. Dairy Food Sci. 2017 , 12 , 115–123. [ Google Scholar ]
- Ibañez, E.; Kubátová, A.; Señoráns, F.J.; Cavero, S.; Reglero, U.; Hawthorne, S.B. Subcritical water extraction of antioxidant compounds from rosemary plants. J. Agric. Food Chem. 2003 , 51 , 375–382. [ Google Scholar ] [ CrossRef ] [ PubMed ]
- Andrew, J.; Masetlwa, J.; Tesfaye, T.; Sithole, B. Beneficiation of eucalyptus tree barks in the context of an integrated biorefinery—Optimisation of accelerated solvent extraction (ASE) of polyphenolic compounds using response surface methodology. Sustain. Chem. Pharm. 2020 , 18 , 100327. [ Google Scholar ] [ CrossRef ]
- Hossain, M.B.; Barry-Ryan, C.; Martin-Diana, A.B.; Brunton, N.P. Optimisation of accelerated solvent extraction of antioxidant compounds from rosemary ( Rosmarinus officinalis L.), marjoram ( Origanum majorana L.) and oregano ( Origanum vulgare L.) using response surface methodology. Food Chem. 2011 , 126 , 339–346. [ Google Scholar ] [ CrossRef ] [ Green Version ]
- Budrat, P.; Shotipruk, A. Enhanced recovery of phenolic compounds from bitter melon ( Momordica charantia ) by subcritical water extraction. Sep. Purif. Technol. 2009 , 66 , 125–129. [ Google Scholar ] [ CrossRef ]
- Repajić, M.; Cegledi, E.; Kruk, V.; Pedisić, S.; Çinar, F.; Kovačević, D.B.; Žutić, I.; Dragojević-Uzelac, V. Accelerated solvent extraction as a green tool for the recovery of polyphenols and pigments from wild nettle leaves. Processes 2020 , 8 , 803. [ Google Scholar ] [ CrossRef ]
- Amo-Mensah, J.; Darko, G.; Borquaye, L.S. Anti-inflammatory and antioxidant activities of the root and bark extracts of Vitex grandifolia (Verbanaceae). Sci. Afr. 2020 , 10 , e00586. [ Google Scholar ] [ CrossRef ]
- Umdale, S.; Mahadik, R.; Otari, P.; Gore, N.; Mundala, P.; Ahire, M. Phytochemical composition, and antioxidant potential of Frerea indica Dalz.: A critically endangered, endemic and monotypic genus of the Western Ghats of India. Biocatal. Agric. Biotechnol. 2021 , 35 , 102080. [ Google Scholar ] [ CrossRef ]
- Bahadori, M.B.; Sarikurkcu, C.; Kocak, M.S.; Calapoglu, M.; Uren, M.C.; Ceylan, O. Plantago lanceolata as a source of health-beneficial phytochemicals: Phenolics profile and antioxidant capacity. Food Biosci. 2020 , 34 , 100536. [ Google Scholar ] [ CrossRef ]
- Da Cruz, J.D.; Mpalantinos, M.A.; Ramos, A.d.S.; Ferreira, J.L.P.; De Oliveira, A.A.; Netto Júnior, N.L.; Silva, J.R.d.A. Chemical standardization, antioxidant activity and phenolic contents of cultivated Alpinia zerumbet preparations. Ind. Crops Prod. 2020 , 151 , 112495. [ Google Scholar ] [ CrossRef ]
- Zhang, N.; Zhou, Q.; Fan, D.; Xiao, J.; Zhao, Y.; Cheng, K.-W.; Wang, M. Novel roles of hydrocolloids in foods: Inhibition of toxic maillard reaction and their harmful effects. Trends Food Techol. 2021 , 111 , 706–715. [ Google Scholar ] [ CrossRef ]
- Wu, B.; Chai, X.; He, A.; Huang, Z.; Chen, S.; Rao, P.; Ke, L.; Xiang, L. Inhibition of acrylamide toxicity in vivo by arginine-glucose maillard reaction products. Food Chem. Toxicol. 2021 , 154 , 112315. [ Google Scholar ] [ CrossRef ]
- Jia, W.; Guo, A.; Zhang, R.; Shi, L. Mechanism of natural antioxidants regulating advanced glycosylation end products of maillard reaction. Food Chem. 2023 , 404 , 134541. [ Google Scholar ] [ CrossRef ]
- Barrales, F.M.; Silveira, P.; Barbosa, P.d.P.M.; Ruviaro, R.; Paulino, B.N.; Pastore, G.M.; Macedo, G.A.; Martinez, J. Recovery of phenolic compounds from citrus by-products using pressurized liquids-an application to orange peel. Food Bioprod. Process. 2018 , 112 , 9–21. [ Google Scholar ] [ CrossRef ]
- Kroh, L.W. Caramelisation in food and beverages. Food Chem. 1994 , 51 , 373–379. [ Google Scholar ] [ CrossRef ]
- Chen, J.; Liu, F.; Ismail, B.B.; Wang, W.; Xu, E.; Pan, H.; Ye, X.; Liu, D.; Cheng, H. Effects of ethephon and low-temperature treatments on blood oranges ( Citrus sinensis L. Osbeck): Anthocyanin accumulation and volatile profile changes during storage. Food Chem. 2022 , 393 , 133381. [ Google Scholar ] [ CrossRef ]
- De Melo, L.F.M.; Aquino-Martins, V.G.D.Q.; Da Silva, A.P.; Rocha, H.A.O.; Scortecci, K.C. Biological and pharmacological aspects of tannins and potential biotechnological applications. Food Chem. 2023 , 414 , 135645. [ Google Scholar ] [ CrossRef ]
- Lyndem, S.; Sarmah, S.; Das, S.; Roy, A.S. Coumarin derivatives: Biomedical properties and interactions with carrier proteins. In Studies in Natural Products Chemistry ; Atta-ur-Rahman, Ed.; Elsevier: Amsterdam, The Netherlands, 2022; Volume 73, pp. 173–220. [ Google Scholar ]
- Tungmunnithum, D.; Thongboonyou, A.; Pholboon, A.; Yangsabai, A. Flavonoids and other phenolic compounds from medicinal plants for pharmaceutical and medicinal aspects: An overview. Medicines 2018 , 5 , 93. [ Google Scholar ] [ CrossRef ]
Click here to enlarge figure
Orange Peel Extract | Extraction Temperature (°C) | Extraction Time (min) |
---|---|---|
Extract 1 | 200 | 60 |
Extract 2 | 180 | 60 |
Extract 3 | 150 | 60 |
Extract 4 | 150 | 35 |
Extract 5 | 120 | 5 |
Orange Peel Extract | Yield (%) | TPC (mg GAE/g) | TFC (mg RE/g) |
---|---|---|---|
Extract 1 | 45.56 ± 0.53 | 27.58 ± 0.38 | 3.94 ± 0.03 |
Extract 2 | 44.68 ± 0.43 | 29.43 ± 0.11 | 5.29 ± 0.07 |
Extract 3 | 43.65 ± 0.39 | 36.16 ± 0.28 | 8.18 ± 0.08 |
Extract 4 | 42.26 ± 0.47 | 36.59 ± 0.56 | 7.88 ± 0.06 |
Extract 5 | 41.28 ± 0.46 | 45.45 ± 0.28 | 9.29 ± 0.08 |
Orange Peel Extract | TCC (g GE/g) | Pectin Content (%) | DFC (%) |
---|---|---|---|
Extract 1 | 0.14 ± 0.01 | 5.78 ± 0.55 | 0.47 |
Extract 2 | 0.30 ± 0.01 | 7.24 ± 0.84 | n.d. |
Extract 3 | 0.48 ± 0.02 | 15.05 ± 0.82 | n.d. |
Extract 4 | 0.38 ± 0.02 | 17.06 ± 1.64 | 0.24 |
Extract 5 | 0.43 ± 0.01 | 23.09 ± 0.90 | 0.26 |
Orange Peel Extract | |||||
---|---|---|---|---|---|
Chemical Classes | Extract 1 | Extract 2 | Extract 3 | Extract 4 | Extract 5 |
Free Flavonoids | + | ++ | +++ | +++ | +++ |
Anthocyanins | - | - | - | - | - |
Total Tannins | +++ | +++ | +++ | +++ | +++ |
Gallic Tannins | +++ | +++ | +++ | +++ | +++ |
Reducing Sugars | +++ | +++ | +++ | +++ | +++ |
Cardiac Glycosides | ++ | ++ | ++ | ++ | ++ |
Alkaloids | + | + | ++ | ++ | - |
Coumarins | + | + | + | + | + |
Saponosides | - | - | - | - | - |
O-Heterosides | +++ | +++ | +++ | +++ | +++ |
C-Heterosides | +++ | +++ | +++ | +++ | +++ |
Extraction Technique | TPC (mg GAE/g DW *) | TFC | Yield (%) | Reference |
---|---|---|---|---|
Orange peel | ||||
Decoction (water) | 9.40 | 4.20 mg QE/g DW | - | [ ] |
MAE (aqueous acetone) | 12.09 | - | - | [ ] |
UAE (aqueous acetone) | 10.35 | - | ||
ASE (aqueous acetone) | 6.26 | - | ||
CSE (aqueous acetone) | 10.21 | - | ||
Maceration (water) | 2.56 | 0.52 mg CE/g DW | 9.40 | [ ] |
Maceration (ethanol) | 3.45 | 0.80 | 10.90 | |
Maceration (methanol) | 3.24 | 0.52 | 15.56 | |
Maceration (acetone) | 3.06 | 0.58 | 8.23 | |
Maceration (petroleum ether) | 1.90 | 0.45 | 10.16 | |
Maceration (hexane) | 1.53 | 0.40 | 11.80 | |
High-temperature pretreatment; extraction with methanol | 22.4 | 12.7 mg RE/g DW | - | [ ] |
UAE (aqueous ethanol) | 1.86 | - | 11.00 | [ ] |
NADES (choline chloride-malic acid) | 10.53 | 0.95 mg CE/g DW | - | [ ] |
SWE | 45.45 | 9.29 mg RE/g DW | 41.28–45.56 | This work |
Lemon peel | ||||
Hydroethanolic extracts | 105–204 | 27–56 mg QE/g | 10.64–14.33 | [ ] |
Ethanolic, n-hexane, ethylacetate extracts | 8.9–15.2 | 2.49–28.9 mg QE/g | - | [ ] |
Tangerine peel | ||||
Methanolic extract | 122.5 | - | - | [ ] |
Grapefruit peel | ||||
Accelerated solvent extraction | 28–85 | - | - | [ ] |
Hexane:methanol:acetone (2:1:1) | 10.78 | - | - | [ ] |
The statements, opinions and data contained in all publications are solely those of the individual author(s) and contributor(s) and not of MDPI and/or the editor(s). MDPI and/or the editor(s) disclaim responsibility for any injury to people or property resulting from any ideas, methods, instructions or products referred to in the content. |
Share and Cite
Brezo-Borjan, T.; Švarc-Gajić, J.; Morais, S.; Delerue-Matos, C.; Rodrigues, F.; Lončarević, I.; Pajin, B. Chemical and Biological Characterisation of Orange ( Citrus sinensis ) Peel Extracts Obtained by Subcritical Water. Processes 2023 , 11 , 1766. https://doi.org/10.3390/pr11061766
Brezo-Borjan T, Švarc-Gajić J, Morais S, Delerue-Matos C, Rodrigues F, Lončarević I, Pajin B. Chemical and Biological Characterisation of Orange ( Citrus sinensis ) Peel Extracts Obtained by Subcritical Water. Processes . 2023; 11(6):1766. https://doi.org/10.3390/pr11061766
Brezo-Borjan, Tanja, Jaroslava Švarc-Gajić, Simone Morais, Cristina Delerue-Matos, Francisca Rodrigues, Ivana Lončarević, and Biljana Pajin. 2023. "Chemical and Biological Characterisation of Orange ( Citrus sinensis ) Peel Extracts Obtained by Subcritical Water" Processes 11, no. 6: 1766. https://doi.org/10.3390/pr11061766
Article Metrics
Article access statistics, further information, mdpi initiatives, follow mdpi.
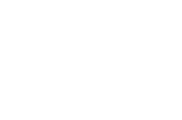
Subscribe to receive issue release notifications and newsletters from MDPI journals
Open Access is an initiative that aims to make scientific research freely available to all. To date our community has made over 100 million downloads. It’s based on principles of collaboration, unobstructed discovery, and, most importantly, scientific progression. As PhD students, we found it difficult to access the research we needed, so we decided to create a new Open Access publisher that levels the playing field for scientists across the world. How? By making research easy to access, and puts the academic needs of the researchers before the business interests of publishers.
We are a community of more than 103,000 authors and editors from 3,291 institutions spanning 160 countries, including Nobel Prize winners and some of the world’s most-cited researchers. Publishing on IntechOpen allows authors to earn citations and find new collaborators, meaning more people see your work not only from your own field of study, but from other related fields too.
Brief introduction to this section that descibes Open Access especially from an IntechOpen perspective
Want to get in touch? Contact our London head office or media team here
Our team is growing all the time, so we’re always on the lookout for smart people who want to help us reshape the world of scientific publishing.
Home > Books > Citrus - Research, Development and Biotechnology
The Orange Peel: An Outstanding Source of Chemical Resources
Submitted: 22 September 2020 Reviewed: 29 January 2021 Published: 30 September 2021
DOI: 10.5772/intechopen.96298
Cite this chapter
There are two ways to cite this chapter:
From the Edited Volume
Citrus - Research, Development and Biotechnology
Edited by Muhammad Sarwar Khan and Iqrar Ahmad Khan
To purchase hard copies of this book, please contact the representative in India: CBS Publishers & Distributors Pvt. Ltd. www.cbspd.com | [email protected]
Chapter metrics overview
1,126 Chapter Downloads
Impact of this chapter
Total Chapter Downloads on intechopen.com

Total Chapter Views on intechopen.com
Overall attention for this chapters
Citrus sinensis (L.) Osbeck is a very common cultivar belonging to the Rutaceae family. It is largely diffused in several areas of the world characterized by mild to warm climate conditions. Its abundant worldwide production (up to 107 Tons. per year) and consumption both as the edible part of the fruit and as several types of derivative products imply the production of a huge amount of waste, such as the fruit pomace. Several ways of recycling this material have been developed in recent years: employment as fertilizer, fodder ingredient, and even cloth material. However, the chemical added value of Citrus sinensis peel has been underestimated despite the diversified and significant content of useful chemicals, such as polyphenols, polymethoxylated phenols, glycosylated flavonoids, volatile and non-volatile terpenoids, pectins, enzymes, etc. This work aims to highlight the outstanding chemical potential of Citrus sinensis peel.
- biological activity
- Citrus sinensis
- essential oil
- orange peels
- polymethoxyphenols
Author Information
Gianfranco fontana *.
- Department of Biological, Chemical and Pharmaceutical Sciences and Technologies (STEBICEF), University of Palermo, Palermo, Italy
*Address all correspondence to: [email protected]
1. Introduction
Citrus sinensis (CS) (L.) Osbeck is a perennial species growing in warm climate areas of the world and largely employed as food in form of fresh fruit, with a global production of ca. 6.7X10 7 tons. per year (TPY) in 2016 [ 1 ], or as a processed derivative (ca. 1.85x10 7 TPY) such as juice, marmalade, flavor, fragrance and coloring additive, pectin.
CS is an evergreen tree, 3 to 9 mts. high with sparingly barbed branches, alternate leaves with toothed blades differently shaped, oval or elliptical, connected to the stem by winged-petioles. Axillary flowers are present singly or in whorls of 6 and possess 5 white petals and up to about 25 yellow colored stamens. The pericarp of CS has a spherical or oval shape of 6–10 cm diameter with the color changing from green to yellow-orange during the ripening; the endocarp containing juice sac glands is enclosed within a wrinkled epicarp or exocarp or flavedo containing a great number of essential oil glands protected by a waxy epidermis. Below the flavedo is the albedo, also called mesocarp, a white filamentary tissue composed of tubular-like cells.
The principal industrial application of CS is the production of frozen concentrated juice. The procedure of juice extraction eventually accompanied by the extraction of the essential oil, implies the generation of a major “by-product” constituted by a pomace, mainly containing peels, accounting for up to around 60% w/w of the original fruit mass processed [ 2 ]. This huge amount of biomass does pose serious environmental concerns because of its high level of total organic carbon (TOC) and biological oxygen demand (BOD) that make disposal procedures rather complex and demanding from both the legal and industrial points of view. This is because there is an increasing trend to modify the way of approaching this problem by reconsidering the post-production orange pomace more like a by-product rather than a waste. In the last years, many producers have subjected this material to processings involving partial acidic fermentation, drying, and packaging to biologically and chemically stabilize the biomass before its application as animal feed in zootechnics, soil conditioners in agriculture, or the manufacturing of compost and biogas [ 2 ].
Beyond the standard workup of the Citrus sinensis peel (CSP) waste, new perspectives have been being opened in the context of the high chemical added value of the CSP [ 3 , 4 , 5 ] also by the complete knowledge of the rich metabolomics profile of this species. The use of CS peel has been proposed for a variety of purposes that include the production of antioxidant-enriched dietary supplements in veterinary [ 6 ], the preparation of human dietary supplements, and nutraceuticals such as citric acid [ 7 ] and flavonoids [ 8 , 9 ]. The extract of CS peel is the source of a huge variety of phytochemicals and has been investigated on several applications including its chemotherapeutic and chemopreventive potential for several relevant human pathologies, such as cancer [ 10 , 11 ] and obesity [ 12 ]. The extraction procedures vary in function of the main components that have to be obtained: from the simple cold pressing of pomace and the extraction with water to obtain highly hydroxylated compounds to the employment of mixtures organic protic solvent/water and finally low polar organic solvents such as Chloroform and Ethyl acetate to obtain polymethoxylated phenols (PMF, see below). New extraction technologies such as ultrasounds and microwaves may help to obtain better extraction yields.
In the following sections, the chemical structures and the biological effects of these compounds will be discussed.
2. The chemistry of Citrus sinensis peel
2.1 essential oils.
The essential oil (EO) is mainly obtained from the CS peel as a major by-product of the juice production process by a cold-pressing method that can provide the intact blend of compounds without losing the lighter, more volatile, components of the complex mixture that can be lost in the standard EO extraction procedure that is the hydrodistillation. The last one is mainly used in small scale applications, for example in research laboratories.
The chemical composition of CSP EO [ 13 , 14 , 15 ] is reported in Table 1 . As it can be seen, the major component is D-Limonene, accompanied by several minor components belonging to the classes of monoterpene alkenes, oxygenated monoterpenes including alcohol aldehydes and esters, sesquiterpenes as well as linear alkanes and aldehydes. This rather complex blend accounts for the numerous deal of biological activities reported for the CSP EO [ 14 , 15 , 16 ], which include anthelmintic, anti-aflatoxigenic [ 17 ], antibacterial [ 18 , 19 , 20 ], anticarcinogenic, antifungal [ 21 ], antioxidant [ 17 ], anti-tumor [ 22 ], anxiolytic [ 23 ], food preservative [ 24 ], hepatocarcinogenesis suppressant, insecticidal and larvicidal [ 25 ], pain relief and relaxant [ 26 ]. It can be argued that the main effects are due to the presence of the major component Limonene that showed several bioactivities when tested as pure compound [ 27 ]. However, it is possible that synergistic effects due to the combination of Limonene with other minor components may be speculated and should have to be demonstrated.
Comp. | Comp. name | % | Compound. | Comp. name | % |
---|---|---|---|---|---|
Aromadendrene | 0.01 | β-Linalool | 0.4–5.6 | ||
δ-Amorphene | 0.05 | β-Myrcene | 1.3–3.3 | ||
D-Cadinene | 0.01–0.03 | Neral | 0.1–1.3 | ||
δ-3-Carene | 0.18 | Neryl acetate | 0.02 | ||
β-Citral | 0.12–0.15 | Nonanal | 0–0.1 | ||
L-(+)-Citronellal | 0.01–0.1 | Nootkatone | 0.01 | ||
Citronellyl acetate | 0.01 | -β-Ocimene | 0.03–0.26 | ||
α-Copaene | 0.04 | Octanal | 0.02–0.8 | ||
α-Cubebene | 0.02–0.26 | Perillaldehyde | 0.03 | ||
β-Cubebene | 0.03 | α-Phellandrene | 0.02–0.07 | ||
Decanal | 0.04–0.4 | α-Pinene | 0.49–0.59 | ||
n-Dodecanal | 0.06 | (+)-Sabinene | 0.2–1.0 | ||
β-Elemene | 0.01–0.02 | γ-Terpinene | 0–1.21 | ||
Geranial | 0–1.8 | γ-Terpineol | 0.04–008 | ||
Germacrene-D | 0.02–0.08 | α-Terpineol | 0.07–0.42 | ||
β-Gurjurene | 0.01 | Terpinolene | 0–0.08 | ||
Hexadecanol | 0.04 | α-Thujene | 0.04 | ||
D-Limonene | Ca. 95 | ||||
L-Limonene | 0.02 | ||||
-Limonene oxide | 0.01 |
Composition of C. sinensis essential oil obtained from peels.
2.2 Polyphenols
2.2.1 flavanoids.
Polyphenols extracted from the CS peel belongs to the general structural categories of flavanones ( Figure 1a ), flavones ( Figure 1b ), flavonols ( Figure 1b ), with and without sugar moieties attached to one or more of the hydroxyl groups [ 28 ]. It is worthy of particular mention the rarely occurring class of C-glycolflavones ( Figure 1b , compounds 63 – 65 , 85 , 86 ).

Chemical structures of flavonoids from C. sinensis peels.
These compounds are produced in vivo from the biogenetic mixed pathway of the Acetate and Shikimate that implies the enantiospecific formation of the basic aromatic bicyclic framework of the flavanone, from which a huge number of flavonoids originate employing selective enzymatic hydroxylations, methylations, and glycosylation steps. As can be seen from the structures shown in Figure 1 , most of the chemical entities found in the peel extract contain several methoxy fragments that decorate the carbon skeleton. This characteristic makes those molecules to get a rather apolar character that explains their presence in the hydrophobic environment of the waxy peel. On the contrary, compounds containing a major number of hydroxyl groups are less present in the peel and are instead more significantly concentrated in the juice of the pericarp. However, some glycosylated compounds are present in the peel. In these molecules, the aglicone bears a monosaccharide unit (mainly glucose) or a disaccharide, in most of the cases being Rutinose ( 91 ) – Rhamnosyl (α1 → 6) glucose – or Neohesperidose ( 92 )- Rhamnosyl (α1 → 2) glucose ( Figure 2 ).

Chemical structures of the disaccharides most commonly bound to flavonoids of C. sinensis peel.
The composition of the peel extracts described in the literature may slightly vary depending on the cultivar and the region of harvesting but some general points are common, that is the presence of the high amount of bioactive polymethoxyflavonoids [ 29 , 30 ](PMF) some of which are rather ubiquitous, e.g. Nobiletin 53 , Sinensetin 51 , 3′,4′,3,5,6,7,8-Heptamethoxyflavone 55 ; some other compounds containing one to six methoxy groups in place of the hydroxyl groups are present at variable amounts. The presence of one or more residual hydroxy groups in the molecule may result in a higher bioavailability and in other general differences in their mechanism of biological and therapeutic actions [ 30 , 31 ].
The biological role of these secondary metabolites in the plant is still matter of debate. It has been proposed their involvement in the mechanism of defense of the fruits exposed to the attack of phytopathogens, such as Phytophthora citrophthora [ 32 ].
Further, the composition of the PMF blend can be employed for the chemiotaxonomic characterization of the Citrus genus [ 33 ].
However, it needs to be stressed that in many cases the reported compounds were recognized by mass spectrometry and electronic spectroscopy. It is not always a matter of simplicity to discern the exact structure of a given PMF and to discriminate between different regioisomers, normally quite similar in terms of mass and electronic spectra, if an isolation procedure is not conducted and followed by a complete bi-dimensional NMR characterization. Significant differences in the extract composition do arise also in consequence of the extraction method; non-polar solvents such as Methanol, Chloroform Ethyl acetate let to obtain PMFs-rich extracts while, on the other hand, hydroalcoholic and aqueous extracts do contain a low concentration of PMFs and a higher concentration of un-methylated polyphenols as well as glycosylated compounds.
The biological activities disclosed for the flavonoids extracted from CSP are variegated. They include antioxidant [ 9 , 34 , 35 , 36 , 37 , 38 , 39 ], anti-inflammatory [ 40 , 41 ], antimicrobial [ 39 , 42 , 43 , 44 ], antimalarial [ 45 ], antitrypanosomal [ 46 ], cardio-protective [ 47 ], anti-osteoporosis [ 48 ], anti-ulcer [ 49 ], vascular protective [ 50 ], anti-diabetes [ 51 , 52 ], hepatoprotective [ 53 , 54 ], neurotrophic [ 55 ], anti-adipogenesis and anti-obesity [ 56 , 57 , 58 ], anti-hypertensive [ 59 ], cataract prevention [ 60 ], sun protection [ 61 ], metabolic syndrome control [ 62 ]. Further, it has been demonstrated [ 63 ] that while both flavonoid set 40 , 42 , 43 and the PMFs 51 – 53 were able to inhibit the anion transportin polypeptide OATP2B1 in HEK293 cells, only the PMF group displayed this inhibitory activity also for the OATP1B1 and OATP1B3 carriers.
The most abundant PMF occurring in CSP, Nobiletin 53 , was proven to possess sevral bioactivities, such as antioxidant, anti-inflammatory, cancer preventive [ 64 ] and also a significant protective effect in vivo against the endotoxic shock [ 65 ] and ethanol-induced acute gastric lesions [ 66 ] in mice. Further, compound 53 demonstrated the capacity to induce autophagy in human keratinocyte HaCaT cells [ 67 ], vasodilatator effect in the rat aorta [ 68 ] and to protect the intestinal barrier from the demages induced by dextran sulfate sodium [ 69 ].
PMFs can be considered as especially promising lead compounds for cancer therapy as asignificant cytotoxic activity has been demonstrated toward a number of cancer cells [ 70 , 71 ] with several mechanisms of action [ 72 , 73 ]; the cytotypes investigated include MCF-7 [ 73 , 74 , 75 , 76 ], Hs578T triple-negative breast cancer [ 73 , 77 ]; colon cancer cells CaCo-2 [ 19 ], LoVo [ 78 ], HTC-116 [ 79 , 80 ] and HT-29 [ 79 , 81 ]; lung cancer cells A549 [ 80 , 82 ], H460 [ 82 , 83 ], H1299 [ 82 , 83 ]; gastric cancer cell lines AGS, BGC-823, and SGC-7901 [ 84 ]; leukemia cells HL-60 [ 85 ]. However, data regarding a possible antitumor activity in vivo are still rather uncommon. An interesting example is the case of the significant reduction of the intestinal tumor mass in ApcMin/+ mice treated with a CSP extract containing various PMF [ 86 ]. Further, CSP extract and pure Naringin 47 were tested for their efficacy against a YM1 esophageal cancer in an animal model [ 87 ].
Given the development of pharmacological applications of CSP extract components, further investigations are needed to better understand the bioavailability, safety, and efficacy of these compounds in humans. Most of the data reported concern in vitro experimentations or animal model tests. For example, the toxicity of Hesperidin 40 was evaluated [ 88 ] in Sprague Dawley rats showing a 50% lethal dose (LD50) of about 5 g/Kg body weight (BW) and a lowest-observed-adverse-effect level (LOAEL) of ca. 1 g/Kg BW.
In general, it should be emphasized as the body of evidence concerning the actual efficacy of sweet orange-derived compounds in human health is still far to be exhaustive. For example, while this work is under typewriting, a severe acute respiratory syndrome pandemic due to a COVID-19 virus is in act and a big deal of research has been being directed toward antiviral remedies and therapies. Research on nutraceuticals is not an exception and in particular some authors have shown by computational and molecular docking methods how Hesperidin 40 , the most abundant polyphenol obtained from C. sinensis , would be able to bind the spyke protein of this virus thus inhibiting its activity [ 89 ]. Despite their undoubted interest, these results need to be further investigated with different experimental approaches.
The pharmacological potential of pure Hesperidin 40 was also investigated for several relevant human morbidity, such as cancer, hypertension, and ulcer [ 90 ].
2.2.2 Hydroxy-acids
Several hydroxylated carboxylic acids belonging to several structural sub-classes are present foremostly in the extract obtained with mixed hydro-organic solvents, such as MeOH/water and EtOH / water [ 37 , 38 , 51 , 78 ]; these include the aliphatic Ascorbic, Citric, Kojic, Lactic, and L-Malic acids; the aromatic 4-Hydroxybenzoic, Protocatechulic, and Gallic acids. Further, the cinnamyl compounds ( Figure 3 ) Cinnamic ( 93 ), p-Cumaric ( 94 ), Caffeic ( 95 ), Ferulic ( 96 ), Sinapinic ( 97 ) acids, and Artepillin ( 98 ) were identified in some CSP extracts that showed relevant biological activities, such as antioxidant [ 34 , 37 , 38 ] and antidiabetes [ 51 ].

Chemical structures of cinnamic acids extracted from C. sinensis peels.
These organic acids are mainly found in free form but in some cases, they are esterified with a variety of alcoholic compounds, such as Ethanol in Ethyl gallate 99 [ 51 ], 2-Phenylethanol in Phenylethyl ester of Caffeic acid 100 [ 51 ] and (−)-Quinic acid in Chlorogenic acid 101 [ 51 ]. An interesting ester derivative ( 102 ) in which the anomeric hydroxyl of Glucose is esterified with a O-Caffeylsinapoyl acid unit was found in the methanolic extract of a Greek cultivar of C. sinensis [ 34 ].
It was shown [ 38 ] that the antioxidant properties of a CSP extract better correlated with the total phenols content (TPC) of the sample rather than with its total flavonoid content (TFC), as it can be expected from the known relevant antioxidant character of hydroxycynamic derivatives.
2.2.3 Coumarins
Coumarins are aromatic compounds biogenetically related to the o-hydroxysubstituted cynamic acids from which originate by the intramolecular condensation between the carboxylic and the o-hydroxy groups. These compounds are most commonly encountered in other species of Citrus taxa [ 91 ], such as C. aurantium (bitter orange), C. limon , (lemon), C. limetta (lime), C. paradisi (grapefruit) and only a few molecules of this class were Isolated from extracts of CSP endowed with activity against osteoporosis [ 48 ] and antioxidant [ 92 ]; these compounds are shown in Figure 4 . As coumarins are relatively less common in C. sinensis cultivars compared to other species of the Citrus taxa, their rarity can be considered as a chemotaxonomic marker for C. sinensis .

Chemical structure of coumarins extracted from C. sinensis peels.
2.2.4 Catechins
The NADPH dependent bioreduction of flavanols is the biogenetic origin of this class of compounds, present as minor constituents in CSP extract possessing significant antioxidant activity [ 38 ]; they are the two enantiomeric forms Catechin 113 and Epicatechin 114 , together with Epigallocatechin 115 ( Figure 5 ).

Chemical structure of catechins from C. sinensis peels.
2.3 Pectins
Pectins [ 93 ] are chemically definable as complex mixtures of polyglyconic acids in which a linear polymeric backbone is structured by a series of α (1 → 4) linkages ( Figure 6 ). The main sugar monomer is always Galacturonic acid with the presence of possible heterogeneous domains of other sugars such as Xylogalacturonan and Rhamnogalacturonan-I. A variable amount of the free carboxy functions may be esterified with methyl groups, while the hydroxy groups at C-2 and C-3 positions of the sugar monomers may be acetylated. Even though the primary structure of the main chain is linear, a possible degree of ramification, depending on the pectin source, may also be found. The differences in the pectins composition and structures, depending on their natural source, do confer them different physio-chemical properties, such as water solubility, sol–gel concentrations, etc. On the ground of the degree of methylation of the acid moieties, pectins are classified as “low methoxyl” (LMP, -COOMe/-COOH <50% mol.) or as “high methoxyl” (> 50% mol). A simplified representation of pectin structure is given in Figure 6 .

Minimal representation of a Homopolygalacturonic acid domain of the linear primary pectin structure with a 1/3 Mol. /Mol. Esterification degree.
Pectins find many applications in the food and drug industry as a thickening and gelling agents, excipients, and colloidal stabilizers [ 93 ].
As it has been already mentioned, the extraction method does affect the structure and the properties of the final product; the traditional acidic water extraction implies a certain degree of hydrolytic deterioration, so that new extraction technologies have been being investigated to improve the quality of the final pectins, that is microwave-assisted extraction (MAE) [ 94 ] and ultrasounds assisted extraction (USAE) [ 35 , 95 ].
2.4 Enzymes
As it can be easily argued, the CSP cellular system, whose genomic profile has been fully characterized [ 96 ], is the site of a complex network of enzymatic activity. Some of the enzymes of CSP have been characterized and employed in many applications.
The acetylesterase (international enzymatic classification: EC 3.1.1.6) from CSP is known since 1947 [ 97 ] and was isolated and characterized [ 98 ]. The acetylesterase activity of the partially purified enzyme was used for the removal of the acetyl group at the 3 positions of β-lactamic antibiotics 116 [ 98 ] ( Figure 7a ). Further, the whole CSP, as well as pomace from the industrial waste of the orange juice production, was successfully employed to catalyze several relevant biotransformations [ 99 ] such as the conversion of Geranyl acetate 118 to Geraniol 119 ( Figure 7b ) and the di-acetoxynaphtalene derivative 120 to the vitamin k1 precursor 121 ( Figure 7c ).

Chemical reactions biocatalysed by enzymes from C. sinensis peels.
Recently, partial purification and functional characterization of a Uronic acid oxidase from CSP was accomplished [ 100 ]; this enzyme promotes the oxidation by O 2 of Galacturonic acid 122 to Galactaric acid 123 ( Figure 7d ).
2.5 Miscellaneous
2.5.1 highly lipophilic compounds.
The waxy environment of flavedo in CSP does contain several long-chain saturated and unsaturated compounds: alkanes, fatty acids, waxes, higher terpenoids.
Tetracosane, Tetratriacontanoic acid, and Ethyl pentacosanoate were identified in CSP of a Pineapple variety [ 101 ]. Further, some carotenoids were identified in the CSP extract obtained with a solvent mixture composed of Ethanol, Ethyl acetate, Petroleum ether 1: 1:1 [ 102 ]. This complex blend of carotenoids includes α- and β-Carotene, Phytoene, Phytofluene, (all-E)- and (9Z)-Violoxanthin, (all-E)-Neoxanthin, (13Z)-, (13Z’)- and (all-E)-Lutein, (9Z)-Zeaxanthin, (all-E)-Zeaxanthin; the mono and di-esters of violaxanthin, antheroxanthins, Xanthophyll, β-Citraurin with various fatty acids, including Lauraic, Myristic, Oleic, Palmitic, Stearic. The composition of the blend has been correlated with the maturity stage of the fruit.
2.5.2 Peptides
Three cyclic peptides have been isolated from the hot water extract of CSP and were structurally characterized by FAB-MS and 2D-NMR techniques [ 103 ]. Their amino-acidic sequences, including a mostly lipophlic heptapeptide 124 , a di-hydroxylated heptapeptide 125, and a Glutamate-rich octapeptide 126 , are reported in Figure 8 .

Primary structure of cyclic peptide isolated from the C. sinensis peels.
3. Conclusions
The chemical richness of the primary and secondary metabolome of C. sinesnis species is undoubtedly impressive. Thousands of different compounds belonging to dozens of structural classes have been isolated and described. The most deeply investigated are sure, on one hand, the mixtures of volatile compounds composing the blend of the essential oil and, on the other hand, polyphenols, especially flavonoids.
The chemical composition of the extract from the exocarp of C. sinensis does differ from the composition of juice, or leaf extracts for some aspects [ 104 ]: the presence of a higher amount of more lipophilic compounds such as polymethoxy-flavonoids, r carotenoids, higher alkanes; a lesser extent of lighter terpenoids, a lower content of glycosylated compounds, the absence of cyanidins and sterols.
It is also a matter of fact that several interesting bioactivities were disclosed in the last years for the C. sinensis extracts that have been variously associated with the well-recognized beneficial effects that regular sweet oranges consumption may have on human health. However, a great deal of research work is still needed to clarify the molecular basis and the mechanism of these chemopreventive effects and to relate them with precise chemical entities that can be recognized as valuable nutraceuticals, as it is already the case for the well-established antioxidants Ascorbic acid, Hesperidin, Hesperetin, Quercetin, etc.
Conflict of interest
The author declares no conflict of interest.
- 1. Food and Agriculture Organization of the United nations: Citrus fruit fresh and processed - Statistical Bulletin 2016 [Internet]. 2017. Available from: http://www . http://www.fao.org/3/a-i8092e.pdf [Accessed: 2020-12-16]
- 2. Tamburino V, Zama DA. I sottoprodotti dell’industria di trasformazione: il pastazzo di agrumi. In: Vacante V, editor. Citrus - Trattato di agrumicoltura. Il Sole 24 Ore-Edagricole; 2009. p. 459–470
- 3. Putnik P, Kovačević DB, Jambrak AR, Barba FJ, Cravotto G, Binello A, Lorenzo JM, Shpigelman A. Innovative “Green” and Novel Strategies for the Extraction of Bioactive Added Value Compounds from CitrusWastes—A Review. Molecules. 2017:22:680–704. DOI: 10.3390/molecules22050680
- 4. Senit JJ, Velasco D, Gomez Manrique A, Sanchez-Barba M, Toledo LM, Santos VE, Garcia-Ochoa F, Yustos P, Ladero M. Orange peel waste upstream integrated processing to terpenes, phenolics, pectin and monosaccharides: Optimization approaches. Ind. Crops & Prod. 2019:134:370–381. DOI: 10.1016/j.indcrop.2019.03.060
- 5. Lamine M, Gargouri M, Rahali FZ, Mliki A. Recovering and Characterizing Phenolic Compounds From Citrus By-Product: A Way Towards Agriculture of Subsistence and Sustainable Bioeconomy. Waste Biomass Valor. 2020. DOI: 10.1007/s1264 9-020-01306 -9
- 6. Williams CA. Specialized dietary supplements. In: Geor RJ, Harris PA, Coenen M, editors. Equine Applied and Clinical Nutrition. Saunders; 2013. p. 351–366. DOI: 10.1016/C2009-0-39370-8
- 7. Patel S, Shukla S. Fermentation of Food Wastes for Generation of Nutraceuticals and Supplements. In: Frias J, Martinez-Villaluenga C, Peñas E, editors. Fermented Foods in Health and Disease Prevention. Academic Press; 2016. p. 707–734. DOI: 10.1016/C2014-0-01734-0
- 8. Khan N, Mongas M, Urpi-sarda M, Llorach R, Andres-La Cueva C. Contribution of Bioactive Foods and Their Emerging Role in Immunomodulation, Inflammation, and Arthritis. In: Watson RR. Preedy VR, editors. Bioactive Food as Dietary Interventions for Arthritis and Related Inflammatory Diseases. Academic Press; 2013. p. 43–65. DOI: 10.1016/C2011-0-07467-7
- 9. Rehman Z. Citrus peel extract – A natural source of antioxidant. Food Chem. 2006;99:450–454. DOI: 10.1016/j.foodchem.2005.07.054
- 10. Abe S, Fan K, Ho CT, Ghai G, Yang K. Chemopreventive Effects of Orange Peel Extract (OPE) II. OPE Inhibits Atypical Hyperplastic Lesions in Rodent Mammary Gland. J. Med. Food 2007;10:18–24; DOI: 10.1089/jmf.2006.0215
- 11. Lai CS, Li S, Miyauchi Y, Suzawa M, Hob CT, Pan MH. Potent anti-cancer effects of citrus peel flavonoids in human prostate xenograft tumors. Food Funct. 2013;4:944–949; DOI: 10.1039/c3fo60037h
- 12. Tung YC, Chang WT, Li S, Wu JC, Badmeav V, Ho CT, Pan MH. Citrus peel extracts attenuated obesity and modulated gut 1 microbiota in a high-fat diet-induced obesity mice. Food Funct. 2018;9:3363–3373; 10.1039/C7FO02066J
- 13. Qiao Y, Jun Xie B, Zhang Y, Zhang Y, Fan G, Yao XL, Pan SY. Characterization of Aroma Active Compounds in Fruit Juice and Peel Oil of Jinchen Sweet Orange Fruit ( Citrus sinensis (L.) Osbeck) by GC-MS and GC-O. Molecules. 2008;13:1333–1344; DOI: 10.3390/molecules13061333
- 14. Dosoky NS, Setzer WN. Biological Activities and Safety of Citrus spp. Essential Oils. Int. J. Mol. Sci. 2018;19:1966; DOI: 10.3390/ijms19071966
- 15. Ettoumi KY, Zouambia Y, Moulai-Mostefa N. Chemical composition, antimicrobial and antioxidant activities of Algerian Citrus sinensis essential oil extracted by hydrodistillation assisted by electromagnetic induction heating. J Food Sci Technol DOI: 10.1007/s13197-020-04808-5
- 16. Leherbauer I, Stappen I. Selected essential oils and their mechanisms for therapeutic use against public health disorders. An overview. Z. Naturforsch. 2020;75: 205–223
- 17. Singh P, Shukla R, Prakash B, Kumar A, Singh S, Mishra PK, Dubey NK. Chemical profile, antifungal, antiaflatoxigenic and antioxidant activity of Citrus maxima Burm. and Citrus sinensis (L.) Osbeck essential oils and their cyclic monoterpene, DL-limonene. Food Chem. Toxicol. 2010;48:1734–1740; DOI:10.1016/j.fct.2010.04.001
- 18. O’Bryan CA, Crandall PG, Chalova VI, Ricke SC. Orange Essential Oils Antimicrobial Activities against Salmonella spp. J. Food Sc. 2008;73:M264-M267; DOI: 10.1111/j.1750-3841.2008.00790.x
- 19. Fisher K, Phillips CA. The effect of lemon, orange and bergamot essential oils and their components on the survival of Campylobacter jejuni, Escherichia coli O157, Listeria monocytogenes , Bacillus cereus and Staphylococcus aureus in vitro and in food systems. J. Appl. Microbiol. 2006;101:1232–1240; DOI:10.1111/j.1365-2672.2006.03035.x
- 20. Muthaiyan A, Martin EM, Natesan S, Crandall PG, Wilkinson BJ, Ricke SC. Antimicrobial effect and mode of action of terpeneless cold-pressed Valencia orange essential oil on methicillin-resistant Staphylococcus aureus . J. Appl. Microbiol. 2012;112:1020–1033; DOI:10.1111/j.1365-2672.2012.05270.x
- 21. Hung PV, Chi PTL, Phi NTL. Comparison of antifungal activities of Vietnamese citrus essential oils. Nat. Prod. Res. 2013;27:506–508; DOI: 10.1080/14786419.2012.706293
- 22. Najar B, Shortrede JE, Pistelli L, Buhagiar J. Chemical Composition and in Vitro Cytotoxic Screening of Sixteen Commercial Essential Oils on Five Cancer Cell Lines. Chem. Biodiversity 2020;17:e1900478; DOI: 10.1002/cbdv.201900478
- 23. Faturi CB, Leite JR, Alves PB, Canton AC, Teixeira-Silva F. Anxiolytic-like effect of sweet orange aroma in Wistar rats. Prog. Neuro-Psychoph. 2010;34:605–609; DOI:10.1016/j.pnpbp.2010.02.020
- 24. Uçar Y. Antioxidant Effect of Nanoemulsions Based on Citrus Peel Essential Oils: Prevention of Lipid Oxidation in Trout. Eur. J. Lipid Sci. Technol. 2020;122:1900405–1900419; DOI: 10.1002/ejlt.201900405
- 25. Ciriminna R, Meneguzzo F, Pagliaro M. Orange Oil. In: Nollet LML, Rathore HS editors. Green Pesticides Handbook – Essential Oils for Pest Control. CRC Press; 2017. p. 215–265
- 26. Lehrner J, Eckersberger C, Walla P, Pötsch G, Deecke L. Ambient odor of orange in a dental office reduces anxiety and improves mood in female patients. Physiology & Behavior 2000;71:83–86
- 27. Anandakumar P, Kamaraj S, Vanitha MK. D-limonene: A multifunctional compound with potent therapeutic effects. J. Food Biochem. 2020;45:e13566; DOI: 10.1111/jfbc.13566
- 28. Li S, Lo CY, Ho CT. Hydroxylated Polymethoxyflavones and Methylated Flavonoids in Sweet Orange ( Citrus sinensis ) Peel. J. Agric. Food Chem. 2006;54:4176–4185. DOI: 0.1021/jf060234n
- 29. Gao Z, Gao W, Zeng SL, Li P, Liu EH. Chemical structures, bioactivities and molecular mechanisms of citrus polymethoxyflavones. J. Funct. Foods 2018;40:498–509; DOI: 10.1016/j.jff.2017.11.036
- 30. Owis AL. Citrus Polymethoxyflavones: Biofunctional Molecules of Therapeutic Interest. Studies in Nat. Prod. Chem. 2019;59: 509–530. DOI: 10.1016/B978-0-444-64179-3.00015-3
- 31. Lai CS, Wu JC, Ho CT, Pan MH. Disease chemopreventive effects and molecular mechanisms of hydroxylated polymethoxyflavones. Biofactors 2015;41:301–313; DOI 10.1002/biof.1236
- 32. Del Río JA, Gómez P, Báidez AG, Arcas MC, Botía JM, Ortuño A. Changes in the Levels of Polymethoxyflavones and Flavanones as Part of the Defense Mechanism of Citrus sinensis (Cv. Valencia Late) Fruits against Phytophthora citrophthora. J. Agric. Food Chem. 2004;52:1913–1917; DOI: 10.1021/jf035038k
- 33. Mizuno M, Iinuma M, Ohara M, Tanaka T, Iwamasa M. Chemotaxonomy of the Genus Citrus Based on Polymethoxyflavones. Chem. Pharm. Bull. 1991;39:945–949; DOI: 10.1248/cpb.39.942
- 34. Kanaze FI, Termentzi A, Gabrieli C, Niopas I, Georgarakis M, Kokkaloua E. The phytochemical analysis and antioxidant activity assessment of orange peel ( Citrus sinensis ) cultivated in Greece–Crete indicates a new commercial source of hesperidin. Biomed. Chromatogr. 2009;23:239–249. DOI: 10.1002/bmc.1090
- 35. Montero-Calderon A, Cortes C, Zulueta A, Frigola A, Esteve MJ. Green solvents and Ultrasound- Assisted Extraction of bioactive orange ( Citrus sinensis ) peel compounds. Scientific Reports. 2019;9:16120–16128. DOI: 10.1038/s41598-019-52717-1
- 36. Manthey JA. Fractionation of Orange Peel Phenols in Ultrafiltered Molasses and Mass Balance Studies of Their Antioxidant Levels. J. Agric. Food Chem. 2004;52:7586–7592. DOI: 0.1021/jf049083j
- 37. Anagnostopoulou MA, Kefalas P, Kokkalou E,. Assimopoulou AN, Papageorgiou VP. Analysis of antioxidant compounds in sweet orange peel by HPLC–diode array detection–electrospray ionization mass spectrometry. Biomed. Chromatogr. 2005;19:138–148. DOI: 10.1002/bmc.430
- 38. Liew SS, Ho WY, Yeap SK, Bin Sharifudin SA. Phytochemical composition and in vitro antioxidant activities of Citrus sinensis peel extracts. PeerJ. 2018;6:e5331. DOI: 10.7717/peerj.5331
- 39. Guo C, Shan Y, Yang Z, Zhang L, Ling W, Liang Y, Ouyang Z, Zhong B, Zhang J. Chemical composition, antioxidant, antibacterial, and tyrosinase inhibition activity of extracts from Newhall navel orange ( Citrus sinensis Osbeck cv. Newhall) peel. J Sci Food Agric 2020;100:2664–2674. DOI: 10.1002/jsfa.10297
- 40. Gosslau A, Chen KY, Ho CT, Li S. Anti-inflammatory effects of characterized orange peel extracts enrichedwith bioactive polymethoxyflavones. Food Sci. Human Well. 2014;3:26–35; DOI:10.1016/j.fshw.2014.02.002
- 41. Hagenlocher Y, Feilhauer K, Schäffer M, Bischoff SC, Lorentz A. Citrus peel polymethoxyflavones nobiletin and tangeretin suppress LPS- and IgE-mediated activation of human intestinal mast cells. Eur J Nutr 2017;56:1609–1620; DOI 10.1007/s00394-016-1207-z
- 42. Liu L, Xu X, Cheng D, Yao X, Pan S. Structure−Activity Relationship of Citrus Polymethoxylated Flavones and Their Inhibitory Effects on Aspergillus niger. J. Agric. Food Chem. 2012;60:4336–4341. DOI: 10.1021/jf3012163
- 43. Shetty SB, Mahin-Syed-Ismail P, Varghese S, Thomas-George B, Kandathil-Thajuraj P, Baby O, Haleem S, Sreedhar S, Devang-Divakar D. Antimicrobial effects of Citrus sinensis peel extracts against dental caries bacteria: An in vitro study. J Clin Exp Dent. 2016;8:e70–7. DOI:10.4317/jced.52493
- 44. Ortuño A, Báidez A, Gómez P, Arcas MC, Porras I, García-Lidón A, Del Río JA. Citrus paradisi and Citrus sinensis flavonoids: Their influence in the defence mechanism against Penicillium digitatum. Food Chem. 2006;98:351–358; DOI:10.1016/j.foodchem.2005.06.017
- 45. Bagavan A, Rahuman AA, Kamaraj C, Kaushik NK, Mohanakrishnan D, Sahal D. Antiplasmodial activity of botanical extracts against Plasmodium falciparum . Parasitol Res 2011;108:1099–1109. DOI: 10.1007/s00436-010-2151-0
- 46. Nakanishi M, Hino M, Yoshimura M, Amakura Y, Nomoto H. Identification of sinensetin and nobiletin as major antitrypanosomal factors in a citrus cultivar. Exp. Parasitol. 2019;200:24–29; DOI: 10.1016/j.exppara.2019.03.008
- 47. Lara Testai L, Calderone V. Nutraceutical Value of Citrus Flavanones and Their Implications in Cardiovascular Disease. Nutrients. 2017;9:502–515. DOI:10.3390/nu9050502
- 48. Shalaby NMM, Abd-Alla HI, Ahmed HH, Basoudan N. Protective effect of Citrus sinensis and Citrus aurantifolia against osteoporosis and their phytochemical constituents. J. Med. Plant. Res. 2011;5:579–588
- 49. Selmi S, Rtibi K, Grami D, Sebai H, Marzouki L. Protective effects of orange ( Citrus sinensis L.) peel aqueous extract and hesperidin on oxidative stress and peptic ulcer induced by alcohol in rat. Lipids in Health and Disease. 2017;16:152–164. DOI: 10.1186/s12944-017-0546-y
- 50. Chen PY, Li S, Koh YC, Wu JC, Yang MJ, Ho CT, Pan MH. Oolong Tea Extract and Citrus Peel Polymethoxyflavones Reduce Transformation of L-Carnitine to Trimethylamine-N-Oxide and Decrease Vascular Inflammation in L-Carnitine Feeding Mice. J. Agric. Food Chem. 2019;67:7869–7879. DOI: 10.1021/acs.jafc.9b03092
- 51. Sathiyabama RG, Gandhia GR, Denadaib M, Sridharanc G, Jothic G, Sasikumard P, Siqueira Quintanse JdS, Narain N, Cuevas LE, Melo Coutinho HD, Barbosa Ramose AG, Quintans-Júniore LJ, Queiroz Gurgela R. Evidence of insulin-dependent signalling mechanisms produced by Citrus sinensis (L.) Osbeck fruit peel in an insulin resistant diabetic animal model. Food Chem. Toxicol. 2018;116:86–99. DOI: 10.1016/j.fct.2018.03.050
- 52. Ahmeda OM, Hassanb MA, Abdel-Twabc SM, Abdel Azeem MN. Navel orange peel hydroethanolic extract, naringin and naringenin have anti-diabetic potentials in type 2 diabetic rats. Biomed. & Pharmacotherapy 2017;94:197–205. DOI: 10.1016/j.biopha.2017.07.094
- 53. Ahmed OM, Fahim H, Ahmed HY, Al-Muzafar HM, Ahmed RR , Amin KA, El-Nahass ES, Abdelazeem WH. The Preventive Effects and the Mechanisms of Action of Navel Orange Peel Hydroethanolic Extract, Naringin, and Naringenin in N-Acetyl-p-aminophenol-Induced Liver Injury in Wistar Rats. Oxidative Medicine and Cellular Longevity. 2019; DOI: 10.1155/2019/2745352
- 54. Kim TW, Lee DR, Choi BK, Kang HK, Jung JY, Lim SW, Hwan Yang S, Suh JW. Hepatoprotective effects of polymethoxyflavones against acute and chronic carbon tetrachloride intoxication. Food Chem. Toxicol. 2016;91: 91–99; DOI: 10.1016/j.fct.2016.03.004
- 55. Chiu SP, Wu MJ, Chen PY, Ho YR, Tai MH, Ho CT, Yen JH. Neurotrophic action of 5-hydroxylated polymethoxyflavones: 5-demethylnobiletin and gardenin A stimulate neuritogenesis in PC12 cells. J. Agric. Food Chem. 2013;61:9453–9463; DOI: 10.1021/jf4024678
- 56. Lai CS, Ho MH, Tsai ML, Li S, Badmaev V, Ho CT, Pan MH. Suppression of Adipogenesis and Obesity in High-Fat Induced Mouse Model by Hydroxylated Polymethoxyflavones. J. Agric. Food Chem. 2013;61:10320–10328; DOI: 10.1021/jf402257t
- 57. Sergeev IN, Li S, Ho CT, Rawson NE, Dushenkov S. Polymethoxyflavones Activate Ca2+-Dependent Apoptotic Targets in Adipocytes. J. Agric. Food Chem. 2009;57:5771–5776; DOI:10.1021/jf901071k
- 58. Yu Wang Y, Lee PS, Chen YF, Ho CT, Pan MH. Suppression of Adipogenesis by 5-Hydroxy-3,6,7,8,30,40-Hexamethoxyflavone from Orange Peel in 3T3-L1 Cells. J. Med. Food 2016;19:830–835; DOI: 10.1089/jmf.2016.0060
- 59. Li GJ, Wang J, Cheng YJ, Tan X, Zhai YL, Wang Q, Gao FJ, Liu GL, Xin Zhao X, Hua Wang H. Prophylactic Effects of Polymethoxyflavone-Rich Orange Peel Oil on N ω -Nitro-L-Arginine-Induced Hypertensive Rats. Appl. Sci. 2018;8:752–768; DOI:10.3390/app8050752
- 60. Miyata Y, Oshitari T, Okuyama Y, Shimada A, Takahashi H, Natsugari H, Kosano H. Polymethoxyflavones as agents that prevent formation of cataract: Nobiletin congeners show potent growth inhibitory effects in human lens epithelial cells. Bioorg. Med. Chem. Lett. 2013;23:183–187; DOI: 10.1016/j.bmcl.2012.10.133
- 61. Li G, Tan F, Zhang Q, Tan A, Cheng Y, Zhou Q, Liu M, Tan X, Huang L, Rouseff R, Wu H, Zhao X, Liang G, Zhao X. Protective effects of polymethoxyflavone-rich cold-pressed orange peel oil against ultraviolet B-induced photoaging on mouse skin. J. Funct. Foods 2020; 67:103834–103844; DOI: 10.1016/j.jff.2020.103834
- 62. Zeng SL, Li SZ, Xiao PT, Cai YY, Chu C, Chen BZ, Li P, Li J, Liu EH. Citrus polymethoxyflavones attenuate metabolic syndrome by regulating gut microbiome and amino acid metabolism. Sci. Adv. 2020;6: eaax6208; DOI: 10.1126/sciadv.aax6208
- 63. Bajraktari-Sylejmani G, Weiss J. Potential Risk of Food-Drug Interactions: Citrus Polymethoxyflavones and Flavanones as Inhibitors of the Organic Anion Transporting Polypeptides (OATP) 1B1, 1B3, and 2B1. Eur. J. Drug Metab. Pharm. 2020;45:809–815; DOI: 10.1007/s13318-020-00634-4
- 64. Li S, Wang H, Guo L, Zhao H, Ho CT. Chemistry and bioactivity of nobiletin and its metabolites. J. Funct. Food 2014;6;2–10. DOI: 10.1016/j.jff.2013.12.011
- 65. Li W, Wang X, Niu X, Zhang H, He Z, Wang Y,1 Zhi W, Liu F. Protective Effects of Nobiletin Against Endotoxic Shock in Mice Through Inhibiting TNF-α, IL-6, and HMGB1 and Regulating NF-κB Pathway. Inflammation 2016;39:786–797. DOI: 10.1007/s10753-016-0307-5
- 66. Li W, Wang X, Zhi W, Zhang H, He Z, Wang Y, Liu F, Niu X, Zhang X. The gastroprotective effect of nobiletin against ethanol-induced acute gastric lesions in mice: impact on oxidative stress and inflammation. Immunopharm. Immunot. 2017;39:354–363; DOI: /10.1080/08923973.2017.1379088
- 67. Abe S, Hirose S, Nishitani M, Yoshida I, Tsukayama M, Tsuji A, Yuasa K. Citrus peel polymethoxyflavones, sudachitin and nobiletin, induce distinct cellular responses in human keratinocyte HaCaT cells. Biosci. Biotechnol. Biochem. 2018;82:2064–2071; DOI: 10.1080/09168451.2018.1514246
- 68. Kaneda H, Otomo R, Sasaki N, Omi T, Sato T, Kaneda T. Endothelium-independent vasodilator effects of nobiletin in rat aorta. J. Pharmacol. Sci. 2019;140:48–53; DOI: 10.1016/j.jphs.2019.04.004
- 69. Wen X, Zhao H, Wang L, Wang L, Du G, Guan W, Liu J, Cao X, Jiang X, Tian J, Wang M, Ho CT, Li S. Nobiletin Attenuates DSS-Induced Intestinal Barrier Damage through the HNF4α-Claudin-7 Signaling Pathway. J. Agric. Food Chem. 2020;68:4641–4649; DOI: 10.1021/acs.jafc.0c01217
- 70. Koolaji N, Shammugasamy B, Schindeler A, Dong Q, Dehghani F, Valtchev P. citrus Peel Flavonoids as Potential Cancer Prevention Agents. Current Developments In Nutrition. 2020;4:ID nzaa025;DOI: 10.1093/cdn/nzaa025
- 71. Tung, YC, Chou YC, Hung WL, Cheng AC, Yu RC, Ho CT, Pan MH. Polymethoxyflavones: Chemistry and Molecular Mechanisms for Cancer Prevention and Treatment. Curr. Pharmacol. Rep. 2019;5:98–113; DOI: 10.1007/s40495-019-00170-z
- 72. Wang L, Wang J, Fang L, Zheng Z, Zhi D, Wang S, Li S, Ho CT, Zhao H. Anticancer Activities of Citrus Peel Polymethoxyflavones Related to Angiogenesis and Others. Biomed. Res. Int. 2014; Article ID 453972; DOI: 10.1155/2014/453972
- 73. Chan EWC, Soo OYM, Tan YH, Wong SK, Chan HT. Nobiletin and tangeretin (citrus polymethoxyflavones): an overview on their chemistry, pharmacology and cytotoxic activities against breast cancer. J. Chin. Pharm. Sci. 2020;29:443–454; DOI: 10.5246/jcps.2020.07.042
- 74. Sergeev IN, Ho CT, Li S, Colby J, Dushenkov S. Apoptosis-inducing activity of hydroxylated polymethoxyflavones and polymethoxyflavones from orange peel in human breast cancer cells. Mol. Nutr. Food Res. 2007;51:1478–1484. DOI: 10.1002/mnfr.200700136
- 75. Rahideh ST, Keramatipour M, Nourbakhsh M, Koohdani F, Hoseini M, Talebi S, Shidfar F. Comparison of the effects of Nobiletin and Letrozole on the activity and expression of aromatase in MCF-7 breast cancer cell line. Biochem. Cell. Biol. 2017;95:468–473;DOI: 10.1139/bcb-2016-0206
- 76. Sergeev IN, Li S, Colby J, Ho CT, Dushenkov S. Polymethoxylated flavones induce Ca2+−mediated apoptosis in breast cancer cells. Life Sci. 2006;80:245–253; DOI: 10.1016/j.lfs.2006.09.006
- 77. Borah N, Gunawardana S, Torres H, McDonnell S, Van slambrouck S. 5,6,7,3′,4′,5'-Hexamethoxyflavone inhibits growth of triple-negative breast cancer cells via suppression of MAPK and Akt signaling pathways and arresting cell cycle. Int. J. Oncol. 2017;51:1685–1693; DOI: 10.3892/ijo.2017.4157
- 78. Ademosun AO, Oboh G, Passamonti S, Tramer F, Ziberna L, Augusti Boligon A, Athayde ML. Inhibition of metalloproteinase and proteasome activities in colon cancer cells by citrus peel extracts. J Basic Clin Physiol Pharmacol 2015;26: 471–477. DOI: 10.1515/jbcpp-2013-0127
- 79. Kaur J, Kaur G. An insight into the role of citrus bioactives in modulation of colon cancer. J. Funct. Foods. 2015;13:239–261;DOI: 10.1016/j.jff.2014.12.043
- 80. Qiu P, Cui Y, Xiao H, Han Z, Ma H, Tang Y, Xu H, Zhang L. 5-Hydroxy polymethoxyflavones inhibit glycosaminoglycan biosynthesis in lung and colon cancer cells. J. Funct. Foods. 2017;30:39–47. DOI: 10.1016/j.jff.2017.01.008
- 81. Silva I, Estrada MF, Pereira CV, Bento da Silva A, Bronze MR, Alves PM,. Duarte CMM, Brito C, Serra AT. Polymethoxylated Flavones from Orange Peels Inhibit Cell Proliferation in a 3D Cell Model of Human Colorectal Cancer. Nutrition and Cancer. 2018;70:257–266. DOI: 10.1080/01635581.2018.1412473
- 82. Charoensinphon N, Qiu P, Dong P, Zheng J, Ngauv P, Cao Y, Li S, Ho CT, Xiao H. 5-Demethyltangeretin inhibits human nonsmall cell lung cancer cell growth by inducing G2/M cell cycle arrest and apoptosis. Mol. Nutr. Food Res. 2013;57:2103–2111; DOI: 10.1002/mnfr.201300136
- 83. Xiao H, Yang CS, Li S, Jin H, Ho CT, Patel T. Monodemethylated polymethoxyflavones from sweet orange ( Citrus sinensis ) peel inhibit growth of human lung cancer cells by apoptosis. Mol. Nutr. Food Res. 2009;53:398–406; DOI 10.1002/mnfr.200800057
- 84. Wang Y, Chen Y, Zhang H, Chen J, Cao J, Chen Q, Li X, Sun C. Polymethoxyflavones from citrus inhibited gastric cancer cell proliferation through inducing apoptosis by upregulating RARβ, both in vitro and in vivo. Food Chem. Toxicol. 2020;146:111811–111821; DOI: 10.1016/j.fct.2020.111811
- 85. Li S, Pan MH, Lai CS, Lo CY, Dushenkovc S, Ho CT. Isolation and syntheses of polymethoxyflavones and hydroxylated polymethoxyflavones as inhibitors of HL-60 cell lines. Bioorg. Med. Chem. 2007;15:3381–338. DOI: 10.1016/j.bmc.2007.03.021
- 86. Fan K, Kurihara N, Abe S, Ho CT, Ghai G, Yang K. Chemopreventive Effects of Orange Peel Extract (OPE) I. OPE Inhibits Intestinal Tumor Growth in ApcMin+ Mice. Med. Food 2007;10:11–17. DOI: 10.1089/jmf.2006.0214
- 87. Tajaldinia M, Samadia F, Khosravib A, Ghasemnejadd A, Asadie J. Polymethoxylated Protective and anticancer effects of orange peel extract and naringin in doxorubicin treated esophageal cancer stem cell xenograft tumor mouse model. Biomed. Pharm. 2020;121:e109594. DOI: 10.1016/j.biopha.2019.109594
- 88. Lia Y, Kandhare AD, Mukherjee AA, Bodhankar SL. Acute and sub-chronic oral toxicity studies of hesperidin isolated from orange peel extract in Sprague Dawley rats. Regul. Toxicol Pharm. 2019;105:77–85. DOI: 10.1016/j.yrtph.2019.04.001
- 89. Bellavite P, Donzelli A. Hesperidin and SARS-CoV-2: New Light on the Healthy Function of Citrus Fruits. Antioxidants. 2020;9:742–760. DOI: 10.3390/antiox9080742
- 90. Ganeshpurkar A, Saluja A. The pharmacological potential of hesperidin. Indian J. Biochem. Biophys. 2019;56:287–300
- 91. Murray RDH. Naturally Occurring Plant Cumarins. In: Herz W, Grisebach H, Kirby GW, editors. Progress in the Chemistry of Organic Natural Products. Springer-Verlag. New York; 1978. p. 199–400
- 92. Mohamed TK. Chemical constituents and antioxidant activity of Citrus paradisi (star-ruby red grapefruit) and Citrus sinensis (blood sweet orange) Egyptian cultivars. Asian J. Chem. 2004:887815
- 93. Cunha AG, Gandini A. Turning polysaccharides into hydrophobic materials: a critical review. Part 2. Hemicelluloses, chitin/chitosan, starch, pectin and alginates. Cellulose. 2010;17:1045–1065. DOI: 10.1007/s10570-010-9435-5
- 94. Sua DL, Li PJ, Quekc SY, Huang ZQ, Yuan YJ, Li GY, Shan Y. Efficient extraction and characterization of pectin from orange peel by a combined surfactant and microwave assisted process. Food Chem. 2019;286:1–7. DOI: 10.1016/j.foodchem.2019.01.200
- 95. Hosseini SS, Khodaiyan F, Kazemi M, Najari Z. Optimization and characterization of pectin extracted from sour orange peel by ultrasound assisted method. Int. J. Biol. Macromol. 2019;125:621–629. DOI: 10.1016/j.ijbiomac.2018.12.096
- 96. Jiao WB, Huang D, Xing F, Hu Y, Deng XX, Xu Q, Chen LL. Genome-wide characterization and expression analysis of genetic variants in sweet oranged. The Plant Journal 2013;75:954–964. DOI: 10.1111/tpj.12254
- 97. Jansen EF, Jang R, Mac Donnel LR. Citrus acetylesterase. Arch Biochem. 1947;15:415–431
- 98. Pasta P, Verga R, Zambianchi F, Daminati M. Acetyl Esterase from Mediterranean Oranges: Partial Purification, Immobilisation and Biotransformations. Biocatal. & Biotrasf. 2004;22:221–224. DOI: 10.1080/10242420410001697089
- 99. Fontana G, Bruno M, Maggio A, Rosselli S. Functional investigation and applications of the acetylesterase activity of the Citrus sinensis (L.) Osbeck peel. Nat. Prod. Res. 2020; DOI: 10.1080/14786419.2020.1737055
- 100. Wei Y, Tan YL, Ang FL, Zhao H. Identification and Characterization of Citrus Peel Uronic Acid Oxidase. ChemBioChem 2020;21:797–800. DOI: 10.1002/cbic.201900546
- 101. Rani G, Yadav L, Kalidhar SB. Chemical Examination of Citrus sinensis Flavedo Variety Pineapple. Indian J. Pharm. Sci. 2009;71:677–679
- 102. Lux PE, Carle R, Zacarías L, Rodrigo MJ, Schweiggert RM, Steingass CB. Genuine Carotenoid Profiles in Sweet Orange [ Citrus sinensis (L.) Osbeck cv. Navel] Peel and Pulp at Different Maturity Stages. J. Agric. Food Chem. 2019; 67:13164–13175. DOI: 10.1021/acs.jafc.9b06098
- 103. Matsubara Y, Yusa T, Sawabe A, Itzuka Y, Takekuma S, Yoshida Y. Structures of New Cyclic Peptides in Young Unshiu ( Citrus unshiu Marcov.), Orange ( Citrus sinensis Osbeck.) and Amanatzu ( Citrus natzudaidai ) Peelings. Agric. Biol. Chem. 1991;55:2923–2929
- 104. Favela-Hernández JMJ, González-Santiago O, Ramírez-Cabrera MA. Esquivel-Ferriño PC, Camacho-Corona MdR. Chemistry and Pharmacology of Citrus sinensis . Molecules. 2016;21, 247–271. DOI: 10.3390/molecules21020247
© 2021 The Author(s). Licensee IntechOpen. This chapter is distributed under the terms of the Creative Commons Attribution 3.0 License , which permits unrestricted use, distribution, and reproduction in any medium, provided the original work is properly cited.
Continue reading from the same book
Edited by Muhammad Sarwar Khan
Published: 03 November 2021
By Mayumi Minamisawa
431 downloads
By Ana M. Cavaco, Dário Passos, Rosa M. Pires, Maria ...
614 downloads
By Ersin Demir, Hülya Silah and Nida Aydogdu
548 downloads
IntechOpen Author/Editor? To get your discount, log in .
Discounts available on purchase of multiple copies. View rates
Local taxes (VAT) are calculated in later steps, if applicable.
Support: [email protected]
SYSTEMATIC REVIEW article
The biological effect of orange ( citrus sinensis l.) by-products on metabolic biomarkers: a systematic review.
- 1 Centro Universitario del Sur, Universidad de Guadalajara, Guadalajara, Mexico
- 2 Centro de Investigación en Alimentación y Desarrollo, A.C., (CIAD), Hermosillo, Sonora, Mexico
Industrial processing of citrus fruits generates an important amount of wastes that evoke environmental damage. Orange is the main citrus fruit consumed worldwide, and after its use, approximately more than a half of the fruit remains as by-products, which comprise important bioactive compounds useful for the development of promising nutraceuticals for the treatment of non-communicable diseases. This study aimed to gather scientific evidence about the biological effects of orange by-products using a systematic review. A total of 14 studies that were carried out in rodent models in the last 10 years were retrieved from PubMed and ScienceDirect databases. Studies that used another animal species, another type of citrus, or a combination of orange with other citrus were excluded. The risk of bias was assessed by using the SYRCLE RoB tool, and the results obtained are shown in an informative table, which showed that most of the studies used a pathological model of chronic diseases. We found that the peel is the most used agri-food by-product, and that it has the potential of reducing the levels of triglycerides, total cholesterol, glucose, and systolic blood pressure. However, to clinically assess these effects, these results need to be tested in future in humans. The included studies on the use of orange by-products strengthen the global sustainable food agenda. It is important to consider new research directions about the use of citrus fruit residues since it not only impacts the problem of its disposal but also provides solutions to eliminate the resulting contamination.
Introduction
Chronic non-communicable diseases (NCDs) are a group of conditions that represents long-term health consequences and treatments, and overall, these are the principal cause of death and disability worldwide ( Pan American Health Organization, 2022 ). In 2022, NCDs such as cancer, diabetes, respiratory diseases, and cardiovascular diseases are the principal causes of death worldwide, representing 74% of cases ( World Health Organization, 2022 ). These four NCDs are contribute to about 80% of premature deaths associated with these diseases ( Pan American Health Organization, 2022 ). Metabolic risk factors of these conditions are overweight, obesity, hypertension, hyperglycemia, and hyperlipidemia, due to tobacco and alcohol use, lack of physical activity, and unhealthy diet ( Pan American Health Organization, 2022 ; World Health Organization, 2022 ). The World Health Organization-recommended “best buys” provide evidence of cost-effective actions for the prevention and control of NCDs, where a change in eating behavior and the promotion of a healthy diet are considered ( Pan American Health Organization, 2022 ). In addition to these actions, the consumption of functional foods can also reduce the risk for developing NCDs ( Plasek et al., 2019 ). Recently, the interest in natural sources to prevent and treat non-communicable diseases has increased, in part due to the need for cost-effective treatments. Diverse fruits and their by-products are natural products and are considered safe, with less side effects and toxicity than drugs ( Ahmed et al., 2017 ).
Among them, Citrus L. is a group of fruits that include several products, such as oranges, mandarins, lemons, grapefruits, citron, bergamots, fingered citron, limes, and others. The internal structure of this fruits consist of an endocarp, flavedo, and albedo ( Garcia-Castello et al., 2022 ). Oranges ( Citrus sinensis L.) are the most cultivated citrus (with over 40% of exportations), followed by tangerines, lemons, and grapefruits. Juices, oils, and citric acid are the main products extracted from citrus fruits. After the extraction of these products, the generated wastes are often discarded or used as animal feed ( Food Agriculture Organization of the United Nations, 2022 ).
The global production of oranges in January 2021/22 was approximately over 48 million tons, with Brazil, China, the European Union, Mexico, and the United States being the main producers. In several countries, oranges are mainly used for extracting juice, representing 1.7 million tons of its production until January 2021/22 ( United States Department of Agriculture, 2022 ). Some important compounds present in orange fruit are folic acid, thiamine, riboflavin, niacin, pantothenic acid, B6, potassium, phosphorus, calcium, iron, magnesium, sodium, ascorbic acid, amino acids, flavonoids, and phenolic compounds ( U.S. Department of Agriculture, 2019 ).
After its use, more than a half of the fruit remains as a by-product. The orange peel represents the highest discarded waste ( Olabinjo et al., 2017 ; Ayala et al., 2021 ), which is an important source of soluble sugar, pectin, ascorbic acid, fiber, and phenolic compounds ( Parmar and Kar, 2008 ; Ezejiofor et al., 2011 ; Ahmed et al., 2017 ; Ashraf et al., 2017 ; Sathiyabama et al., 2018 ). Seeds are another most discarded waste, which contains an important quantity of oil, protein, potassium, sodium, calcium, phosphorous, iron, ascorbic acid, oxalate, alkaloids, fatty acids, phytosterols, tocopherols, and fiber ( Okoye et al., 2011 ; El-safy et al., 2012 ; Malacrida et al., 2012 ; Zayed et al., 2018 ; Adubofuor et al., 2021 ). Orange pomace is yet another waste with a large number of bio-functional compounds; however, its use requires special attention because its large amount of moisture makes it the most perishable waste due to its high susceptibility to microbial spoilage ( Afrin et al., 2022 ). The proximate composition of orange by-products (including peel, seed, albedo, and flavedo) includes ~60–70 of dietary fiber, 5.9–8.9 protein, and 1.8–4.5 g/100g dry matter of lipids, and 534 mg GAE/100 g of dry matter of phenolic compounds ( Fernández-López et al., 2009 ; de Moraes Crizel et al., 2013 ; de Castro et al., 2020 ). These by-products can be revalorized as a natural source of vitamins, pectin, polyphenols, or essential oils with important health effects ( Garcia-Castello et al., 2022 ). Therefore, this review aimed to gather scientific evidence about the biological effects of orange by-products, and to discuss the proposed mechanism of action.
Materials and methods
Studies retrieved from two databases were searched in this systematic review using the PRISMA 2020 statement ( Page et al., 2021 ).
Search strategy and information sources
PubMed and ScienceDirect were the two databases used for the search of information of this review. The bibliographic search was carried out in English from March 2022 to May 2022.
The following combination of keywords were sought: “orange” OR “citrus sinensis” AND “peel” OR “seed” OR “waste” AND “triglycerides” OR “cholesterol” OR “glucose” OR “arterial pressure.” A total of 12 combinations of search terms were used. The filters applied to each database were as follows: articles published in the last 10 years (2012–2022) and “research articles.”
Eligibility criteria
This review included research studies that have evaluated the biological effects of orange ( Citrus sinensis L.) by-products conducted in rodents. The inclusion criterion was studies written in English that evaluated the effect exerted by the administration of orange by-products on metabolic biomarkers. The exclusion criteria are as follows: articles with results other than those of interest or with incomplete results; articles written in languages other than English; studies used animal species other than rodents, studies used citrus other than orange, or studies used a combination of orange with other citrus.
Selection process and data collection
The selected articles were systematically reviewed and analyzed by two authors individually and in an independent manner for the effect of orange by-products on metabolic biomarkers such as triglycerides, total cholesterol, HDL cholesterol, glucose levels, and arterial pressure. Duplicated articles were eliminated from the final review. The data extracted from each article were organized and are presented in tables. In addition to the by-product effect on metabolic biomarkers, this review included data on the characteristics of the experimental model and the sample of the by-product used.
The realization of the meta-analysis was not considered due to the significant heterogeneity between study designs and interventions.
Risk of bias assessment
The SYRCLE RoB tool was used to assess the risk of bias (RoB) of experimental animal studies. The tool contains 10 entries related to six types of bias: selection, performance, detection, attrition, reporting, and other bias ( Hooijmans et al., 2014 ). Two independent reviewers made the judgment for each study. The answers were “yes” (low RoB), “no” (high RoB), and “unclear” (insufficient details have been reported to assess the RoB).
Search for articles and data collection
Through the keyword search in PubMed and ScienceDirect databases, 21,039 records were identified. An additional three records were identified from the citation search. Duplicate studies, studies including different topics, studies that used another animal species, studies that used a combination of citrus or food were eliminated. Finally, a total of 14 studies fulfilled the eligibility criteria and were included in the review. Figure 1 depicts the PRISMA 2020 flow diagram of the selection process for the articles included in this systematic review.
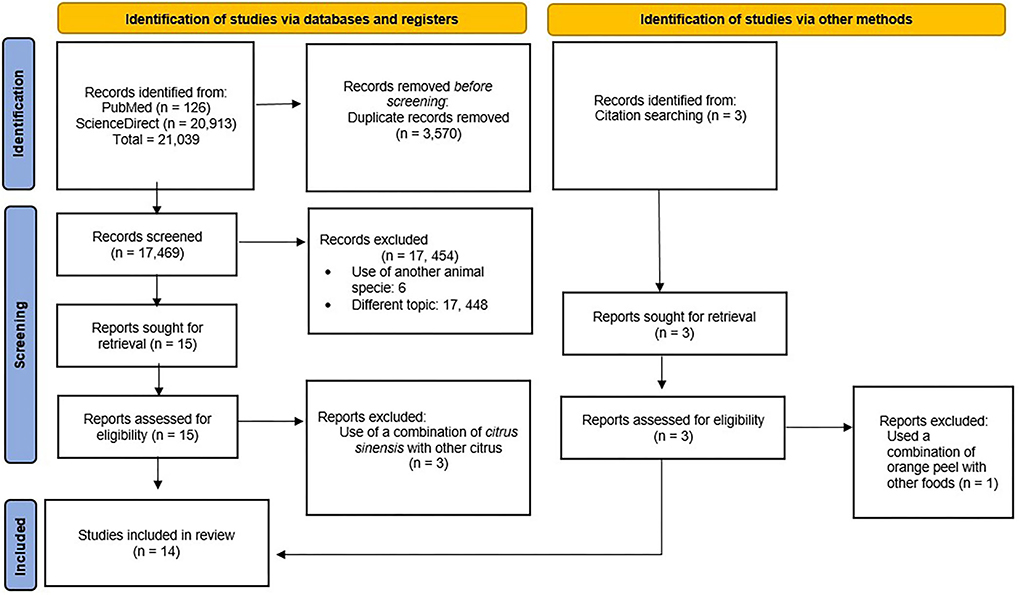
Figure 1 . PRISMA 2020 flow diagram of the selection process for the articles included in the systematic review. From Page et al. (2021) . For more information, visit: http://www.prisma-statement.org/ .
In Figure 2 , overall and individual RoBs were assessed for all the included studies. For selection bias, >90% of the studies did not specify the random allocation of animals. For those studies, we assumed a non-random application because for this type of research, the researchers included the subjects based on their preference for sex or animal strain, the result of a laboratory test, or even the availability of animals in the laboratory. Baseline characteristics (>90%) were frequently reported because these features were mostly considered the starting point for assigning experimental groups. Allocation concealment was not considered in these investigations, which resulted in a high RoB. Performance bias considered random housing (>90%), which resulted in a low RoB. However, blinding of investigators and caregivers was completely unclear. For detection bias, the random outcome assessment and blinding of outcome assessment items were rated as unclear RoBs. For attrition and reporting bias, incomplete outcome data and selective outcome reporting were mostly judged as a low RoB. Reporting of other biases was unclear as animal studies often do not report them. Overall, for the 10 entries of the RoB tool, 50% was ranked as “unclear” due to the lack of information from the studies, almost 40% was evaluated as a low RoB, and 10% as a high RoB.
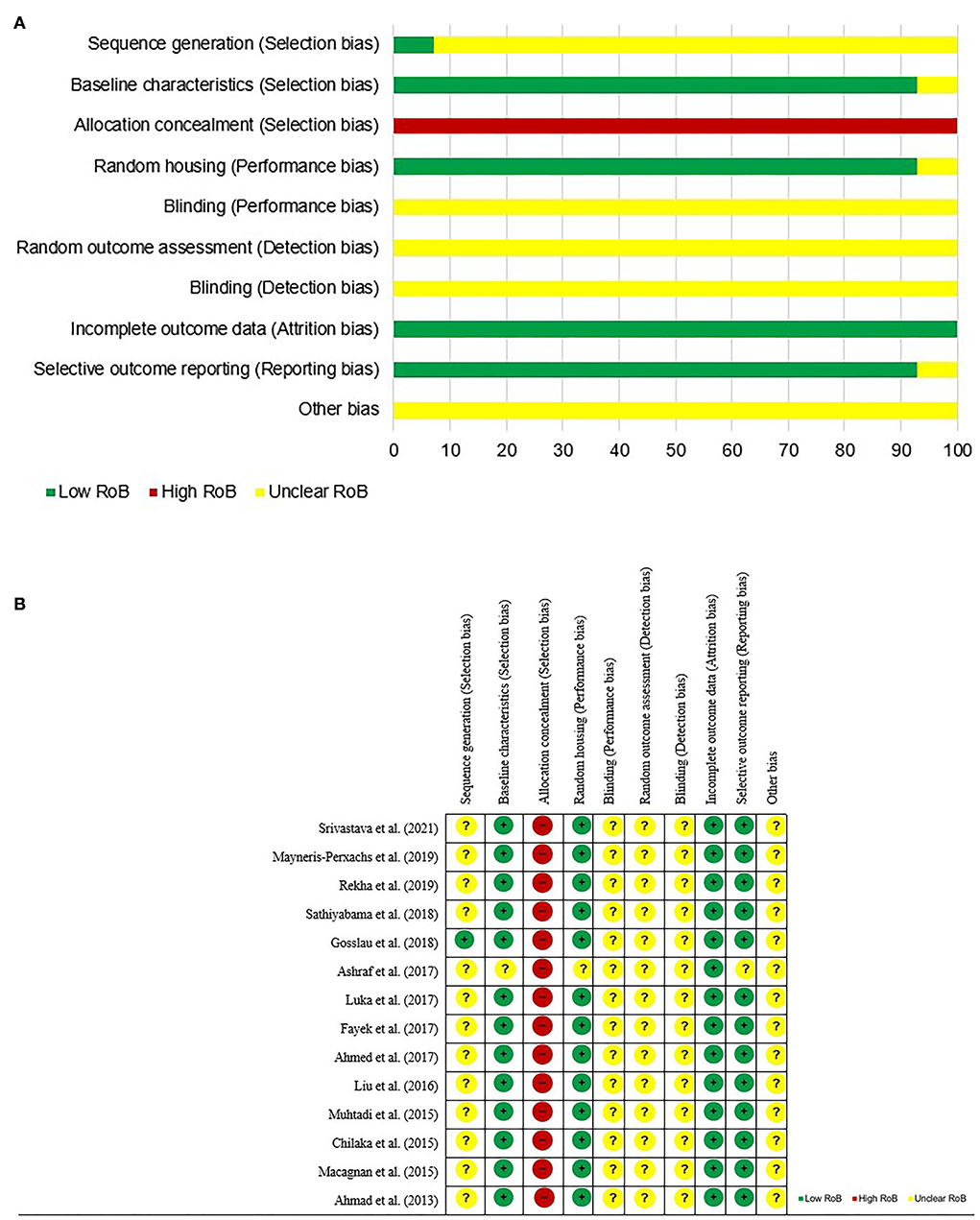
Figure 2 . SYRCLE's RoB tool to assess the Risk of Bias (RoB). (A) Percentage of overall RoB for all included studies. (B) Individual RoB for each item of the tool.
Characteristics of the studies
From the 14 articles, 28.6% of the articles were published in 2017, 21.42% in 2015, 14.28% between 2018 and 2019, and 7.14% in 2013, 2016, and 2021.
Table 1 shows the articles that used in vivo rodent models to evaluate the effect of by-products. The data only included the information of the experimental groups exposed to an orange by-product and their comparative results with the negative control group or the healthy control group, as appropriate. The rest of groups that served as controls or were not exposed to the by-product were not included in the review.
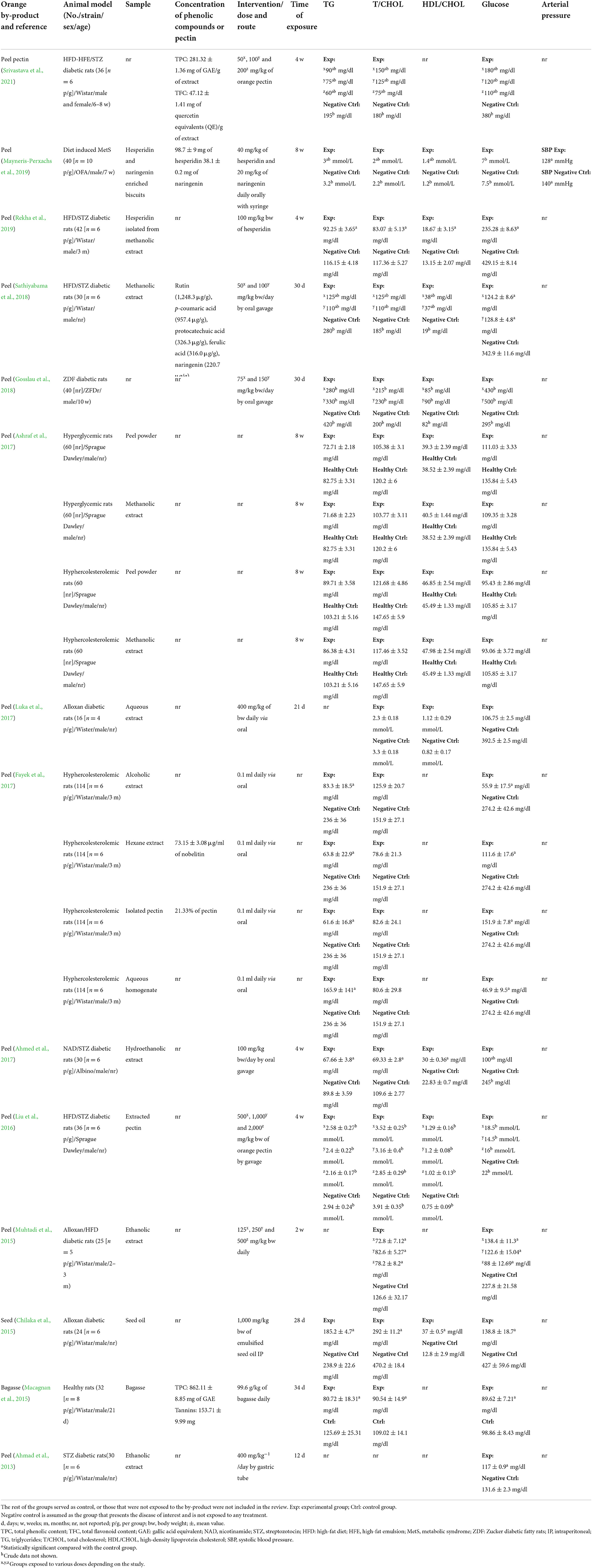
Table 1 . Characteristics of the in vivo rodent studies and the effect of orange ( Citrus sinensis ) by-products.
Characteristics of animal models
Most of the studies used a pathological model of chronic diseases. Overall, 35.7% of the studies used genetically and chemically induced diabetic rats ( Ahmad et al., 2013 ; Chilaka et al., 2015 ; Ahmed et al., 2017 ; Luka et al., 2017 ; Gosslau et al., 2018 ); another 35.7% of studies used rat models induced by ingestion of high-fat diets with chemical induction by streptozotocin or alloxan ( Muhtadi et al., 2015 ; Liu et al., 2016 ; Sathiyabama et al., 2018 ; Srivastava, 2018 ; Rekha et al., 2019 ); one study used rats with diet-induced metabolic syndrome ( Mayneris-Perxachs et al., 2019 ); two studies used hyperglycemic and hypercholesterolemic rodents ( Ashraf et al., 2017 ; Fayek et al., 2017 ); and one study evaluated the effect of orange bagasse in healthy rats ( Macagnan et al., 2015 ).
Experimental groups with 4–10 rats per group were integrated; 57% of the studies had groups composed of six animals. Wistar rats were used in most of the studies ( Ahmad et al., 2013 ; Chilaka et al., 2015 ; Macagnan et al., 2015 ; Muhtadi et al., 2015 ; Ahmed et al., 2017 ; Fayek et al., 2017 ; Luka et al., 2017 ; Sathiyabama et al., 2018 ; Srivastava, 2018 ; Rekha et al., 2019 ), followed by Sprague Dawley ( Liu et al., 2016 ; Ashraf et al., 2017 ), OFA ( Mayneris-Perxachs et al., 2019 ), and Zucker diabetic fatty rats ( Gosslau et al., 2018 ). Male rodents were used in 92.85% of the studies, and one study used rats of both sexes ( Srivastava, 2018 ). The age of the rats ranged between 3 and 12 weeks ( Macagnan et al., 2015 ; Muhtadi et al., 2015 ; Fayek et al., 2017 ; Gosslau et al., 2018 ; Srivastava, 2018 ; Mayneris-Perxachs et al., 2019 ; Rekha et al., 2019 ), whereas 50% of studies did not report the age of experimental animals.
Orange by-product intervention
Peel was the most used by-product ( Ahmad et al., 2013 ; Muhtadi et al., 2015 ; Liu et al., 2016 ; Ahmed et al., 2017 ; Ashraf et al., 2017 ; Fayek et al., 2017 ; Luka et al., 2017 ; Gosslau et al., 2018 ; Sathiyabama et al., 2018 ; Srivastava, 2018 ; Mayneris-Perxachs et al., 2019 ; Rekha et al., 2019 ), and some studies also used seed ( Chilaka et al., 2015 ) and bagasse ( Macagnan et al., 2015 ). In most research studies, an extract from the by-product was used, including methanolic, alcoholic, hexane, hydroethanolic, and aqueous extracts, whereas in other studies, a part of the by-product was obtained, for example, pectin from orange peel.
Some studies determined and quantified phenolic compounds and pectin from the by-products ( Macagnan et al., 2015 ; Fayek et al., 2017 ; Sathiyabama et al., 2018 ; Srivastava, 2018 ; Mayneris-Perxachs et al., 2019 ). The preferred route of administration was orally by gavage or syringe, whereas seed oil was administered intraperitoneally ( Chilaka et al., 2015 ). Various doses were evaluated on the basis of milligrams per kilogram body weight. The effect of low, medium, and high doses was compared ( Gosslau et al., 2018 ; Sathiyabama et al., 2018 ; Srivastava, 2018 ). The time of exposure varied between 12 days and 8 weeks; exposure for 4 weeks was the most frequently used ( Chilaka et al., 2015 ; Liu et al., 2016 ; Ahmed et al., 2017 ; Srivastava, 2018 ; Rekha et al., 2019 ).
Effect on metabolic biomarkers
Overall, 78.6% of the articles reported levels of triglycerides, total cholesterol, and glucose levels, while arterial pressure was reported in one article ( Mayneris-Perxachs et al., 2019 ). Regardless of the control group with which it was compared, the effect of orange by-products was always reported to be lower in the group exposed to orange.
Orange represents 58% of global citrus production. After its industrial processing, around a half of its weight is discarded as waste, contributing to environmental pollution ( Pacheco et al., 2019 ). This represents a great challenge for agroindustry; therefore, current research efforts focus on considering these wastes as potential products to obtain cost-efficient and promising nutraceuticals.
Derived from these arguments, this research focused on collecting scientific evidence of the assessment of the potential biological effect reported by the studies on orange by-products through a systematic review.
The results indicated that studies on orange residues are scarce. This review collected only 14 studies that met the eligibility criteria. Another aspect to consider is that this research was interested in experiments conducted in rodents as models as rats are the most widely used animal model in medical research since their pathophysiology is close to that of humans as well as since their genome is similar to the human genome ( Iannaccone and Jacob, 2009 ; Blais et al., 2017 ). Overall, 92.8% of studies included in this review used models that mimic conditions of human chronic diseases.
Undoubtedly, animal experimentation provides various advantages; however, transferring the results to clinical practice in humans has been widely debated. Systematic reviews help collect the information needed for making evidenced-based decisions. Tools such as SYRCLE RoB allows for assessing the methodological quality of studies. When we applied this tool for the review, we noticed that the lack of information in the studies made the assessment of their quality unclear and that randomization bias represented a high RoB for the results, which prohibits most of animal studies from translating their results to human trials ( van der Worp et al., 2010 ).
In addition, it is important to highlight that half of the studies reviewed did not indicate the age of their experimental subjects. Data on the age of the animal is essential since the attempt to transfer the results to human beings requires identifying the stage of animal life in which the results were obtained and it has also been shown that the age of the animal plays an important role in the modeling of human diseases ( Ghasemi et al., 2021 ). It has been calculated that in adulthood, 1 day in the life of the rat is equivalent to 34.8 human days ( Sengupta, 2013 ).
On the other hand, the bio-functionality of orange by-products requires evidence of its biosafety. In relation to this, the toxicity of Citrus sinensis has been evaluated. Li et al. determined the safety of hesperidin isolated from the methanolic extract of dried peel. They concluded that hesperidin is lethal at a median dose of 4,837.5 mg/kg and showed a low adverse effect at 1,000 mg/kg in the studied rats ( Li et al., 2019 ), while Unanma et al. showed using the test of acute toxicity of the citrus methanol peel extract that a dose of 5,000 mg/kg was tolerable ( Unanma et al., 2021 ).
The peel was extensively reported in the studies probably because this derivative is the major by-product, representing 50%−65% of the total weight of the citrus ( Pacheco et al., 2019 ; Ricci et al., 2019 ). The citrus fruit peel contains more antioxidant compounds than pulp and seeds ( Zayed et al., 2021 ). Also, the peel has been used to extract essential oils, carotenoids, pectin, and flavonoids. Some of the compounds that have been identified in orange peel are nobiletin, aglycone quercetin, diosmin, naringenin, rutin, hesperidin, naringin, among others, with naringin and hesperidin being the two major flavonoid glycosides present in citrus ( El-Kersh et al., 2021 ; Saini et al., 2022 ).
The fiber and phenolic compounds present in the fruit contribute to biological effects obtained from orange by-products. Anti-diabetic effects have been associated with flavonoids, which are widely found in citrus peels ( Ahmed et al., 2017 ). Soluble fiber and pectin extracted from orange peel have been associated with glucose improvement and lower plasma lipid levels ( Srivastava et al., 2021 ). Ahmed et al. (2017) demonstrated the anti-hyperglycemic, anti-hyperlipidemic, and antioxidant effects in diabetic rats due to the content of naringin and naringenin present in the orange peel extract. Some of the mechanisms of action are the improvement in excretion and tissue sensitivity of insulin, which could be due to improved expression of insulin, adiponectin, and GLUT4 receptors ( Ahmed et al., 2017 ). The same compounds extracted from lemon peel exerted an inhibitory effect on lipase, α-amylase, and α-glucosidase ( Gavahian et al., 2022 ), while hesperidin showed a protective effect against oxidative stress in embryos of diabetic pregnant rats and an inhibitory effect in the Cu 2+ -induced oxidation of low-density lipoprotein in an in vitro study. Naringin also demonstrated an antioxidant effect and led to an increase in the effect of enzymes like catalase and superoxide dismutase in case of tissue injuries ( Zhou et al., 2015 ). Sweet orange peel extract has been shown to lower the lipid profile by inhibiting the activity of the NPC1L1 gene in the intestinal wall, which can be attributed to the flavonoid content, especially hesperidin ( Samsudin et al., 2017 ). Similarly, Sathiyabama et al. (2018) reported an antilipidemic effect of orange peel extract and also a protective effect on the pancreatic β-cell structure and function ( Sathiyabama et al., 2018 ). Seeds of fruits are an important source of compounds such as tocopherols (α-tocopherol), phytosterols (β-sitosterol, campesterol, and stigmasterol), and carotenoids (α and β carotenes). The antioxidant activity of oils derived from different varieties of orange seeds was correlated with the α-tocopherol content ( Jorge et al., 2016 ).
Overall, total phenolic compounds, total flavonoid content, and compounds hesperidin, eriocitrin, narirutin, didymin, tangeretin, sinensetin, nobiletin, limonene, myrcene, β-pinene, β-ocimene, and sabinene from Citrus sinensis juice, pulp, seed, peel, and leaves have been associated with antioxidant activity, inhibition of peroxidation, and inhibition of α-amylase and β-glucosidase ( Leporini et al., 2021 ). These properties have advantage in the treatment of non-communicable diseases.
Of the studies reviewed, positive effects were observed in the physiological parameters evaluated since the experimental groups exposed to the orange by-product presented a reduction in the levels of triglycerides, total cholesterol, glucose, and systolic blood pressure, while HDL cholesterol increased (see Table 1 ). These findings were observed both in the pathological models of rats and in the healthy experimental groups, so we suggest orange by-products could be considered not only for the treatment of chronic diseases but also as a preventive approach both in healthy people and those with risk factors.
The proposed mechanisms of actions related to the biological effects of orange are diverse. The by-products have demonstrated to improve glucose levels via insulinotropic effects by enhancing the GLUT4 insulin receptor and adiponectin expression in adipose tissue ( Ahmed et al., 2017 ), reduce the formation of advanced glycation end products (AGEs) via their capacity to inhibit the glycation activity ( Deve et al., 2014 ), and delay the absorption and digestion of carbohydrates by soluble fibers that increase the viscosity of the food and disturb the diffusion of glucose, and, on the other hand, demonstrated the possibility of the fiber to inhibit α-amylase enzyme activity ( Macagnan et al., 2015 ; Huang et al., 2019 ). Fruit fiber is related to the reduction of triglycerides through the secondary increase in insulin derived from absorption of glucose and the increase in lipoprotein lipase activity ( Macagnan et al., 2015 ). These positive effects are also attributed to the mediation of lipid and cholesterol homeostasis by altering liver lipid metabolites and even regulating genes (SREBP-1c, ACC, and FAS) related to lipid metabolism ( Feng et al., 2020 ).
It is important to mention that Citrus sinensis exerted similar results obtained by common drugs in the treatment of metabolic diseases ( Chilaka et al., 2015 ; Sathiyabama et al., 2018 ). The results obtained from experimental animals increase the knowledge of the disease and the mechanism of action of treatments, including those from natural sources. Nevertheless, the disparity between the results in animals and those in clinical trials remains controversial due to various aspects in both types of studies ( van der Worp et al., 2010 ). Therefore, to predict the results of clinical trials, these results should be tested in human beings in future.
Finally, the studies collected on the use of orange by-products, either through the recovery of bioactive compounds or through the incorporation of these elements in functional foods, strengthen the global sustainable food agenda. Researchers are currently working on incorporating new and more efficient innovative methods to use fruit waste in ecological and sustainable food chains. The purpose of recovering bioactive compounds from waste should follow processes such as extraction, isolation, and characterization of bioactive components. To properly harness its biological potential, appropriate steps must be taken to ensure that bioactive components are not destroyed, lost, or altered during the extraction process. Thus, in addition to studies of the health effects of these wastes, lines of biotechnological research should be strengthened to identify new “green” extraction techniques for the recovery of specific bioactive compounds and in order to improve its performance through time- and resource-efficient methods. In this context, an interdisciplinary study is appreciated for the use of resources, food issues, and their pathologies, as well as biosafety and consumption limits. It is important to consider new research directions for the use of citrus fruit residues since it not only impacts the problem of its disposal but also provides solutions to eliminate the resulting contamination. The final goal should be closer to a responsible use of fruit waste that leads to zero waste.
Although there are only few studies that have evaluated the functional properties of orange by-products, based on this systematic review of the biological effects of by-products, the peel was found to be the most used derivative, and positive effects on the lipid profile and glucose levels have been reported in the intervention with animal models. These properties have advantage in the treatment of non-communicable diseases. Despite the fact that the results have been favorable in terms of the functional effect of orange by-products, methodological quality assessed using the SYRCLE RoB revealed the lack of information from studies, which represents a high risk of bias for translational results. It would be important for researchers to note this tool when planning animal interventions.

Data availability statement
The original contributions presented in the study are included in the article/supplementary material, further inquiries can be directed to the corresponding author.
Author contributions
JP-L, VF-B, AM-M, and CV-C: conceptualization, investigation, writing—original draft, writing—review and editing, and project administration. JP-L and CV-C: methodology and visualization. VF-B and CV-C: formal analysis. JP-L and AM-M: resources. VF-B and AM-M: supervision. All authors have read and agreed to the published version of the manuscript.
Acknowledgments
The authors thank to the Departamento de Promoción, Preservación y Desarrollo de la Salud, Departamento de Ciencias Exactas y Metodologías and Departamento de Ciencias Sociales of the Centro Universitario del Sur de la Universidad de Guadalajara, Mexico.
Conflict of interest
The authors declare that the research was conducted in the absence of any commercial or financial relationships that could be construed as a potential conflict of interest.
Publisher's note
All claims expressed in this article are solely those of the authors and do not necessarily represent those of their affiliated organizations, or those of the publisher, the editors and the reviewers. Any product that may be evaluated in this article, or claim that may be made by its manufacturer, is not guaranteed or endorsed by the publisher.
Adubofuor, J., Akyereko, Y. G., Batsa, V., Apeku, O.-J. D., Amoah, I., Diako, C., et al. (2021). nutrient composition and physical properties of two orange seed varieties. Int. J. Food Sci. 2021, 1–11. doi: 10.1155/2021/6415620
PubMed Abstract | CrossRef Full Text | Google Scholar
Afrin, S. M., Acharjee, A., and Sit, N. (2022). Convective drying of orange pomace at different temperatures and characterization of the obtained powders. J. Food Sci. Technol. 59, 1040–1052. doi: 10.1007/s13197-021-05108-2
Ahmad, M., Ansari, M. N., Alam, A., and Khan, T. H. (2013). Oral dose of citrus peel extracts promotes wound repair in diabetic rats. Pak. J. Biol. Sci. 16, 1086–1094. doi: 10.3923/pjbs.2013.1086.1094
Ahmed, O. M., Hassan, M. A., Abdel-Twab, S. M., and Abdel Azeem, M. N. (2017). Navel orange peel hydroethanolic extract, naringin and naringenin have anti-diabetic potentials in type 2 diabetic rats. Biomed. Pharmacother. 94, 197–205. doi: 10.1016/j.biopha.2017.07.094
Ashraf, H., Butt, M. S., Iqbal, M. J., and Suleria, H. A. R. (2017). Citrus peel extract and powder attenuate hypercholesterolemia and hyperglycemia using rodent experimental modeling. Asian Pac. J. Trop. Biomed. 7, 870–880. doi: 10.1016/j.apjtb.2017.09.012
CrossRef Full Text | Google Scholar
Ayala, J. R., Montero, G., Coronado, M. A., García, C., Curiel-Alvarez, M. A., León, J. A., et al. (2021). Characterization of orange peel waste and valorization to obtain reducing sugars. Molecules 26, 1348. doi: 10.3390/molecules26051348
Blais, E. M., Rawls, K. D., Dougherty, B. V., Li, Z. I., Kolling, G. L., Ye, P., et al. (2017). Reconciled rat and human metabolic networks for comparative toxicogenomics and biomarker predictions. Nat. Commun. 8, 14250. doi: 10.1038/ncomms14250
Chilaka, K. C., Ifediba, E. C., and Ogamba, J. O. (2015). Evaluation of the effects of Citrus sinensis seed oil on blood glucose, lipid profile and liver enzymes in rats injected with alloxan monohydrate. J. Acute Dis. 4, 129–134. doi: 10.1016/S2221-6189(15)30022-6
de Castro, L. A., Lizi, J. M., das Chagas, E. G. L., de Carvalho, R. A., and Vanin, F. M. (2020). From orange juice by-product in the food industry to a functional ingredient: application in the circular economy. Foods 9, 593. doi: 10.3390/foods9050593
de Moraes Crizel, T., Jablonski, A., de Oliveira Rios, A., Rech, R., and Flôres, S. H. (2013). Dietary fiber from orange byproducts as a potential fat replacer. LWT - Food Sci. Technol . 53, 9–14. doi: 10.1016/j.lwt.2013.02.002
Deve, A. S., Kumar, T. S., Kumaresan, K., and Rapheal, V. S. (2014). Extraction process optimization of polyphenols from Indian Citrus sinensis - as novel antiglycative agents in the management of diabetes mellitus. J. Diabetes Metab. Disord . 13. doi: 10.1186/2251-6581-13-11
El-Kersh, D. M., Ezzat, S. M., Salama, M. M., Mahrous, E. A., Attia, Y. M., Ahmed, M. S., et al. (2021). Anti-estrogenic and anti-aromatase activities of citrus peels major compounds in breast cancer. Sci. Rep. 11, 7121. doi: 10.1038/s41598-021-86599-z
El-safy, S., Salem, R., and Abd El-Ghany, M. (2012). Chemical and nutritional evaluation of different seed flours as novel sources of protein. World J. Dairy Food. Sci . 7, 59–65. doi: 10.5829/idosi.wjdfs.2012.7.1.61215
Ezejiofor, T. L. N., Eke, N. V., Okechukwu, R. I., Nwoguikpe, R. N., and Duru, C. M. (2011). Waste to wealth: industrial raw materials potential of peels of Nigerian sweet orange ( Citrus sinensis ). Afr. J. Biotechnol. 10, 6257–6264. doi: 10.5897/AJB10.1931
Fayek, N. M., El-Shazly, A. H., Abdel-Monem, A. R., Moussa, M. Y., Abd-Elwahab, S. M., El-Tanbouly, N. D., et al. (2017). Comparative study of the hypocholesterolemic, antidiabetic effects of four agro-waste citrus peels cultivars and their HPLC standardization. Rev. Bras. Farmacogn. 27, 488–494. doi: 10.1016/j.bjp.2017.01.010
Feng, K., Zhu, X., Liu, G., Kan, Q., Chen, T., Chen, Y., et al. (2020). Dietary citrus peel essential oil ameliorates hypercholesterolemia and hepatic steatosis by modulating lipid and cholesterol homeostasis. Food Funct. 11, 7217–7230. doi: 10.1039/D0FO00810A
Fernández-López, J., Sendra-Nadal, E., Navarro, C., Sayas, E., Viuda-Martos, M., Alvarez, J. A. P., et al. (2009). Storage stability of a high dietary fibre powder from orange by-products. Int. J. Food Sci. Technol. 44, 748–756. doi: 10.1111/j.1365-2621.2008.01892.x
Food Agriculture Organization of the United Nations. (2022). Citrus. Markets and Trade . Available online at: https://www.fao.org/markets-and-trade/commodities/citrus/en/ (accessed June 6, 2022).
Google Scholar
Garcia-Castello, E. M., Rodriguez-Lopez, A. D., Conidi, C., and Cassano, A. (2022). “Valorization of citrus by-products by membrane processes,” in Membrane Engineering in the Circular Economy , eds A. Lulianelli, C. Conidi, A. Cassano, and K. Petrotos (Amsterdam: Elsevier), 413–436. doi: 10.1016/B978-0-323-85253-1.00009-5
Gavahian, M., Yang, Y.-H., and Tsai, P.-J. (2022). Power ultrasound for valorization of Citrus limon (cv. Eureka) waste: effects of maturity stage and drying method on bioactive compounds, antioxidant, and anti-diabetic activity. Innov. Food Sci. Emerg. Technol. 79, 103052. doi: 10.1016/j.ifset.2022.103052
Ghasemi, A., Jeddi, S., and Kashfi, K. (2021). The laboratory rat: age and body weight matter. EXCLI J. 20, Doc1431; ISSN 1611–2156. doi: 10.17179/excli2021-4072
Gosslau, A., Zachariah, E., Li, S., and Ho, C. T. (2018). Effects of a flavonoid-enriched orange peel extract against type 2 diabetes in the obese ZDF rat model. Food Sci. Hum. Wellness 7, 244–251. doi: 10.1016/j.fshw.2018.10.001
Hooijmans, C. R., Rovers, M. M., de Vries, R. B., Leenaars, M., Ritskes-Hoitinga, M., Langendam, M. W., et al. (2014). SYRCLE's risk of bias tool for animal studies. BMC Med. Res. Methodol. 14, 43. doi: 10.1186/1471-2288-14-43
Huang, Y. L., Ma, Y. S., Tsai, Y. H., and Chang, S. K. C. (2019). In vitro hypoglycemic, cholesterol-lowering and fermentation capacities of fiber-rich orange pomace as affected by extrusion. Int. J. Biol. Macromol. 124, 796–801. doi: 10.1016/j.ijbiomac.2018.11.249
Iannaccone, P. M., and Jacob, H. J. (2009). Rats! Dis. Model. Mech. 2, 206–210. doi: 10.1242/dmm.002733
Jorge, N., da Silva, A. C., and Aranha, C. P. M. (2016). Antioxidant activity of oils extracted from orange ( Citrus sinensis ) seeds. An. Acad. Bras. Ciênc . 88, 951–958. doi: 10.1590/0001-3765201620140562
Leporini, M., Tundis, R., Sicari, V., and Loizzo, M. R. (2021). Citrus species: modern functional food and nutraceutical-based product ingredient. Ital. J. Food Sci. 33, 63–107. doi: 10.15586/ijfs.v33i2.2009
Li, Y., Kandhare, A. D., Mukherjee, A. A., and Bodhankar, S. L. (2019). Acute and sub-chronic oral toxicity studies of hesperidin isolated from orange peel extract in Sprague Dawley rats. Regul. Toxicol. Pharmacol. 105, 77–85. doi: 10.1016/j.yrtph.2019.04.001
Liu, Y., Dong, M., Yang, Z., and Pan, S. (2016). Anti-diabetic effect of citrus pectin in diabetic rats and potential mechanism via PI3K/Akt signaling pathway. Int. J. Biol. Macromol. 89, 484–488. doi: 10.1016/j.ijbiomac.2016.05.015
Luka, C., Istifanus, G., George, M., and Philip, C. (2017). The effect of aqueous extract of Citrus sinensis peel on some biochemical parameters in normal and alloxan-induced diabetic wister rats. Am. J. Phytomed. Clin. Ther. 5, 17. doi: 10.21767/2321-2748.100330
Macagnan, F. T., Santos, L. R. D., Roberto, B. S., Moura, F. A. D., Bizzani, M., Silva, L. P. D., et al. (2015). Biological properties of apple pomace, orange bagasse and passion fruit peel as alternative sources of dietary fibre. Bioact. Carbohydr. Diet. Fibre 6, 1–6. doi: 10.1016/j.bcdf.2015.04.001
Malacrida, C. R., Kimura, M., and Jorge, N. (2012). Phytochemicals and antioxidant activity of citrus seed oils. Food Sci. Technol. Res. 18, 399–404. doi: 10.3136/fstr.18.399
Mayneris-Perxachs, J., Alcaide-Hidalgo, J. M., de la Hera, E., del Bas, J. M., Arola, L., and Caimari, A. (2019). Supplementation with biscuits enriched with hesperidin and naringenin is associated with an improvement of the metabolic syndrome induced by a cafeteria diet in rats. J. Funct. Foods 61, 103504. doi: 10.1016/j.jff.2019.103504
Muhtadi, M., Haryoto, H., Azizah, T., Suhendi, A., and Yen, K. (2015). Antidiabetic and antihypercholesterolemic activities of Citrus sinensis peel: in vivo study. Natl. J. Physiol. Pharm. Pharmacol . 5, 382. doi: 10.5455/njppp.2015.5.2807201561
Okoye, C. O. B., Ibeto, C. N., and Ihedioha, J. N. (2011). Preliminary studies on the characterization of orange seed and pawpaw seed oils. Am. J. Food Technol . 6, 422–426. doi: 10.3923/ajft.2011.422.426
Olabinjo, O. O., Ogunlowo, A. S., Ajayi, O. O., and Olalusi, A. P. (2017). Analysis of physical and chemical composition of sweet orange ( Citrus sinensis ) peels. Int. J. Environ. Agric. Biotechnol. 2, 2201–2206. doi: 10.22161/ijeab/2.4.80
Pacheco, M. T., Moreno, F. J., and Villamiel, M. (2019). Chemical and physicochemical characterization of orange by-products derived from industry. J. Sci. Food Agric. 99, 868–876. doi: 10.1002/jsfa.9257
Page, M. J., McKenzie, J. E., Bossuyt, P. M., Boutron, I., Hoffmann, T. C., Mulrow, C. D., et al. (2021). The PRISMA 2020 statement: an updated guideline for reporting systematic reviews. BMJ . 372, n71. doi: 10.1136/bmj.n71
Pan American Health Organization. (2022). Noncommunicable Diseases. Noncommunicable Diseases . Avaialbe online at: https://www.paho.org/en/topics/noncommunicable-diseases (accessed June 07, 2022).
Parmar, H. S., and Kar, A. (2008). Antiperoxidative, antithyroidal, antihyperglycemic and cardioprotective role of Citrus sinensis peel extract in male mice. Phytother. Res. 22, 791–795. doi: 10.1002/ptr.2367
Plasek, B., Lakner, Z., Kasza, G., and Temesi, Á. (2019). Consumer evaluation of the role of functional food products in disease prevention and the characteristics of target groups. Nutrients 12, 69. doi: 10.3390/nu12010069
Rekha, S. S., Pradeepkiran, J. A., and Bhaskar, M. (2019). Bioflavonoid hesperidin possesses the anti-hyperglycemic and hypolipidemic property in STZ induced diabetic myocardial infarction (DMI) in male Wister rats. J. Nutr. Intermed. Metab. 15, 58–64. doi: 10.1016/j.jnim.2018.12.004
Ricci, A., Diaz, A. B., Caro, I., Bernini, V., Galaverna, G., Lazzi, C., et al. (2019). Orange peels: from by-product to resource through lactic acid fermentation. J. Sci. Food Agric. 99, 6761–6767. doi: 10.1002/jsfa.9958
Saini, R. K., Ranjit, A., Sharma, K., Prasad, P., Shang, X., Gowda, K. G. M., et al. (2022). Bioactive compounds of citrus fruits: a review of composition and health benefits of carotenoids, flavonoids, limonoids, and terpenes. Antioxidants 11, 239. doi: 10.3390/antiox11020239
Samsudin, R. R., Kunsah, B., and Widyastuti, R. (2017). The effect of pacitan's sweet orange's ( Citrus sinensis (L.) osbeck) peel powder on the lipid profile of male dyslipidemia rats ( Rattus novergicus ). Bali. Med. J . 6, 51. doi: 10.15562/bmj.v6i3.720
Sathiyabama, R. G., Gandhi, G. R., Denadai, M., Sridharan, G., Jothi, G., Sasikumar, P., et al. (2018). Evidence of insulin-dependent signalling mechanisms produced by Citrus sinensis (L.) Osbeck fruit peel in an insulin resistant diabetic animal model. Food Chem. Toxicol. 116, 86–99. doi: 10.1016/j.fct.2018.03.050
Sengupta, P. (2013). The laboratory rat: relating its age with human's. Int. J. Prev. Med. 4, 624–630.
PubMed Abstract | Google Scholar
Srivastava, R., Tripathi, L., Swain, S. R., and Singh, J. (2021). Neuroprotective validation of pectin in T2DM-induced allodynia and hyperalgesia in diabetic peripheral neuropathic pain. Arch. Physiol. Biochem . 23, 1–12. doi: 10.1080/13813455.2021.1884725
Srivastava, R. K. (2018). Need of nutraceuticals/functional food products for health benefits to world-wide people. J. Biotechnol. Biomed. Sci. 1, 1–13. doi: 10.14302/issn.2576-6694.jbbs-18-2408
U.S. Department of Agriculture. (2019). Oranges, raw, navels . Available online at: https://fdc.nal.usda.gov/fdc-app.html#/food-details/746771/nutrients (accessed April 14, 2022).
Unanma, H. C., Anaduaka, E. G., Uchendu, N. O., Ononiwu, C. P., and Ogugua, V. N. (2021). Ananas comosus and Citrus sinensis peels ameliorate CCl4-induced liver injury in Wistar rats. Sci Afr. 14:e01026. doi: 10.1016/j.sciaf.2021.e01026
United States Department of Agriculture. (2022). Citrus: World Markets and Trade . Available online at: https://usda.library.cornell.edu/concern/publications/w66343603?locale=en (accessed June 7, 2022).
van der Worp, H. B., Howells, D. W., Sena, E. S., Porritt, M. J., Rewell, S., O'Collins, V., et al. (2010). Can animal models of disease reliably inform human studies? PLoS Med. 7, e1000245. doi: 10.1371/journal.pmed.1000245
World Health Organization. (2022). Noncommunicable diseases: progress monitor 2022. Geneva: World Health Organization.Available online at: https://apps.who.int/iris/handle/10665/353048 (accessed September 4, 2022).
Zayed, A., Badawy, M. T., and Farag, M. A. (2021). Valorization and extraction optimization of citrus seeds for food and functional food applications. Food Chem. 355, 129609. doi: 10.1016/j.foodchem.2021.129609
Zayed, E. A., AinShoka, A. A., El Shazly, K. A., and Abd El Latif, H. A. (2018). Improvement of insulin resistance via increase of GLUT4 and PPARγ in metabolic syndrome-induced rats treated with omega-3 fatty acid or L-carnitine. J. Biochem. Mol. Toxicol. 32, e22218. doi: 10.1002/jbt.22218
Zhou, L., Pan, Y., Chonan, R., Batey, R., Rong, X., Yamahara, J., et al. (2015). Mitigation of insulin resistance by mangiferin in a rat model of fructose-induced metabolic syndrome is associated with modulation of CD36 redistribution in the skeletal muscle. J. Pharmacol. Exp. Ther. 356, 74–84. doi: 10.1124/jpet.115.229005
Keywords: Citrus sinensis , agri-food by-product, agri-food waste, phytochemicals, health effects, triglycerides, cholesterol, glucose
Citation: Pineda-Lozano JE, Fonseca-Bustos V, Martinez-Moreno AG and Virgen-Carrillo CA (2022) The biological effect of orange ( Citrus sinensis L.) by-products on metabolic biomarkers: A systematic review. Front. Sustain. Food Syst. 6:1003144. doi: 10.3389/fsufs.2022.1003144
Received: 25 July 2022; Accepted: 15 September 2022; Published: 02 November 2022.
Reviewed by:
Copyright © 2022 Pineda-Lozano, Fonseca-Bustos, Martinez-Moreno and Virgen-Carrillo. This is an open-access article distributed under the terms of the Creative Commons Attribution License (CC BY) . The use, distribution or reproduction in other forums is permitted, provided the original author(s) and the copyright owner(s) are credited and that the original publication in this journal is cited, in accordance with accepted academic practice. No use, distribution or reproduction is permitted which does not comply with these terms.
*Correspondence: Carmen Alejandrina Virgen-Carrillo, carmen.virgen@academicos.udg.mx
Disclaimer: All claims expressed in this article are solely those of the authors and do not necessarily represent those of their affiliated organizations, or those of the publisher, the editors and the reviewers. Any product that may be evaluated in this article or claim that may be made by its manufacturer is not guaranteed or endorsed by the publisher.
- Angiosperms
Citrus peel essential oils: a review on composition and antimicrobial activities
- January 2017
- 9(5):975-8712

- Kano University of Science & Technology

- Bayero University, Kano
Discover the world's research
- 25+ million members
- 160+ million publication pages
- 2.3+ billion citations

- Ibtisam Abalmawla
- Asma M Etbika
- Refaa Almousa
- J FOOD QUALITY

- Sahar Sarfraz

- Madras Agricultural Journal

- Saad Mohammed Hussein Ayoub

- Sara Kavosi

- Aayushi Kadam

- Lahsen El Ghadraoui

- Amira A Mohamed

- Sarah J Salih
- Jorge Luis Amorim

- Mariana Martins Gomes Pinheiro

- CHEM CENT J

- Yuanyan Liu

- R.M. Horowitz

- J STORED PROD RES
- Kamilla de Andrade Dutra

- Daniela Maria do Amaral Ferraz Navarro
- José Peroba Oliveira Santos
- Mohammad-Taghi Golmakani

- Recruit researchers
- Join for free
- Login Email Tip: Most researchers use their institutional email address as their ResearchGate login Password Forgot password? Keep me logged in Log in or Continue with Google Welcome back! Please log in. Email · Hint Tip: Most researchers use their institutional email address as their ResearchGate login Password Forgot password? Keep me logged in Log in or Continue with Google No account? Sign up

An official website of the United States government
The .gov means it’s official. Federal government websites often end in .gov or .mil. Before sharing sensitive information, make sure you’re on a federal government site.
The site is secure. The https:// ensures that you are connecting to the official website and that any information you provide is encrypted and transmitted securely.
- Publications
- Account settings
The PMC website is updating on October 15, 2024. Learn More or Try it out now .
- Advanced Search
- Journal List
- Evid Based Complement Alternat Med
- v.2021; 2021

This article has been retracted.
Citrus genus and its waste utilization: a review on health-promoting activities and industrial application, usman mir khan.
1 National Institute of Food Science and Technology, University of Agriculture, Faisalabad 38000, Pakistan
Aysha Sameen
Rana muhammad aadil, muhammad shahid.
2 Department of Biochemistry, Faculty of Sciences, University of Agriculture, Faisalabad 38000, Pakistan
Serap Sezen
3 Faculty of Engineering and Natural Science, Sabanci University, Tuzla, Istanbul 34956, Turkey
4 Sabanci University Nanotechnology Research and Application Center (SUNUM), Tuzla, Istanbul 34956, Turkey
Ali Zarrabi
5 Department of Biomedical Engineering, Faculty of Engineering and Natural Sciences, Istinye University, Istanbul, Turkey
Betul Ozdemir
6 Department of Cardiology, Faculty of Medicine, Nigde Ömer Halisdemir University, Nigde, Turkey
Mustafa Sevindik
7 Bahçe Vocational High School, Osmaniye Korkut Ata University, Osmaniye 80500, Turkey
Dilara Nur Kaplan
8 Department of Nutrition and Dietetics, Faculty of Health Sciences, Karabuk University, Karabuk 78050, Turkey
Zeliha Selamoglu
9 Department of Medical Biology, Faculty of Medicine, Nigde Ömer Halisdemir University, Nigde 51240, Turkey
Alibek Ydyrys
10 Biomedical Research Centre, Al-Farabi Kazakh National University, Al-Farabi Av. 71, Almaty 050040, Kazakhstan
11 Department of Postharvest Technology, Horticultural College and Research Institute, Periyakulam 625604, Tamil Nadu, India
Manoj Kumar
12 Chemical and Biochemical Processing Division, ICAR–Central Institute for Research on Cotton Technology, Mumbai 400019, India
Javad Sharifi-Rad
13 Phytochemistry Research Center, Shahid Beheshti University of Medical Sciences, Tehran, Iran
Monica Butnariu
14 Banat's University of Agricultural Sciences and Veterinary Medicine “King Michael I of Romania” from Timisoara, Timisoara, Romania
Associated Data
The data supporting this review are from previously reported studies and data sets, which have been cited. The processed data are available from the corresponding author upon request.
Citrus fruits such as oranges, grapefruits, lemons, limes, tangerines, and mandarins, whose production is increasing every year with the rise of consumer demand, are among the most popular fruits cultivated throughout the globe. Citrus genus belongs to the Rutaceae family and is known for its beneficial effects on health for centuries. These plant groups contain many beneficial nutrients and bioactive compounds. These compounds have antimicrobial, anticancer, antidiabetic, antiplatelet aggregation, and anti-inflammatory activities. Citrus waste, generated by citrus-processing industries in large amounts every year, has an important economic value due to richness of bioactive compounds. The present review paper has summarized the application and properties of Citrus and its waste in some fields such as food and drinks, traditional medicine practices, and recent advances in modern approaches towards pharmaceutical and nutraceutical formulations.
1. Introduction
Vegetables and fruits, which have been used as flavours, fragrances, dyes, preservatives, and pharmaceuticals for centuries, have an important place in human nutrition with their very important nutritional functions. Epidemiological studies have shown that fruit and vegetable consumption has significant beneficial effects on health, like reducing the risk of coronary heart disease, stroke, and some types of cancer. These positive effects on health were mainly attributed to dietary fibres and secondary metabolites. Also, carotenoids and flavonoids were basically thought to be effective because of their strong antioxidant properties. Compounds with the most important therapeutic effects among flavonoids are the flavanols like catechin, epicatechin, and procyanidin that are present in apples and grapes with heart-protecting effects, as well as flavanones, which are the dominant flavonoid class in Citrus fruits [ 1 ]. Essential minerals for humans are mainly provided by dietary fruits, vegetables, and juices. Especially, fruit and fruit products are important sources of minerals [ 2 ]. Citrus fruits are known as one of the most prevalent products in the world that are cultivated in more than 100 countries. The suitable regions for cultivation of these types of fruits are tropical and subtropical areas due to their soil and favourable climatic conditions. Orange (61%), mandarin (22%), lime and lemon (11%), and grapefruit (6%) of total are the most important citrus fruits. These fruits are commercially important in both fresh-consumption market and processing industry. It is estimated that the citrus-processing industry, which mainly have focused on fruit juice and essential oil production for a long time, use 33% of the citrus harvest for fruit juice production. Moreover, most parts of them are converted to waste. For instance, while about 70% of the manufactured orange are used for the production of derivative products, nearly 50–60% of them are converted to wastes (peels, seeds, and membrane residue) [ 3 ].
Citrus fruits such as lemons, grapefruits, limes, oranges, tangerines, and mandarins are the most cultivated fruits in the world, whose production is increasing every year with the rise of consumer demand. However, most parts of these fruits are wasted during their industrial processing, for instance about 50% of the wet mass of citrus is their peel waste. These large amounts of citrus waste are rich sources of contents with high economic value, like different types of flavonoids, dietary fibre, polyphenols, carotenoids, essential oils, sugars, ascorbic acid, and important levels of some trace elements. Another favourable feature is the high amount of sugar, which is suitable for the production of bioethanol via fermentation [ 4 ].
The most produced and consumed fruit of the citrus group is sweet orange ( Citrus sinensis ) with 70%. The other types of widely consumed citrus fruits are tangerine or mandarin ( Citrus reticulata ), lime ( Citrus aurantifolia ), grapefruit ( Citrus vitis ), and lemon ( Citrus limonum ). It is well known that citrus fruits and their products are rich in vitamins, minerals, and dietary fibres (nonstarch polysaccharides), which are important for human nutrition, growth, and development [ 4 ].
Citrus fruits are also famous due to their other active compounds such as flavonoids, vitamins, carotenoids, and minerals, which could eliminate the risk of various chronic diseases (e.g., cardiovascular diseases and age-related macular degeneration). These bioactive compounds have therapeutic effects such as antioxidant, anticancer, and antitumour properties. These compounds have antimicrobial, anticancer, antidiabetic, antiplatelet aggregation, and anti-inflammatory activities. As mentioned before, citrus fruits are mostly cultivated in tropical and subtropical regions with the annual production of about 88 million tons. As the increasing demand for low-fat carbohydrates, low-sodium minerals, dietary fibres, and vitamins (especially B complex and C), citrus fruits have become an integral part of the human diet. Most of the citrus fruits (about 80%) are used in the production of items like juice, jellies, jams, marmalades, and similar processed products that leads to the generation of about 40 million tons of waste [ 5 ].
Different citrus fruits have different characteristics in terms of type, variety, quality, and degree of maturity. Citrus wastes contain soluble sugar, starch, cellulose and hemicellulose fibres, ash, pectin, lignin, fat and protein, and some bioactive compounds, which could be used in different fields from pharmaceutical and nutraceutical to food, health drinks, and cosmetics. These are renewable resources of materials with economical values. Thus, the cost of the formulated products is reduced, and utilizing the synthetic agents is limited along with keeping the environment from pollution danger. In addition, these natural bioactive compounds, which are believed to significantly protect people from many diseases, are effectively used in therapeutic formulations [ 4 ].
This review compiles new aspects of Citrus genus that were not covered in previous literature works, such as historical existence of important Citrus genus along with its role as important health-promoting ingredients. The important biofunctionalities of the Citrus extracts and utilization of Citrus waste as biofuel, food, and pharmaceutical ingredients are also covered.
2. Historical Process of Genus Citrus
Orange, tangerine, and grapefruit plant groups, also known as Citrus plants, contain many beneficial nutrients [ 6 ]. The by-products of the plants are rich in phenolic content and dietary fibres due to the high amount of shells/peels produced by them. The Citrus wastes are among potential nutraceuticals that can be used as a rich and cheap source for nutritional supplements due to their easy extraction [ 7 ]. It is also one of the integral parts of the food and cosmetics industry. Plant parts of the members of the genus also used in the traditional healthcare system in the treatment of dermatitis, cough, muscle pain, nausea, vomiting, cancer, hypertension, and stomach pains using different descriptions [ 8 , 9 ]. A lot of research has been done due to the phytochemical contents of Citrus genus [ 10 ]. The phytochemical contents of the members of the genus have been researched, and there are ingredients such as coumarin, limonoid, flavonoid, terpenoid, and terpene.
The Citrus genus includes a wide diverse type of fruits that are high in volume and widely available in markets. Different types of fruit of the genus are grown due to their essential oils. Citrus species are known to originate mainly from southeast China and the Malaysian archipelago [ 11 ]. Different trade routes have led to the spread and cultivation of Citrus varieties to different climates of the world. In Japan, fruits such as yuzu, sikuwasya, hassaku, kabosu, mikan, iyokan, suchi, natsumikan, and ponkan are very popular. In the historical process, citron trees have been found in archaeological excavations where they were grown in Iran around 4000 BC [ 12 ]. In the following processes, Alexander the Great brought these fruits to the ancient Greek and Roman civilizations in 300 BC. It has been defined as the “fruit of Iran” or “the fruit of the media” in Greek and Roman literatures. This fruit, thought to have been taken by the Greeks to Philistines, is depicted on one side of a Jewish coin minted in 136 BC. This fruit was grown in different parts of the empire during the Roman Empire. In addition, during the Renaissance, the cultivation of Citrus trees expanded especially towards the north of the Mediterranean [ 13 – 16 ].
3. Phytochemical and Nutritional Profile of Citrus
Citrus fruits are ample sources of the antioxidant secondary metabolites belonging to the subclasses terpenoids and phenolics. Zhou reported as much as 170 antioxidant compounds from the Citrus fruit [ 17 ]. Carotenoids and limonoids are the main examples of the terpenoids, whereas flavonoids (naringenin, naringin, hesperidin, quercetin, and rutin), phenolic acids, and coumarins are the main examples of phenolic compounds present in Citrus fruits [ 18 ]. The chemical structure of the various bioactive compounds present in the citrus fruits is shown in Figure 1 . The flesh of Citrus paradisi Macf. Changshanhuyou constitutes a total phenolic content of 180 mg/g dry weight. The limonoid content of citrus fruits varied between 0 and 95.46 mg/100 g, whereas the carotenoid content was reported between 0.021 and 2.04, in case of peels of C . reticulata Blanco., C . grandis Osbeck, and C . sinensis Osbeck [ 18 ]. The extraction of these bioactive compounds from the citrus fruits and their by-products can be done using conventional extraction techniques (maceration, hydrodistillation, solvent extraction) and via green extraction approaches (enzyme-assisted extraction, ultrasound-assisted extraction, microwave-assisted extraction, pulse electric field extraction). Various extraction strategies have been recently reviewed; hence, we have limited this aspect in the current review [ 19 ].

Chemical structure of the important bioactive compounds from the Citrus fruit.
Among vitamins, vitamin A, B1, B2, B3, C, and E are found, which further improves the antioxidant profile of citrus fruits. The vitamin C content of Citrus unshiu Marc., Citrus maxima (Burm Merr.), C . sinensis Osbeck, and C . reticulata Blanco. varied between 30 and 60 mg/100 g, whereas the vitamin E content was found between 4.5 and 11.4 mg/kg [ 17 , 20 ]. Citrus also contains as much as 19 elements, such as Ca, Na, S, Mg, Ni, Fe, Cu, Zn, Mn, Mo, and Se [ 17 ]. Fe, Mn, and Zn are having the antioxidant role by associating as a cofactor with antioxidative enzymes and save the cells from oxidative damage. Se in C . sinensis Osbeck, C . reticulata Blanco., and C . limon Burm.f. is found in a range of 0.31–0.50 µ g/100 g [ 17 ].
The waste generated from horticultural crops in form of peels, leaves, seeds, and pomace are also rich sources of bioactive compounds with numerous reported health benefits [ 21 – 28 ]. The peel waste of Citrus contains an enormous quantity of the bioactive compounds that is even more (up to 5000 mg/g of phenolic content) than the edible portion of the fruit and having good health-promoting activities [ 29 ]. Apart from the bioactive components, citrus waste, more specifically peel, is good in minerals, vitamins, fatty acids, and free amino acids [ 29 ]. Citrus natsudaidai peel contains around 1.07 g/100 g of protein, 0.33–0.47 g/100 g of lipids, and 17.3–18.8 g/100 g of carbohydrates, whereas the pulp of C . natsudaidai showed the presence of 0.9, 0.1, and 10 g of proteins, lipids, and carbohydrates, respectively [ 29 ]. Extracts also showed the presence of free amino acids (121 mg/100 g) with the presence of eight essential amino acids such as Thr, Lys, Phe, His, Ile, Val, Met, and Leu. The authors also reported the total fatty acid content of 111 mg/100 g and showed the presence of both saturated and unsaturated fatty acids such as lauric acid, myristic acid, palmitic acid, palmitolic acid, heptadecanoic acid, stearic acid, oleic acid, and alpha-linolenic acid. Hence, the presence of phytochemicals and nutritional components make citrus fruits as one of the emerging nutraceuticals in the food industry.
4. Beneficial Role of Citrus Genus on the Health
Nutrition has significant effects on the development and protection of living organisms. Nontoxic phytochemicals for humans are important for human health due to their high antioxidant, antimutagenic, and anticancer activities. Many studies have shown that using foods with high value of flavonoids and low amounts of fatty acids, like fruits and vegetables, could lead to the reduction of the incidence of metabolic diseases in humans [ 30 ]. For example, Citrus extracts have been demonstrated to eliminate the risk of diseases such as cardiovascular diseases, diabetes, and cancer. They prevent oxidative stress, tissue damage, and inflammatory processes with the effects of antioxidants on the organism.
It has positive effects on human health depending on its contents such as vitamin C and flavonoids [ 31 – 33 ]. Flavonoids are the most common secondary plant metabolites found in a wide variety of edible fruits and vegetables [ 34 ]. More than sixty flavonoids have been identified, which are divided into three main groups: flavanones, flavones, and flavonols [ 35 ]. The other types of phenolic compounds found in Citrus species include phenolic acids, ferulic acids, and gallic acid. In general, Citrus fruits are rich in flavonoids that are an important part of the diet, especially their role in preventing diseases such as obesity, diabetes, lowering blood lipids, cardiovascular diseases, and different types of cancer [ 36 – 42 ]. Flavonoids with a wide range of biological activities have been used in medicine for preserving vascular integrity, antiosteoporotic agents, and antihepatotoxic properties. The anticancer activity of some of these flavonoids was confirmed via in vitro and in vivo tests. Some of them could also inhibit the activity of enzymes like xanthine oxidase and aldose reductase. The ability of these components in preventing the production of inflammatory mediators (like prostaglandins, leukotrienes, or nitric oxide) introduced them as ideal anti-inflammatory agents [ 43 ].
Flavanones are the most common Citrus flavonoids (e.g., 98% in grapefruit, 96% in lemon, and 90% in lemon) that show both antioxidant and anti-inflammatory properties [ 44 ]. Vitamin C, which is a water-soluble antioxidant with reactive oxygen species (ROS) scavenging property, can be found in two active forms in nature: ascorbic acid (1-AA) and dehydroascorbic acid (1-DHAA) [ 45 , 46 ]. The antioxidant function of this vitamin is from its hydrogen donor ability [ 47 – 49 ], and it inactivates free radicals. It is also involved in the synthesis of collagen, a connective tissue material [ 50 ]. Carotenoids are the other important components of Citrus species that have the ability of protection against photo-oxidation damage [ 51 ]. It has been reported that vitamin C and flavonoids have synergistic biological activities [ 52 ]. Finally, the health effects of flavonoids and vitamin C have direct relationship to their amount consumed and bioavailability.
Synthetic antioxidants can cause chronic toxicity. Therefore, natural antioxidants obtained from plants gain importance. Polyphenols are natural powerful nontoxic antioxidant agents that act as free-radical scavengers with no side effects [ 53 ]. Hesperidin and aglycone form have been shown to have a critical role in preventing the oxidative stress and inflammation-related diseases (cardiovascular disease, cancer). Their anti-inflammation ability is related to their antioxidant effects and their capability to inhibit the activity of different types of enzymes (such as cyclooxygenase, phosphodiesterase, lipoxygenase, protein kinase, and phospholipase), which are necessary for the activation and transduction of cellular signals. Various useful bioactivities imparted by the Citrus phytochemicals are discussed in following subsections.
4.1. Cardioprotective Activity
Cardiovascular disease is known as one of the leading causes of death in the world. Hesperidin with properties like antihypertensive, antioxidant, and anti-inflammatory activities, lipid-lowing, and improving the insulin-sensitivity has shown to have good cardiovascular protective capability. According to several epidemiological research works, there is a direct relationship between the dietary fibre consumption and reduction in the risk of different types of disorders, especially cardiovascular diseases [ 54 ]. On the other hand, soluble dietary fibre could eliminate the amount of cholesterol absorption via increasing the excretion of cholesterol and bile acids [ 55 ].
Grapefruit flavanones also have high effects on promoting the health quality, especially in the case of heart diseases. In other words, the fantastic impacts of grapefruit juice on preventing the coronary heart disease led to introducing a “healthy heart check” symbol by the American Heart Association for its different commercially available forms. Hesperidin, as a type of flavanone, has blood pressure-reducing effects. Hypotensive effects of hesperidin are associated with correction of endothelial dysfunction, increasing the bioavailability of NO, and also the expression of nicotinamide adenine dinucleotide phosphate (NADPH) oxidase [ 56 , 57 ]. In studies on rats with hesperidin, one of the Citrus flavonoids, it has been observed that the reperfusion damage that occurs after myocardial infarction is under control [ 58 – 60 ]. Kakadiya et al. also confirmed the protective effect of hesperidin in experimentally induced myocardial infarction in diabetic rats [ 58 ]. Indeed, hesperidin could elevate the systolic and diastolic blood pressure in diabetic rats and also could regulate the vasomotor function and hence can be used in the prevention and treatment of cardiovascular disease. It is also revealed that hesperidin could prevent the secretion of potent contracting factors, like endothelin (ET-1), and also increase the release of NO from endothelial cells [ 61 , 62 ].
Citrus juices could reduce the cholesterol level in the human organism that is resulted from the presence of some types of flavanone derivatives (like naringin and hesperidin). The main characteristic of these flavanones is the presence of a hydroxyl mevalonate moiety (brutieridin, melitidin, and 3-hydroxy-3-methylglutaryl-glycosyl-quercetin) with a similar structure to statins, which is widely used for the inhibition of cholesterol biosynthesis. It is shown in literature that utilizing eriocitrin and hesperetin metabolites could effectively lower the amount of lipids in high-cholesterol-fed rats [ 42 ]. Using rich antioxidant daily diet could be helpful in preventing atherosclerosis via reducing LDL oxidation. Essential oils from lemon could also prevent LDL oxidation and reduce the amounts of plasmatic cholesterol and triglycerides [ 42 ].
4.2. Antidiabetic Activity
Hesperidin has significant effects on insulin and glucose metabolism. Disruption in the regulation of blood glucose is the main pathological event in diabetes mellitus. Studies have shown that hesperidin reduces glucose and lipid levels by activating glucose-regulating enzymes [ 63 ]. It has been shown to reduce possible complications of diabetes as it exerts antioxidant effects and suppresses proinflammatory cytokines. The antihyperglycaemia effect of Citrus flavonoids is reported by a study completed on HepG2 cells. The flavonoids tested (hesperidin, naringin, nobiletin, and neohesperidin) inhibited starch digestion, catalyzed by amylase enzyme. They also enhanced hepatic glycolysis activity and glycogen deposition, showing potential to prevent hyperglycaemia [ 64 ].
4.3. Anticancer Activity
Cancer is a broad group of diseases resulted from unregulated cell division and growth. These irregular cells need a source for their abnormal energy metabolism, and glucose is regarded as a preferred metabolic substrate for them. By using antioxidant-rich foods with phenolic compounds and other types of antioxidant phytochemicals, the oxidative stress and its consequences like DNA mutations and cancer development can be prevented. Citrus flavonoids are considered quite safe and nontoxic drugs that can act as modulators of tyrosine kinases that regulate apoptosis and have antiproliferative activities. In both in vitro and in vivo studies, flavanones have been shown to inhibit tumour growth and stop cell cycle, inducing cancer cell apoptosis by death receptors and caspase-related mitochondrial pathways [ 65 ]. Aranganathan et al., studied the inhibition effect of Citrus flavonoids on oral carcinogenesis and their antineoplastic activity in hamsters. The results of this study revealed that while hesperetin, neohesperidin, tangeretin, and nobiletin were ineffective, naringin and naringenin showed good results [ 66 ]. The preventing effects of hesperetin on multiple cancer types, like breast and colon cancers, were confirmed in previous research [ 67 ]. Essential oils are also currently accepted as the anticarcinogenic agents. The application of citral as a cancer chemopreventive agent against inflammation-related carcinogenesis (like skin cancer and colon cancer) has been reported [ 53 ]. Besides, the beneficial effect of the vitamin C and dietary fibre in prevention and treatment of cancer has also been approved [ 68 – 71 ]. In a study, flavonoids in the extract of Citrus aurantium L. induced apoptosis in human leukaemia cells via inhibition of protein kinase B [ 72 ]. In another study, the extract of the same fruit induced cell cycle arrest and apoptotic death in non-small-cell lung cancer cells (A549) [ 73 ]. On these cell lines, the flavonoids obtained from Citrus platymamma extract showed similar effects, in addition to anti-inflammatory and antiangiogenic features [ 74 ]. Studies have shown that although the normal concentration of Citrus flavanones in oranges is insufficient to induce apoptosis in cancer cells, it could prevent the disease [ 74 ].
4.4. Antimicrobial Activity
Many studies have shown the antimicrobial effects of flavonoids. Moon et al. studied flavonoids' antimicrobial effects on Helicobacter pylori strains in which it is confirmed that hesperetin and naringenin had the strongest antimicrobial effect [ 71 ]. In another study, the antibacterial properties of bergamot extract have been shown to be more effective in Gram-negative bacteria [ 75 ]. The antimicrobial activity of naringin and its derivatives against Gram-positive bacteria were also confirmed in other research [ 76 ]. The C . aurantium flower has shown to have high antioxidant activity and broad-spectrum antibacterial activity. Phenolics and flavonoids have been confirmed to have antioxidant and antimicrobial properties in the samples tested. As a result, this herb can be used as an antimicrobial agent for functional food and medical application [ 77 ]. The mechanism of the antimicrobial activity of flavanones is thought to be the breakdown of the bacterial membrane and the interaction of bacterial DNA synthesis and microbial enzymes.
4.5. Neuroprotective Activity
Neurological disorders have rapidly become a significant and growing problem. The most common neurological disorders are Alzheimer's disease, Parkinson's disease, Huntington's disease, and ischaemic brain injury in which the oxidative stress and neuroinflammation are seen as the pathogenesis [ 78 ].
Alzheimer's disease is a progressive neurodegenerative disorder, which is pathologically characterized by the accumulation of beta-amyloid that leads to the cytotoxic effects in neuronal cells via inducing mitochondrial dysfunction and apoptosis. Utilizing hesperidin could prevent the mitochondrial dysfunction via opening the mitochondrial permeability transition pores, increasing the intracellular free calcium, and preventing the production of reactive oxygen species (ROS) [ 78 ]. Parkinson's disease is known as the second most common neurodegenerative disease in the world that is resulted from the progressive loss of dopaminergic neurons within the substantia nigra. With the antioxidant effect of hesperidin, it can increase the cellular glutathione content and protect Parkinson's disease [ 79 ]. Epilepsy is another type of neurological disease, which is characterized by current episodes of convulsive seizures, loss of consciousness, and sensory disturbances. Hesperidin could improve the treatment with epileptic conditions via affecting the NO–cyclic guanosine monophosphate (cGMP) pathway [ 80 ].
Polyphenols could also affect the psychiatric disorders, which are abundant and a major health problem in the world. Citrus aurantium is frequently used for central nervous system disorders. Anxiolytic activity was determined by soothing activity with increased plus spent time in open arms of the maze and widening of barbiturate sleep time. No disturbances in attention status or motor activity were observed in rats treated with essential oils. The biological effect may result from a specific compound or, more commonly, synergistic effect among several compounds. Limonene (97.83%) and mirsen (1.43%) are the main essential oils of Citrus aurantium , which are found in about a tenth of these amounts and have the ability of acting against depression of the central nervous system [ 81 ].
4.6. Miscellaneous Activities of Dietary Fibres, Vitamins, and Minerals
Dietary fibres (in both soluble and insoluble forms) show various beneficial effects on human health. The fibres of Citrus fruits, either soluble or insoluble ones, are beneficial to health and provide the benefits such as removal of toxins, enhancement of nutrient adsorption in the gastrointestinal system, lowering the energy absorption, and help to proper functioning of the liver and bile duct [ 82 ]. Apart from phytochemicals and fibre components, vitamins and minerals play a crucial role in maintaining the human health. The Citrus genus is an important source of vitamin C and B derivatives. Vitamin C possesses antioxidant activity and helps remodelling of connective tissue as well as enhances iron adsorption. Thiamin (vitamin B1) regulates neural functions and acts as a cofactor for muscle contraction and relaxation. Folic acid (vitamin B9) has a role in nucleic acid metabolism. Riboflavin (vitamin B2) takes action in reduction reactions in the cells and acts as a coenzyme in oxidation reactions. Macro- and microelements including magnesium, calcium, potassium, phosphorus, zinc, selenium, manganese, sodium, and copper help to proper functioning of several systems and organs. For instance, potassium plays a crucial role in ion homoeostasis and has a role in neural health, and magnesium plays a key role in muscle contraction and relaxation [ 82 ].
By-products from the lemon industry are rich sources of dietary fibre, which could play a critical role in preventing the occurrence of different types of digestive diseases, like constipation, haemorrhoids, hypercholesterolaemia, and colorectal cancer [ 83 – 85 ]. Due to the natural ability of citrate in inhibition of urinary crystallization, the citric acid of Citrus fruits could be used for calcium urolithiasis therapy [ 86 ]. According to several literature works, Citrus fruits are rich sources of flavanones with a wide range of physiologic and pharmacologic effects. The therapeutic effect of hesperidin and its aglycone hesperetin on several types of diseases was confirmed via different preclinical and clinical studies. This therapeutic capability is resulted from different interesting properties of these components like their antioxidant, anti-inflammatory, lipid-lowering, and insulin-sensitizing abilities. Citrus flavonoids have proved to be very valuable due to their antioxidant, antiallergic, vasotonic, anti-inflammatory properties, and antimicrobial activities. They are also one of the substances recognized by the FDA as generally safe. Therefore, in functional foods, it makes sense to assume their potential as a component that will increase human health and prevent both roughness and microbial contamination. Various important bioactivities of the Citrus genus are presented in Figure 2 .

Various important bioactivities of Citrus genus.
5. Beneficial Role of Citrus Genus in Food Application
Citrus fruits such as oranges, tangerines, limes, grapefruits, bitter oranges, and lemons are consumed daily, either raw or processed, due to the abundance of valuable nutrients such as vitamins, fibres, minerals, organic acids, and various phytochemicals. Therefore, they possess several nutritional and health benefits and are consumed as bare fruits or processed products such as peels, pulps, juice, and other extracts that are commercialized and used as additives in the food industry. As an example, bitter orange extracts are employed as aroma in several beverages, desserts, sweets, drinks, and ice creams. The juice of bitter oranges is used in salads, and the peel is added to the marmalade. In some countries, bitter oranges are the main part of regional cuisine, either raw or processed.
The third most important species in Citrus genus is lemon. Lemon, as mentioned in many studies, is important due to its health-promoting activities. In addition, it is an important source of phenolic compounds, vitamins, minerals, dietary fibre, essential oils, and carotenoids [ 87 ]. In the fresh products market and food industry, the lemon fruit has gained strong commercial value. Although the productive networks of lemons have generated high amounts of wastes, they can be used as a rich source for by-product technology, with potential for animal feed, manufactured foods, and health care [ 88 ]. Section 5 deals with the application and health benefits of Citrus by-products. Citrus has gained importance for its use in the food industry and its phytochemistry; analytical aspects of lemon compounds relevant with their nutrition and health are mentioned here by bringing an overview of what is published on the bioactive compounds of this fruit [ 89 ]. Gene expression may alter by dietary phytochemicals, which is a different subject of study. The influence of diet on health of an individual basis is the principle of understanding the guidance about nutrigenomic approaches. Human health can be optimized by genes and nutritional genomics in a futuristic way for food processing technology [ 90 ]. Gene expression can be regulated by bioactive components of the diet at protein abundance, transcriptome, and protein turnover level. The activation state of target molecules and their gene expression modulation are current research scenario, which are providing large evidence about the role of flavonoids in diet. Citrus flavonoids reported to have regulation effects on gene expression by coding low-density lipoprotein receptor (LDLR) expressions. Reports have shown several genes (COX2, microsomal cytochrome P450 A1, and NF-kB) modulation by hesperidin, naringenin, and hesperetin, which are present in Citrus . These bioactive compounds have gained attention for their recognition as characteristic biological effect on genes. However, implication of lemon in nutrigenomic therapy is still lacking with authentic evidence.
Citrus essential oils, extracted from different parts of Citrus plants were used as food additives due to their antimicrobial, flavouring, and antioxidant properties and for the preservation of raw foods (i.e., fruits, vegetables, meat, and fish), processed foods, and animal breeding. They are regarded as safe and involved in several food industries. As preservatives, essential oils are added to the thin and edible films to protect vegetables and fruits from dehydration and microbial spoilage, extending the shelf life [ 91 ]. As an example, bergamot essential oil is blended with chitosan and hydroxypropyl methylcellulose and applied on muscatel grape to protect the quality of the fresh fruit in cold storage. The essential oil contributed to improved antimicrobial activity, inhibition of respiration rate, control over water loss, and enhanced mechanical property of muscatel table grape [ 92 ]. In another example, lemon essential oil exhibited antibacterial activity against E . coli and S . enterica and used as an additive for the preservation of apple juice, without any change in antibacterial activity upon increased storage time [ 93 ]. The antibacterial activity of these essential oils can be further increased via encapsulation methods. Nanoencapsulated mixture of terpenes and D-limonene showed improved antimicrobial activity against food-borne pathogens including Lactobacillus delbrueckii , Saccharomyces cerevisiae , and Escherichia coli , thus used as a preservative in fruit juices without affecting the organoleptic features of the juices [ 94 ]. For meat and fish, essential oils provide improvement of tenderness, texture, flavour, aroma, and juiciness and protect from microbial invasion and oxidative deterioration. In some cases, essential oils are blended with the freezing water of ice for preservation and storage of fish and meat to extend their freshness and shelf life [ 91 ].
Another use of Citrus essential oils in the food industry is flavouring the processed foods and beverages. The flavour is mostly provided by essential oils extracted from peel, dominated by the terpenoids. As an example, linalool and linalyl acetate, found in bergamot oil, have characteristic flavours and used in sweets and jams in different countries [ 93 ]. Phenolic compounds along with essential oils from Citrus peel showed antioxidant activity, and this activity was proven for the meat preservation in the food industry. Except for synthetic antioxidants to preserve meat quality, kinnow rind powder was applied for meat preservation and exhibited radical scavenging activity, thus proposed as a safer alternative to synthetic antioxidants [ 6 ].
6. Citrus Waste and Its Utilization
Citrus fruits are considered the most consumed crop after grapes in the world crop production ranking. Citrus has attractive colours and distinctive flavours and considered the highly richest source of vitamin C. Impressive research works on Citrus fruits consumption were carried out and accumulated by workers to serve the needs of food processing. The raw materials of Citrus production are used for cordials and soft drinks, pasteurized, concentrates, preservatives and frozen canned juices, candied peels, flavouring oils, and marmalades in food industries [ 3 , 95 , 96 ]. The Citrus worldwide production was 137.8 million tonnes in 2014, and it is increasing every year. European production of Citrus was 11.3 million tonnes, while Spain (62%), Italy (24%) and Greece (9%) are in the leading positions [ 97 ]. Citrus has an important effect on the world economy due to its usage in producing different products like orange juice, marmalade, Citrus honey, and essential oils. Citrus fruits generate more than 70% higher amounts of Citrus waste, only 50 to 65% of which is processed. The waste of Citrus includes seeds, peels, and membrane residues. To prevent the serious pollution in environment, it is critical to process the huge amounts of Citrus waste [ 98 ]. There are numerous utilization avenues of the Citrus waste that is discussed in the following subsections.
6.1. Citrus Waste and Biofuel Production
Citrus fruits waste has soluble and nonsoluble carbohydrate polymers, which can be converted to biofuels like biogas and ethanol. The orange peel pressing liquid and Citrus waste can be used for methane production by anaerobic digestion, while cattle feed can be produced by orange peel degradation [ 99 ]. Citrus waste can be processed under different conditions such as mesophilic or thermophilic bacterial treatments, co-digestion, municipal waste, and other vegetable and fruit wastes as co-substrates will provide useful biomass products. The anaerobic digestion with use of mesophilic bacteria is considered a cheap and easy treatment for agroindustrial waste management. The limonene and linalyl acetate, which are abundantly present lipid components, can be degraded by combined action of fungi, yeasts, and bacteria with concentrations of 300 ppm [ 100 ]. The rate of methane production is increased via increasing the amounts of organic and decreasing the hydraulic retention times in thermophilic processes, but these are more sensitive and less advantageous in terms of energy consumption compared with mesophilic conditions [ 101 ]. A strong inhibition property was observed in essential oils of Citrus , which represent 2–3% of dry matter of Citrus peel. However, limonene treatment showed inhibition of fermentation at concentrations of 0.05–0.20%. There is a need to remove inhibitory compounds by biorefinery approaches to have proper ethanol or biogas production [ 102 ]. The production of ethanol is complicated since different types of enzymes or chemicals are needed for converting polymers into sugars before sugar fermentation. Nowadays, bacterial digestion, which requires hydrolysis of polymers and then conversion to biogas, is used for ethanol production because of high market demand [ 103 ]. Citrus wastes treated with Candida albicans , Kluyveromyces marxianus , Saccharomyces cerevisiae , and Zymomonas mobilis showed 90% of bioethanol production [ 104 ]. Waste biomass can be used for biofuel production, but essential oils and pectin must be extracted first, and then ethanol or methane from lignocellulosic and hemicellulose residues can be produced. However, it requires strong expertise to correctly run some modern techniques that are difficult in small or medium enterprises because they have simpler treatment schemes and technologies. So, small enterprises can enhance biochemical processes by treating the substrate by anaerobic digestion as a simple treatment technique production, but period is limited to about half a year. Therefore, necessary requirements and better control to operate the anaerobic digester continuously over the time of whole year is required. Such ways provide less investment and operational cost in biogas production [ 105 , 106 ]. One aspect of these treatments that must not be neglected is Citrus waste treatment and use of anaerobic methods on commercial level by using anaerobic reactors to have less environmental pollution. Anaerobic digestion is a promising solution for processing of stored waste with less operational cost compared with fresh Citrus wastes [ 104 , 107 ].
Most of our food spoils and losses are done by oxidation during processing, transportation and storage, and microbial contamination. But, nowadays, preservatives are added for shelf life extension of food products. Mostly, we use synthetic or chemical preservatives that had harmful effects to human health and at same time due to agricultural and ecological environment issues [ 108 ]. The food-borne pathogens and oxidation led to food quality and safety issues. Therefore, it is need of the modern era to find natural antioxidants as good substitutes for synthetic antioxidants [ 109 ]. Recently, in the current era, the trend of research changed to use natural preservatives from plant extracts or essential oils to enhance natural products consumption and at same time to overcome the environment pollution issues.
6.2. Citrus Waste and Food Preservation against Spoilage Microbes
In the agroindustrial sector, 50–60% of total Citrus production is mostly from cultivated lemon, mandarin, lime, and grapefruit worldwide. The Citrus fruit plants contain many essential oil sources. These essential oils are liquids and volatile plant secondary metabolites and are considered generally recognized as safe (GRAS) by the US Food and Drug Administration. Essential oils provide biological activities, including antioxidant, antimicrobial, anticancer, insecticidal, and anti-inflammatory properties [ 110 ]. There are more than 200 components identified in lipid components of Citrus , which are commonly made up of aldehydes, ketones, esters, acids, terpenes, and alcohols. Essential oils have gained numerous applications against food-borne or spoilage microorganisms in the food industry. Further studies showed that major essential oils show antimicrobial ability that is related to additive, synergistic, and antagonistic effects. The antimicrobial ability of these essential oils and major components against bacteria and yeasts showed that these are more susceptive to Gram-positive bacteria, Gram-negative bacteria, and yeasts relative to bacteria. Citrus medica var. sarcodactylis Swing and Citrus Changshan huyou B. Chang species showed high antimicrobial activity [ 111 ]. While some essential oils from species of Citrus Changshan huyou B. Chang and Citrus medica var. sarcodactylis Swing showed strong free-radical scavenging activity [ 112 ].
6.3. Citrus Waste and Bioactive Compounds
The main residue resulted from the processing of Citrus is its peel waste that contained high water content and high amounts of essential oils and biomass. These Citrus peel wastes, considering economic and environmental factors, have gained numerous applications and potential uses in pectin and dietary fibres production in the food industry, animal feeds to provide nutritional value, organic soil conditioner, and as substrates in compost production to improve the organic matter content of the soil in agricultural land [ 113 ]. Citrus peel wastes can be used for the production of flavouring agents, flavonoids, and citric acid for cosmetic and pharmaceutical industries. Citrus peel waste produced during Citrus fruits processing is 50–70%, so there is need for technology adoption to convert this waste into useful products [ 114 ]. But higher concentrations and low pH of organic compounds present in Citrus peel waste are the main problems for biological management options. So, there is need for management of economic and environmental factors to extract essential oils from large amounts of Citrus -processing residues to have beneficial and environment-friendly usage in agrofood industries [ 5 ]. Each part of Citrus plants, including seeds, fruits, pulps, juice, peel, and leaves, contains several types of phytochemicals, which are considered anti-inflammatory, antidiabetic, antithrombotic, anticarcinogenic, antidepressant, antifungal, and antiviral. These ingredients are useful for the treatment of chronic diseases, metabolic syndrome, hypertension, obesity, and diabetes by lowering the blood cholesterol level [ 82 , 115 ]. Hesperidin and quercetin as flavonoids participate in defence against herpes virus and polioviruses [ 82 ]. Naringenin is known to accelerate carbohydrate metabolism, dismiss reactive oxygen species, involve in fatty acid metabolism, prevent lipid deposition in the liver, take part in immune defence, and prevent atherogenesis and inflammation [ 116 ].
The extraction of value-added polyphenols from various citrus peels—a quick, sustainable, and economical technique—with low instrumental needs and operating simplicity has been developed. The proposed extraction approach may be simpler to carry out than alternative extraction procedures such as UAE, MAE, or ASE, particularly on a large scale, and may also have reduced economic expenses. Temperature should be set at 90°C for the maximum extraction efficiency for all examined analytes; however, for a specific polyphenol, temperature might be set at 62°C and the ethanol ratio dropped to 20% ( v ) under some extraction conditions. As a result, the cost of heating or using organic solvents is reduced. However, the economic gain obtained from the polyphenols extracted should pay for the global cost of the extraction procedure at industrial scale due to heating or solvents. 100 g of synthetic trans-ferulic acid might cost 116 €, 100 g of hesperidin 124 €, 100 g of rutin 128 €, and 100 g of p-coumaric acid 410 €, according to an estimate. Using the proposed process, each gram of clementine peels yields up to 673 mg of hesperidin, and each gram of orange peels yields 4.7 mg of rutin. The amounts extracted are significantly higher than those reported by other researchers. Overall, the findings revealed that clementine peel may be a significant and plentiful source of health-promoting bioactive phenolic compounds, particularly hesperidin, but orange peel (29) may have higher rutin concentration. Furthermore, the LC-MS/MS study confirmed particularly high levels of naringin, which was isolated mostly from lemon peels. The most abundant extracted polyphenol from all citrus matrices was hesperidin, which has shown promising therapeutic properties as a UVA irradiation and oxidative stress protector [ 117 ].
Experimental design and complementary chemometric tools (RSM, MRA, multifactorial-ANOVA, and PCA) have, on the other hand, proven to be extremely useful in analyzing and summarizing large amounts of data, as well as in facilitating the recognition of relevant underlying information and determining the best extraction conditions. As a result, the study's optimum conditions might be utilized as a guideline for future pilot plant-scale experiments and industrialization of the extraction process. Furthermore, the proposed technologies CLC-DAD and LC-MS/MS have given a simple and efficient strategy for the fast characterization and quantification of recovered phenolic natural compounds [ 118 ].
As a result, evaluated citrus peel waste could be reused and valorized, reducing environmental impacts and converting into value-added products, with potential interest in the development of functional foods, cosmetics, or likely preventive therapies for certain diseases, thereby adding value to Citrus -processing waste and companies.
6.4. Citrus Waste and Food, Pharma, and Other Applications
Nowadays, basic waste management and valorization strategies are used for processing of most agricultural wastes to obtain animal feed, fertilizer, composts generation, and anaerobic digestion of waste to produce biogas. Due to the high costs of transport, storage, and drying of these wastes as well as the environmental issues, their recycling is a critical process [ 1 ]. Solid waste can be utilized for by-products, which become more valuable than the main products as agricultural industries generate main products about 10–60% only from raw materials. Skins, leaves, seeds, unusable pulp, and wastewaters are 40% of the total plant food in Citrus fruits, mango, pineapple, papaya, artichoke, and asparagus, as these are usually discarded and can be converted to main by-products. These food by-products are rich in organic acids, minerals, dietary fibre, sugars, and bioactive compounds and contain some polyphenols and carotenoids. Thus, this led to growing interest to find their use as natural ingredients alternative to synthetic ingredients in production of high-value compounds, as they are used in different industrial fields such as nutraceutics, food, cosmetics, and pharmaceutics [ 119 , 120 ].
Recent studies focus on newer techniques to use Citrus waste for applications in chemical industries. Pulps, peels, seeds, and membrane residues (40–60% of the whole fruit) in Citrus waste exceeds to about 110–120 million tons per year in the world. Food processing, pharmaceutical, and chemical industries have developed biodegradable polymers and functional materials by organic acids obtained from Citrus wastes [ 121 ]. There are current investigations on peel microstructures to develop techniques for bio-inspired materials and production of important chemicals from the Citrus waste to have environment-friendly and economical aspects. Many bioactive molecules, biogas, fuels, and ethanol were extracted from Citrus waste by physicochemical and microbial processes and applied in food and pharmaceutical industries [ 122 ]. The organic acids extracted from Citrus waste were used to derive biodegradable polymers. These polymers gain importance in reducing pollution to a substantial level, human health and medical treatment, and production of commodity products. The other important usage of the wet biomass is their application in bioimaging and energy storage materials and for electrochemically active microbial fuel cells [ 123 ]. The future of complete valorization of biomass to have zero waste left behind depend solely on industrial researchers, Citrus -processing industries, collaboration among farmers, and transportation systems, and also there is a need for integrated research incorporating active coordination to realize a future of green, clean, and safe environment for next generations [ 121 , 124 ].
In developing countries, the agricultural waste is often left in environment without any treatment, which is accompanied by the elimination in the agricultural by-products and environmental pollution. The efficiency of reduction of recycling and economic costs can be done by treating biowaste as a rich resource of high valuable materials and reuse it by biowaste-to-resource (BTR) systems. In addition, there is a need for silage making of Citrus waste collected at the farm level if farmers want to store it for further processing because it cannot be transferred to long distances. In addition, more care must be taken in silage storage and it must be well sealed as it is easily polluted by certain climate conditions such as humidity. The farmers can use these silages for their poultry, especially in seasons that feeding supply is not good (like winter or spring) [ 6 , 125 ].
Recent research focused on functional properties of Citrus by-products as a low cost and ease accessible nutraceutical resources for nutritional dietary supplements. These can be used in the production of value-added food supplements, which are rich in bioactive compounds, advantageous dietary fibres, and polyphenols. These by-products can improve emulsion by their noncaloric bulking agents and oil retention and enhance water abilities. Thus, oxidative stress is reduced, which prevents human health from a wide range of diseases. A good source of bioactive compounds is fruit peel extract from the food industry, which helps overcome pollution problems caused by poor disposal peel residues. However, there is wide research needed to explore the in vivo potential of Citrus peel and its benefits in bioavailability [ 126 , 127 ].
Cheese is an ideal source of milk fat, different types of minerals like calcium and phosphorous, proteins, and energy, which are required for human health. There are different varieties of cheese that vary from country to country due to colours, flavour, and texture, and it is valued for long life [ 128 ]. Cheese industry is still growing at rapid stage and needs new technologies, innovations, development, and sustainability. There is a need for consistent reproducible characteristics, high quality, and processing capability of food products in the food industry. But, growing demand for cheese, decrease in slaughtering of young calves, increase in price of calf rennet, and controversy in using rennet (whether obtained from halal source or not) lead to new innovative studies to find new valuable rennet substitutes [ 129 – 132 ]. In vegetarian population, less amounts of rennet are supplied by animals for cheese production. Therefore, finding alternatives in the milk coagulation process can be an economical way for cheese production in developed countries. Substitutes must have suitable thermal stability and high ratio of milk clotting to proteolytic activity like calf rennet. The microbial rennet that is caught from genetically engineered bacteria is an appropriate alternative; however, the natural vegetative coagulants with favourable properties have gained more attention [ 133 ].
There are many research studies on kiwi, melon, and papaya; some roots of ginger rhizome; papaya fruit latex; Sodom apple Calotropis procera ; and flowers of Cynara cardunculus and Centaurea calcitrapa as alternative vegetative proteases for milk clotting. These extracts gained importance and innovative applications due to their functional properties to impart unique flavour and texture retention in final products [ 134 ]. Acid proteases (aspartic proteases) obtained from organisms including plants and animals can be activated at acidic pH values due to the existence of two aspartic acid residues, which are located at their active sites and can be inhibited by pepstatin A. There are high levels of homology and three-dimensional structure of these enzymes as shown by amino acid sequences of aspartic proteases. Plant aspartic proteases participate in seed germination and degradation of stored protein during ripening because they are present mostly in seeds. Aspartic proteases had a role in stress responses. They have gained importance in the food industry due to stability in acidic conditions and their high activity in milk coagulation in cheese production [ 135 ]. During the milk coagulation process, the casein is hydrolyzed, so destabilizing the casein micelles by enzymes, in this respect chymosin enzyme (main component of calf rennet), is considered to be the best and most applicable method for milk clotting. Many regions of Mediterranean, West African, and Southern European countries are extensively using different plants of the Solanaceae family such as Solanum innacum , S. dubium Fresen, and W. coagulans Dunal for cheese production. Some plants have been applied as sources of milk-clotting enzymes [ 136 ]. In another study [ 137 ], the authors studied the milk-clotting enzymes used for cheese production via applying proteases obtained from plants [ 138 ]. Sometimes excessive proteolytic activity of many plant origins leads to bitter taste, poor cheese yield, and defects in their texture. Thus, there is a need to find alternative methods for the production of various high-quality cheese-making enzymes.
An enzyme in berry plants (berry's pulp and husk) also showed milk coagulation ability. Recently, a small shrub of Solanaceae (growing widely in Pakistan, India, Afghanistan, and Iran) having monomeric aspartic protease (molecular weight about 25 to 35 kD) showed proteolytic activity at pH 4.25 and 37°C with high yield and quality of cheese especially in cottage and cheddar cheese. There was a sensible bitter taste that was decreased by extended ripening period so biochemical characteristics of protease from W. coagulans are still to be explored [ 136 , 139 ].
There is an increased interest to explore new proteases for the coagulation process that could replace the calf rennet for cheese making, leading to characterize proteases development from Citrus flower extracts, evaluating milk-clotting and proteolytic activities of these extracts. There are many beneficial aspects in Citrus flowers (azahar) such as highly pleasant and desirable aroma, essential oils (by steam distillation), enzymes, proteins, and functional ingredients for health and nutrition [ 140 ]. Citrus aurantium ( bitter orange) is an ornamental tree grown in the State of Sonora, Mexico, but sadly most of its fruits and flowers are destroyed because of the rainy season and mechanical or other defects. Its seeds, flowers, peels, leaves, and pulp can be used more efficiently in agroindustrial products to have numerous benefits in economical, ecological, and technological ways. C . aurantium (sour orange) flowers were also evaluated in research studies to be used as an alternative natural source of milk-clotting proteases, and their protease ability is affected by different temperature and pH parameters in cheese-making processes. Crude flower extract showed 20 to 35 mg/mL of soluble protein content, which can be incremented up to about 85% of the total protein content at the final stage of floral growth. The proteases from Citrus flowers were adequate to clot milk over a broad pH range, and they have the capacity to hydrolyze different substrates. The inhibition of protease from Citrus flower extract with pepstatin showed it has abilities similar to chymosin. Thus, in bioprocesses such milk coagulation, Citrus flowers proved as new potential sources of proteases [ 130 , 133 ].
The crude flower extracts from Citrus fruits have many actions on protein substrates (caseins, albumin, and haemoglobin) because of milk-clotting activity. The Citrus flower offers a high potential if broad pH range for activity and high concentration of proteases used in different biotechnological processes [ 141 ]. There is also presence of putative proteases like chymosin in Citrus flowers that show proteolytic activity for cheese making at low pH. The recent advances to use the plants as good rennet substitutes for milk coagulation protease is no doubt more valuable, and these all vegetative coagulants from the plants have gained importance in biotechnological processes such as in medicine, food, and detergent industries [ 130 , 142 ].
Considering all the case studies, it is evident that Citrus waste contains essential oils and nutrients in large quantities. A biorefinery setup to extract these components from Citrus wastes will be an economical and environmentally friendly approach. In biorefinery processes, bioethanol produced by alcoholic fermentation and biomethane produced by anaerobic digestion processes of Citrus peel waste are considered useful for crops environmental conditioning and organic loading. The bioenergy conversion systems of Citrus peel waste, recovery of beneficial characteristics of lipid compounds, and nutraceuticals from Citrus peel waste can be improved with suitable techniques by lowering toxic effects of their essential oils [ 143 , 144 ]. Pectin, flavonoids, dietary fibres, citric acid, and flavouring compounds extraction from Citrus waste are expensive but have gained high market values. Their processing at large scale will open door for the development of large agriculture-devoted areas, and it will improve the economic and environmental aspects worldwide. However, serious notice must be taken on unauthorized Citrus waste disposal to overcome environmental risks regarding water and soil pollution [ 113 , 145 ]. Figure 3 presents various application of Citrus waste.

Various application of Citrus waste.
7. Conclusion
“Green consumerism,” the use of “friendly compounds” such as Citrus extract for food preservation, is the trend in food technology. Literature studies have shown that flavonoids and other bioactive compounds play important roles in imparting the biological activities to the Citrus extracts. It is evident from the discussed literature that Citrus extracts are potent antimicrobial, anticancer, anti-inflammatory, antidiabetic, cardioprotective, and neuroprotective agents. Extracts from Citrus have been successfully used in some food products to limit contamination and to prevent yeast spoilage. Citrus extract has been proven as a natural additive to some foods for improving the shelf life of the fruits. In addition, it is emerging as an important ingredient in the dairy sector for milk clotting. Developing novel techniques for exploring different applications of the chemicals that are derived from Citrus wastes is the main searching topic on recent investigations. Besides, using the Citrus peel could help lowering the pollution problems resulted from the poor removal of such residues. Also, more investigation ( in vitro and in vivo ) is needed for determining the bioavailability and real benefits of these peel extracts, which are obtained from Citrus peel.
Acknowledgments
The authors acknowledge that some of the icons used in figures are adapted from Flaticon.
Data Availability
Conflicts of interest.
The authors declare that they have no conflicts of interest.
Academia.edu no longer supports Internet Explorer.
To browse Academia.edu and the wider internet faster and more securely, please take a few seconds to upgrade your browser .
Enter the email address you signed up with and we'll email you a reset link.
- We're Hiring!
- Help Center
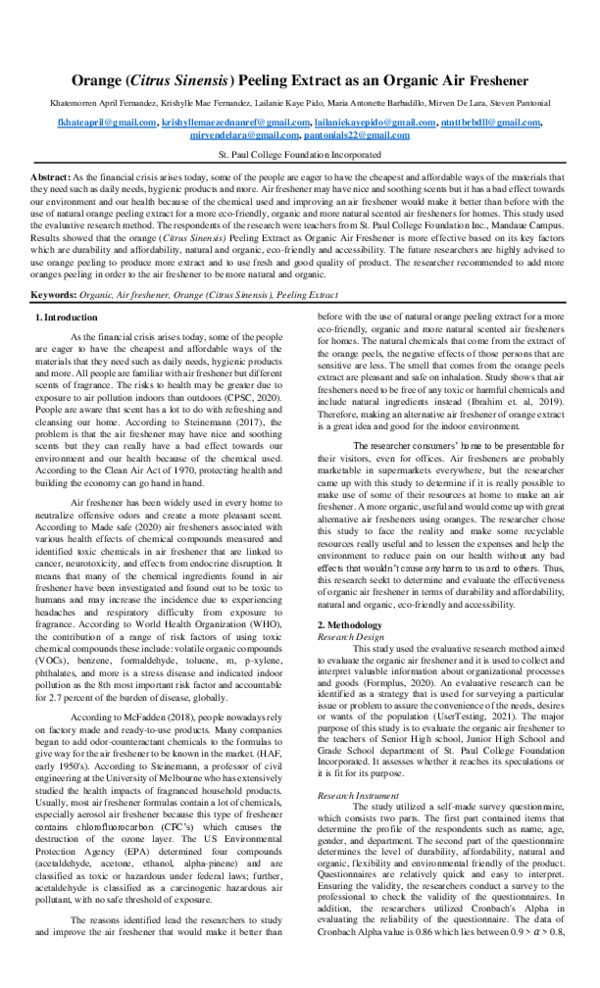
Orange (Citrus Sinensis) Peeling Extract as an Organic Air Freshener

As the financial crisis arises today, some of the people are eager to have the cheapest and affordable ways of the materials that they need such as daily needs, hygienic products and more. Air freshener may have nice and soothing scents but it has a bad effect towards our environment and our health because of the chemical used and improving an air freshener would make it better than before with the use of natural orange peeling extract for a more eco-friendly, organic and more natural scented air fresheners for homes. This study used the evaluative research method. The respondents of the research were teachers from St. Paul College Foundation Inc., Mandaue Campus. Results showed that the orange (Citrus Sinensis) Peeling Extract as Organic Air Freshener is more effective based on its key factors which are durability and affordability, natural and organic, eco-friendly and accessibility. The future researchers are highly advised to use orange peeling to produce more extract and to use fresh and good quality of product. The researcher recommended to add more oranges peeling in order to the air freshener to be more natural and organic.
Related Papers
Brazilian Journal of Chemical Engineering
Ricardo Batista
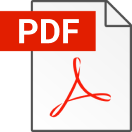
European Food Research and Technology
Peter Schieberle
IOP Conference Series: Earth and Environmental Science
Ika Atsari Dewi
Journal of Environmental and Public Health
hafsa ahmed
Air fresheners contain various chemicals that may or may not be harmful to human health and the environment. These products are widely used in different settings such as homes, schools, offices, and hospitals with ignorance of their real ingredients and their relative health effects. Thus, this preliminary study was carried out to identify the presence of different compounds in spray air fresheners that were not disclosed on the product’s label. Four different brands of spray air fresheners were selected randomly from a local store, in which two were of mid-to-high cost and the remaining two of low cost. The samples were analyzed using gas chromatography/mass spectrometry headspace, in which single components of the samples were identified by the mass spectrometry detector. The results were shown as a chromatogram of several peaks, each representing different compounds. The chemicals found in the samples include; lilial, galaxolide, benzenemethanol, musk ketone, butylated hydroxytol...
Mahmood Muhammad
Presently, in Nigeria orange peels are discarded as wastes after consumption of edible parts of orange fruits. However, the country depends on imported essential oil for use in industries for manufacture of products ranging from food, to beverage, cosmetics and pharmaceuticals. This research work was carried out to demonstrate the utilization of orange peel in the production of essential oils by employing different extraction methods. The methods considered in this work were steam distillation, water distillation and solvent extraction. For solvent extraction, Design Expert 7.0 was used to design experimental runs because of its relative popularity among the extraction methods. After the extraction, the oil was analysed to determine its physical and chemical properties. From the results obtained, it was discovered that the orange peels could give the maximum yields of essential oil to be 4.40%, 3.47% and 2.536% when steam distillation, water distillation and solvent extraction were ...
Journal of Agricultural and Food Chemistry
Journal of Advanced Thermal Science Research
Avanti Publisher
During the production of orange juice, more specifically after the commercial extraction of fruit juice, other waste materials are generated, consisting of peel, pieces of membranes, pulp bagasse, juice vesicles and seeds. In this way, the final destination of the waste can become a problem when not managed correctly. Therefore, there are several possibilities for using these solid residues, as they present substances of great commercial interest. In this perspective, the present work evaluates the recovery of orange essential oil from the citrus industry waste using hydrodistillation. The oil obtained was characterized by acidic index, FTIR, GC / MS, TGA and DSC. The results exhibited that oil isolated by hydrodistillation has a similarity with cold-pressed orange oil. The chemical constitution of oil obtained from waste was almost the same as the commercial orange oil analyzed. However, the thermal behaviour presents a few differences in thermal stability and vaporization temperature between analysed essential oils. Therefore, this work produces an alternative to obtain a product with quality, high yields and added value that can be used in cosmetic and pharmaceutical industries.
Journal of Medicinal Plants Research
Dalia Hamdan
The journal of phytopharmacology
DEEPTI KATIYAR
Loading Preview
Sorry, preview is currently unavailable. You can download the paper by clicking the button above.
RELATED PAPERS
Nguyễn Trần Minh Tú
Flavour and Fragrance Journal
P. Giampaoli , Julien Delarue , Martine Decloux
Umar Sahibzada
Türk Tarım ve Doğa Bilimleri Dergisi
Ahmet Alçiçek
Osvaldir Pereira Taranto , Karine Zanella
nitin satao
jean-marc brillouet
Aroma Research
Hirotoshi Tamura
Journal of Applied Horticulture
IOP Conference Series: Materials Science and Engineering
Natural Product Research
Domenico Schillaci
International Journal of Chemical Studies
Journal of New Sciences
NOURHENE BOUDHRIOUA
RELATED TOPICS
- We're Hiring!
- Help Center
- Find new research papers in:
- Health Sciences
- Earth Sciences
- Cognitive Science
- Mathematics
- Computer Science
- Academia ©2024
Comparative performance of fruit peel materials for methylene blue dye adsorption
- Original Paper
- Published: 16 September 2024
Cite this article
- P. Praipipat 1 , 2 ,
- P. Ngamsurach 1 , 2 ,
- K. Bunchu 1 ,
- V. Lekwaree 1 ,
- P. Srirat 1 ,
- P. Chaiphumee 1 ,
- J. Noisri 1 &
- T. Aeamsa-ard 1
The recycling fruit wastes of banana, pomelo, and mangosteen as biosorbents for eliminating methylene blue dye (MBD) were investigated in this study by synthesizing 3 materials of banana ( Musa ABB cv. Kluai ‘Namwa’) powder beads (BPB), pomelo ( Citrus maxima (Burm.f.) Merr) powder beads (PPB), and mangosteen ( Garcinia mangostana Linn) powder beads (MPB) and characterizing with several techniques. Their MBD adsorption performances were examined by a series of batch experiments, desorption experiments, adsorption isotherms, kinetics, and thermodynamics studies. MPB had a higher specific surface area and pore volume than BPB and PPB, whereas its pore size was smaller than theirs. All materials had amorphous structures. BPB had rough surfaces, whereas PPB had coarse surfaces with layer structures. MPB had rough surfaces with irregular shapes. They consisted of carbon, oxygen, calcium, chloride, and sodium. The chemical functional groups of hydroxyl, methine, carbonyl, and ether were detected in all materials. The pH pzc of BPB, PPB, and MPB were 5.41, 5.00, and 5.05. MPB showed a higher MBD removal efficiency of 98.92% and adsorption capacity ( q e ) of 6.59 mg/g than BPB and PPB, and all materials could be reused for 3 cycles with the adsorption efficiency of more than 61%. Their adsorption patterns and mechanisms were described by Freundlich and pseudo-second-order kinetic models. BPB and MPB were endothermic processes, whereas PPB was an exothermic process.
Graphical abstract

This is a preview of subscription content, log in via an institution to check access.
Access this article
Subscribe and save.
- Get 10 units per month
- Download Article/Chapter or eBook
- 1 Unit = 1 Article or 1 Chapter
- Cancel anytime
Price includes VAT (Russian Federation)
Instant access to the full article PDF.
Rent this article via DeepDyve
Institutional subscriptions
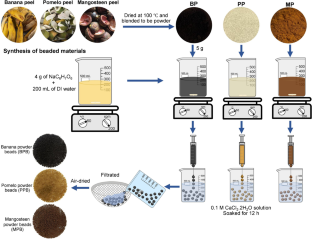
Explore related subjects
- Environmental Chemistry
Data availability
The data presented in the study are available upon request from the corresponding author.
Abbaz A, Arris S, Viscusi G et al (2023) Adsorption of safranin O dye by alginate/pomegranate peels beads: kinetic, isotherm and thermodynamic studies. Gels 9:916. https://doi.org/10.3390/gels9110916
Article CAS Google Scholar
Ahmed FS, Alsaffar MA, AbdulRazak AAJ (2022) One-step synthesis of magnetic fly ash composites for methylene blue removal: batch and column study. Environ Sci Pollut Res. https://doi.org/10.1007/s11356-022-23491-x
Article Google Scholar
Ajmal M, Siddiq M, Aktas N, Sahiner N (2015) Magnetic Co–Fe bimetallic nanoparticle containing modifiable microgels for the removal of heavy metal ions, organic dyes and herbicides from aqueous media. RSC Adv 5:43873–43884. https://doi.org/10.1039/c5ra05785j
Akter M, Rahman FBA, Abedin MZ, Kabir SMF (2021) Adsorption characteristics of banana peel in the removal of dyes from textile effluent. Textiles 1:361–375. https://doi.org/10.3390/textiles1020018
Aljar MAA, Rashdan S, El-Fattah AA (2021) Environmentally friendly polyvinyl alcohol−alginate/ bentonite semi-interpenetrating polymer network nanocomposite hydrogel beads as an efficient adsorbent for the removal of methylene blue from aqueous solution. Polymers (Basel) 13:4000. https://doi.org/10.3390/polym13224000
Allou NB, Tigori MA, Koffi AA et al (2023) Methylene blue magnetic adsorption separation process from aqueous solution using corn cob. Sci African 21:e01828. https://doi.org/10.1016/j.sciaf.2023.e01828
Azzaz AA, Jellali S, Bengharez Z et al (2019) Investigations on a dye desorption from modified biomass by using a low-cost eluent: hysteresis and mechanisms exploration. Int J Environ Sci Technol 16:7393–7408. https://doi.org/10.1007/s13762-018-2171-3
Bediako JK, Sarkar AK, Lin S et al (2019) Characterization of the residual biochemical components of sequentially extracted banana peel biomasses and their environmental remediation applications. Waste Manag 89:141–153. https://doi.org/10.1016/j.wasman.2019.04.009
Chen W, Chen F, Ji B et al (2019) Insights into the mechanism of methylene blue removed by novel and classic biochars. Water Sci Technol 79:1561–1570. https://doi.org/10.2166/wst.2019.158
Chikri R, Elhadiri N, Benchanaa M, El maguana Y (2023) Applications of sugar scum-sawdust composite for dye removal from aqueous solutions: kinetic and isotherm studies. Biomass Convers Biorefin 13:15949–15964. https://doi.org/10.1007/s13399-021-02110-8
Cwalinski T, Polom W, Marano L et al (2020) Methylene blue—current knowledge, fluorescent properties, and its future use. J Clin Med 9:3538. https://doi.org/10.3390/jcm9113538
de Lima RS, Tonholo J, Rangabhashiyam S et al (2024) Enhancing methylene blue dye removal using pyrolyzed Mytella falcata shells: characterization, kinetics, isotherm, and regeneration through photolysis and peroxidation. Environ Manage 73:425–442. https://doi.org/10.1007/s00267-023-01898-7
Dhar L, Hossain S, Rahman MS et al (2021) Adsorption mechanism of methylene blue by graphene oxide-shielded Mg-Al-layered double hydroxide from synthetic wastewater. J Phys Chem A 125:954–965. https://doi.org/10.1021/acs.jpca.0c09124
Dinh VP, Huynh TDT, Le HM et al (2019) Insight into the adsorption mechanisms of methylene blue and chromium(III) from aqueous solution onto pomelo fruit peel. RSC Adv 9:25847–25860. https://doi.org/10.1039/c9ra04296b
Gonzales C, Ubalde MC, Carpentero TC et al (2024) Efficiency of soda sludge–derived activated carbon in the removal of methylene blue dye from an aqueous solution. Mater Circ Econ. https://doi.org/10.1007/s42824-024-00103-2
Handayani T, Emriadi D et al (2024) Modelling studies of methylene blue dye removal using activated corn husk waste: isotherm, kinetic and thermodynamic evaluation. South African J Chem Eng 47:15–27. https://doi.org/10.1016/j.sajce.2023.10.003
Hock PE, Faizal ANM, Sirajo L, Zaini MAA (2023) Insight into kinetics, equilibrium, and thermodynamics of malachite green adsorption onto banana peel adsorbents. Biomass Convers Biorefin. https://doi.org/10.1007/s13399-023-04117-9
Ibragić S, Smječanin N, Milušić R, Nuhanović M (2022) Pomelo peel and sugar beet pulp as novel biosorbents in purification of biodiesel. Biofuels 13:755–762. https://doi.org/10.1080/17597269.2021.1920198
Ighalo JO, Iwuozor KO, Igwegbe CA, Adeniyi AG (2021) Verification of pore size effect on aqueous-phase adsorption kinetics: A case study of methylene blue. Colloids Surf A Physicochem Eng Asp 626:127119. https://doi.org/10.1016/j.colsurfa.2021.127119
Jangkorn S, Youngme S, Praipipat P (2022) Comparative lead adsorptions in synthetic wastewater by synthesized zeolite A of recycled industrial wastes from sugar factory and power plant. Heliyon 8:e09323. https://doi.org/10.1016/j.heliyon.2022.e09323
Jawad AH, Saber SEM, Abdulhameed AS et al (2022) Mesoporous activated carbon from mangosteen ( Garcinia mangostana ) peels by H 3 PO 4 assisted microwave: Optimization, characterization, and adsorption mechanism for methylene blue dye removal. Diam Relat Mater 129:109389. https://doi.org/10.1016/j.diamond.2022.109389
Kazemi J, Javanbakht V (2020) Alginate beads impregnated with magnetic Chitosan@Zeolite nanocomposite for cationic methylene blue dye removal from aqueous solution. Elsevier LTD
Khan I, Saeed K, Zekker I et al (2022) Review on methylene blue: Its properties, uses, toxicity and photodegradation. Water 14:242. https://doi.org/10.5040/9781501365072.12105
Lau KS, Azmi NAS, Chin SX et al (2023) Chitosan-bead-encapsulated polystyrene sulfonate for adsorption of methylene blue and regeneration studies: batch and continuous approaches. Polymers (Basel) 15:1269. https://doi.org/10.3390/polym15051269
Mallakpour S, Behranvand V, Mallakpour F (2021) Adsorptive performance of alginate/carbon nanotube-carbon dot-magnesium fluorohydroxyapatite hydrogel for methylene blue-contaminated water. J Environ Chem Eng 9:105170. https://doi.org/10.1016/j.jece.2021.105170
Mehrez M, Chaari I, Medhioub M (2024) Biosorption of a leather dye (Dycem TTO) onto banana peel: characterization, isotherm, kinetic, thermodynamic, and mechanism studies. Water Air Soil Pollut 235:119. https://doi.org/10.1007/s11270-024-06905-z
Momina MS, Suzylawati I (2020) Study of the adsorption/desorption of MB dye solution using bentonite adsorbent coating. J Water Process Eng 34:101155. https://doi.org/10.1016/j.jwpe.2020.101155
Ngamsurach P, Nemkhuntod S, Chanaphan P, Praipipat P (2022) Modified beaded materials from recycled wastes of bagasse and bagasse fly ash with iron (III) oxide-hydroxide and zinc oxide for the removal of reactive blue 4 dye in aqueous solution. ACS Omega 7:34839–34857. https://doi.org/10.1021/acsomega.2c03250
Oladoye PO, Ajiboye TO, Omotola EO, Oyewola OJ (2022) Methylene blue dye: toxicity and potential elimination technology from wastewater. Results Eng 16:100678. https://doi.org/10.1016/j.rineng.2022.100678
Oyekanmi AA, Ahmad A, Hossain K, Rafatullah M (2019) Adsorption of Rhodamine B dye from aqueous solution onto acid treated banana peel: response surface methodology, kinetics and isotherm studies. PLoS ONE 14:e0216878. https://doi.org/10.1371/journal.pone.0216878
Praipipat P, Ngamsurach P, Prasongdee V (2022a) Comparative reactive blue 4 dye removal by lemon peel bead doping with iron(III) oxide-hydroxide and zinc oxide. ACS Omega 7:41744–41758. https://doi.org/10.1021/acsomega.2c05956
Praipipat P, Ngamsurach P, Saekrathok C, Phomtai S (2022b) Chicken and duck eggshell beads modified with iron (III) oxide-hydroxide and zinc oxide for reactive blue 4 dye removal. Arab J Chem 15:104291. https://doi.org/10.1016/j.arabjc.2022.104291
Praipipat P, Ngamsurach P, Kosumphan S, Mokkarat J (2023a) Powdered and beaded sawdust materials modified iron (III) oxide-hydroxide for adsorption of lead (II) ion and reactive blue 4 dye. Sci Rep 13:531. https://doi.org/10.1038/s41598-023-27789-9
Praipipat P, Ngamsurach P, Pratumkaew K (2023b) The synthesis, characterizations, and lead adsorption studies of chicken eggshell powder and chicken eggshell powder-doped iron (III) oxide-hydroxide. Arab J Chem 16:104640. https://doi.org/10.1016/j.arabjc.2023.104640
Praipipat P, Ngamsurach P, Rattanavaru M et al (2023c) Synthesis and characterization of metal oxide dopped beaded sugarcane bagasse fly ash for direct red 28 dye removal. J Ind Eng Chem 25:495–514. https://doi.org/10.1016/j.jiec.2023.08.015
Praipipat P, Ngamsurach P, Thanyahan A, Sakda A (2023d) Reactive blue 4 adsorption efficiencies on bagasse and bagasse fly ash beads modified with titanium dioxide (TiO 2 ), magnesium oxide (MgO), and aluminum oxide (Al 2 O 3 ). Ind Crop Prod 191:115928. https://doi.org/10.1016/j.indcrop.2022.115928
Praipipat P, Ngamsurach P, Khamkhae P (2024a) Iron(III) oxide-hydroxide modification on Pterocarpus macrocarpus sawdust beads for direct red 28 dye removal. Arab J Chem 17:105514. https://doi.org/10.1016/j.arabjc.2023.105514
Praipipat P, Ngamsurach P, Libsittikul N et al (2024b) Cationic oxides and dioxides of modified sugarcane bagasse beads with applications as low-cost sorbents for direct red 28 dye. Sci Rep 14:1278. https://doi.org/10.1038/s41598-024-51934-7
Praipipat P, Ngamsurach P, Srirat P, Chaiphumee P (2024) Engineered biosorbents of pomelo (Citrus maxima (Burm.f.) Merr) peels modified with zinc oxide and titanium dioxide for methylene blue dye sorption. Sci Rep 14:5763. https://doi.org/10.1038/s41598-024-56499-z
Rashid R, Shafiq I, Akhter P et al (2021) A state-of-the-art review on wastewater treatment techniques: the effectiveness of adsorption method. Environ Sci Pollut Res 28:9050–9066. https://doi.org/10.1007/s11356-021-12395-x
Seifi S, Levacher D, Razakamanantsoa A, Sebaibi N (2023) Microstructure of dry mortars without cement: specific surface area, pore size and volume distribution analysis. Appl Sci 13:5616. https://doi.org/10.3390/app13095616
Sing KSW, Everett DH, Haul RAW et al (1985) Reporting physisorption data for gas/solid systems with special reference to the determination of surface area and porosity. Pure Appl Chem 57:603–619. https://doi.org/10.1351/pac198557040603
Singh S, Parveen N, Gupta H (2018) Adsorptive decontamination of rhodamine-B from water using banana peel powder: a biosorbent. Environ Technol Innov 12:189–195. https://doi.org/10.1016/j.eti.2018.09.001
Stavrinou A, Aggelopoulos CA, Tsakiroglou CD (2018) Exploring the adsorption mechanisms of cationic and anionic dyes onto agricultural waste peels of banana, cucumber and potato: adsorption kinetics and equilibrium isotherms as a tool. J Environ Chem Eng 6:6958–6970. https://doi.org/10.1016/j.jece.2018.10.063
Su H, Guo X, Zhang X et al (2022) Ultrafine biosorbent from waste oyster shell: a comparative study of Congo red and Methylene blue adsorption. Bioresour Technol Reports 19:101124. https://doi.org/10.1016/j.biteb.2022.101124
Usman MA, Aftab RA, Zaidi S et al (2022) Adsorption of aniline blue dye on activated pomegranate peel: equilibrium, kinetics, thermodynamics and support vector regression modelling. Int J Environ Sci Technol 19:8351–8368. https://doi.org/10.1007/s13762-021-03571-0
Waghmare C, Ghodmare S, Ansari K et al (2023) Experimental investigation of H 3 PO 4 activated papaya peels for methylene blue dye removal from aqueous solution: evaluation on optimization, kinetics, isotherm, thermodynamics, and reusability studies. J Environ Manage 345:118815. https://doi.org/10.1016/j.jenvman.2023.118815
Wang J, Guo X (2023) Adsorption kinetics and isotherm models of heavy metals by various adsorbents: an overview. Crit Rev Environ Sci Technol 53:1837–1865. https://doi.org/10.1080/10643389.2023.2221157
Yadav S, Asthana A, Chakraborty R et al (2020) Cationic dye removal using novel magnetic/activated charcoal/β-cyclodextrin/alginate polymer nanocomposite. Nanomaterials 10:170. https://doi.org/10.3390/nano10010170
Yu XL, He Y (2018) Optimal ranges of variables for an effective adsorption of lead(II) by the agricultural waste pomelo ( Citrus grandis ) peels using Doehlert designs. Sci Rep 8:729. https://doi.org/10.1038/s41598-018-19227-y
Zhang B, Wu Y, Cha L (2019) Removal of methyl orange dye using activated biochar derived from pomelo peel wastes: performance, isotherm, and kinetic studies. J Dispers Sci Technol 41:125–136. https://doi.org/10.1080/01932691.2018.1561298
Zhang Z, Xu L, Liu Y et al (2021) Efficient removal of methylene blue using the mesoporous activated carbon obtained from mangosteen peel wastes: Kinetic, equilibrium, and thermodynamic studies. Microporous Mesoporous Mater 315:110904. https://doi.org/10.1016/j.micromeso.2021.110904
Download references
No funding was received for conducting this study.
Author information
Authors and affiliations.
Department of Environmental Science, Faculty of Science, Khon Kaen University, Khon Kaen, 40002, Thailand
P. Praipipat, P. Ngamsurach, K. Bunchu, V. Lekwaree, P. Srirat, P. Chaiphumee, J. Noisri & T. Aeamsa-ard
Environmental Applications of Recycled and Natural Materials (EARN) Laboratory, Khon Kaen University, Khon Kaen, 40002, Thailand
P. Praipipat & P. Ngamsurach
You can also search for this author in PubMed Google Scholar
Contributions
Pornsawai Praipipat: Supervision, project administration, conceptualization, investigation, methodology, validation, visualization, writing–original draft, writing–review & editing. Pimploy Ngamsurach: Visualization, writing–original draft. Kanokwan Bunchu: Investigation. Varunya Lekwaree: Investigation. Pratchayaporn Srirat: Investigation. Punjaporn Chaiphumee: Investigation. Junjira Noisri: Investigation. Thani Aeamsa-ard: Investigation.
Corresponding author
Correspondence to P. Praipipat .
Ethics declarations
Conflict of interest.
The authors declare that they have no conflict of interest.
Ethical approval
Not applicable.
Consent to participate
Consent for publication, additional information.
Editorial responsibility: Maryam Shabani.
Supplementary Information
Below is the link to the electronic supplementary material.
Supplementary file1 (DOCX 29 KB)
Rights and permissions.
Springer Nature or its licensor (e.g. a society or other partner) holds exclusive rights to this article under a publishing agreement with the author(s) or other rightsholder(s); author self-archiving of the accepted manuscript version of this article is solely governed by the terms of such publishing agreement and applicable law.
Reprints and permissions
About this article
Praipipat, P., Ngamsurach, P., Bunchu, K. et al. Comparative performance of fruit peel materials for methylene blue dye adsorption. Int. J. Environ. Sci. Technol. (2024). https://doi.org/10.1007/s13762-024-06037-1
Download citation
Received : 27 April 2024
Revised : 21 August 2024
Accepted : 02 September 2024
Published : 16 September 2024
DOI : https://doi.org/10.1007/s13762-024-06037-1
Share this article
Anyone you share the following link with will be able to read this content:
Sorry, a shareable link is not currently available for this article.
Provided by the Springer Nature SharedIt content-sharing initiative
- Cationic dye
- Reusability
- Find a journal
- Publish with us
- Track your research

IMAGES
COMMENTS
This paper reviews the current literature on clinical and epidemiological investigations on the beneficial effects of citrus components on brain health and related functions. ... nobiletin-rich citrus peel extracts may improve cognitive function in the elderly and AD patients by antioxidant and anti ... A research group studied the impact of ...
The current review identified a research gap in utilizing citrus peel extract in the food industry. Many studies have already proven the utility of extraction of bioactive compounds from citrus peels and their biological properties. However, only a few studies have focused on the potential applications of these compounds in different food products.
Citrus peel, the primary waste, is a good source of molasses, pectin and limonene and is usually dried, mixed with dried pulps and sold as cattle feed (Bocco et al., 1998). Citrus peels are subdivided into the epicarp or flavedo (coloured peripheral surface) and mesocarp or albedo (white soft middle layer) as shown in Fig. 1.
The highest free radical scavenging activity (93.1%) of DPPH was exhibited by lemon peels, whereas the least activity (78.6%) was shown by mousami peels. Ethanolic extract of orange peels ...
In orange peel, there are two unique classes of flavones i.e. glycosylated flavanones and polymethoxylated flavones. [Citation 8] Flavanones are the most abundant group of flavonoids present in citrus peel comprising hesperidin (0.066 to 66.09 mg/g) and narirutin (0.03 to 26.5 mg/g), rarely present in other plants.
Citrus peel has untapped potential as a source of medicinal compounds because it contains carotenes, essential oils, pectin, and a range of polyphenolic compounds (3). Epidemiological studies have suggested that high consumption of fruits and vegetables (>400 g/d) can reduce cancer risk by ≥20% (4). The Mediterranean diet is rich in fruit ...
Orange peels, generally considered as waste, were treated with subcritical water (SWE)—a green technology and environmentally friendly extraction process—at different temperatures (120-200 ...
Citrus peels are a rich source of flavonoids, with concentrations ranging from 2.5 to 5.5 g/100 g dry weight, depending on the citrus variety. The most abundant flavonoids in citrus peel include hesperidin and naringin, as well as essential oils rich in monoterpenes like limonene. ... Feature papers represent the most advanced research with ...
To put it in a simple way, citrus peels have moved from the waste bin to the laboratory benches. The peels are been investigated as raw materials, ingredients or additives in the food industry, cosmetic industry and in the pharmaceutical and nutraceutical companies ( Rafiq et al., 2018 ).
Due to agronomic practices and climatic condition, the total flavonoids of citrus peel may vary accordingly. In orange peel, there are two unique classes of flavones i.e. glycosylated flavanones and polymethoxylated flavones.[8] Flavanones are the most abundant group of flavonoids present in citrus peel comprising hesperidin (0.066 to 66.09 mg ...
Citrus sinensis is one of the most abundant citrus species consumed. Orange peels are a waste by-product of the fruit and may potentially contain useful phytonutrients with biological relevance. Fresh and dry peels of sweet orange were subjected to Soxhlet extraction and then concentrated using a rotary evaporator. Total phenolic, flavonoid and tannin content were determined using standard ...
Orange peels, generally considered as waste, were treated with subcritical water (SWE)—a green technology and environmentally friendly extraction process—at different temperatures (120-200 °C) and extraction times (5-60 min). The extracts which were obtained were chemically and biologically characterised to evaluate this potential source of bioactive compounds. The extracts total ...
Citrus sinensis (L.) Osbeck is a very common cultivar belonging to the Rutaceae family. It is largely diffused in several areas of the world characterized by mild to warm climate conditions. Its abundant worldwide production (up to 107 Tons. per year) and consumption both as the edible part of the fruit and as several types of derivative products imply the production of a huge amount of waste ...
It is important to consider new research directions about the use of citrus fruit residues since it not only impacts the problem of its disposal but also provides solutions to eliminate the resulting contamination. ... more than a half of the fruit remains as a by-product. The orange peel represents the highest discarded waste (Olabinjo et al ...
3.1. Phenolic acids. Peels of citrus family fruits are a major source of phenolic acids. The amounts of free and bound phenolic acids was documented significantly higher in peels than in peeled fruits (Gorinstein et al., 2001).Four hydroxycinnamic acids (caffeic, p-coumaric, ferulic and sinapic acids) in free and bound form were reported in the methanolic extracts of calamansi (Citrus ...
Research has shown that calcium of vegetable origin is well absorbed from the human digestive tract , so losses of it should be limited by using citrus peels for consumption. Citrus fruits are also a valuable source of phosphorus, which together with calcium, participates in the formation of strong bones and teeth [ 22 ].
The extract of CS peel is the source of a huge variety. of phytochemicals and has been investigated on several applications including its. chemotherapeutic and chemopreventive potential for ...
Kinnow peel oil has been reported to possess antimicrobial and antioxidant activities due to presence of many bioactive compounds. Therefore, the experiments reported in this research paper aimed ...
Citrus (Citrus limetta, family Rutaceae) is the world's largest produced fruit, accounting for 23% of the world's total fruit production. Citrus peel (CP) is a potential source of certain essential oils and yields about 0.5-3 kg oil/tonne of fruit. The essential oils extracted are used for various purposes, such as pharmaceuticals, confectioner
This waste includes peels (50-55% of the total fruit mass), seeds (20-40% of total fruit mass), pomace, and wastewater. Citrus wastewater covers portions of spoiled fruit, seeds, pulp, and peels. Global citrus fruit processing generates approximately 10 million MT of waste each year (Zema et al., 2018), creating a serious ecological issue.
Citrus fruits such as oranges, grapefruits, lemons, limes, tangerines, and mandarins, whose production is increasing every year with the rise of consumer demand, are among the most popular fruits cultivated throughout the globe.Citrus genus belongs to the Rutaceae family and is known for its beneficial effects on health for centuries. These plant groups contain many beneficial nutrients and ...
Presently, in Nigeria orange peels are discarded as wastes after consumption of edible parts of orange fruits. However, the country depends on imported essential oil for use in industries for manufacture of products ranging from food, to beverage, cosmetics and pharmaceuticals. ... References Air Freshener (Research Paper Terms). Retrieved from ...
The recycling fruit wastes of banana, pomelo, and mangosteen as biosorbents for eliminating methylene blue dye (MBD) were investigated in this study by synthesizing 3 materials of banana (Musa ABB cv. Kluai 'Namwa') powder beads (BPB), pomelo (Citrus maxima (Burm.f.) Merr) powder beads (PPB), and mangosteen (Garcinia mangostana Linn) powder beads (MPB) and characterizing with several ...
The orange peel waste contains mainly cellulose, pectin, lignin, hemicellulose, and they are inevitable sources for renowned biomaterials synthesis. The orange peel also consists of about 5.4 kg of oil per 1000 kg oranges, through which around 90% of d -limonene can be obtained [[60], [61], [62]].