We have 2 roche PhD Projects, Programmes & Scholarships
All disciplines
All locations
Institution
All Institutions
All PhD Types
All Funding

roche PhD Projects, Programmes & Scholarships
Psychological causes and factors relevant to the development, maintenance and improvement of formal thought disorder in psychosis (project id shsc0057), phd research project.
PhD Research Projects are advertised opportunities to examine a pre-defined topic or answer a stated research question. Some projects may also provide scope for you to propose your own ideas and approaches.
Self-Funded PhD Students Only
This project does not have funding attached. You will need to have your own means of paying fees and living costs and / or seek separate funding from student finance, charities or trusts.
Fully funded PhD studentships at the EPSRC Centre for Doctoral Training in Statistics and Operational Research in Partnership with Industry (STOR-i)
Funded phd programme (students worldwide).
Some or all of the PhD opportunities in this programme have funding attached. Applications for this programme are welcome from suitably qualified candidates worldwide. Funding may only be available to a limited set of nationalities and you should read the full programme details for further information.
EPSRC Centre for Doctoral Training
EPSRC Centres for Doctoral Training conduct research and training in priority areas funded by the UK Engineering and Physical Sciences Research Council. Potential PhD topics are usually defined in advance. Students may receive additional training and development opportunities as part of their programme.
FindAPhD. Copyright 2005-2024 All rights reserved.
Unknown ( change )
Have you got time to answer some quick questions about PhD study?
Select your nearest city
You haven’t completed your profile yet. To get the most out of FindAPhD, finish your profile and receive these benefits:
- Monthly chance to win one of ten £10 Amazon vouchers ; winners will be notified every month.*
- The latest PhD projects delivered straight to your inbox
- Access to our £6,000 scholarship competition
- Weekly newsletter with funding opportunities, research proposal tips and much more
- Early access to our physical and virtual postgraduate study fairs
Or begin browsing FindAPhD.com
or begin browsing FindAPhD.com
*Offer only available for the duration of your active subscription, and subject to change. You MUST claim your prize within 72 hours, if not we will redraw.
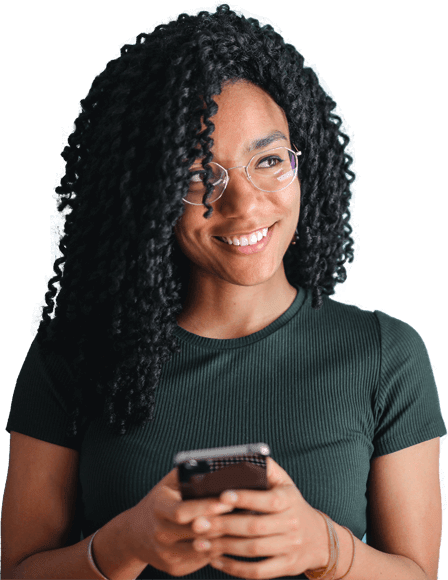
Do you want hassle-free information and advice?
Create your FindAPhD account and sign up to our newsletter:
- Find out about funding opportunities and application tips
- Receive weekly advice, student stories and the latest PhD news
- Hear about our upcoming study fairs
- Save your favourite projects, track enquiries and get personalised subject updates

Create your account
Looking to list your PhD opportunities? Log in here .
Filtering Results
- Press Enter to activate screen reader mode.
Department of Biosystems Science and Engineering
Doctoral programmes.
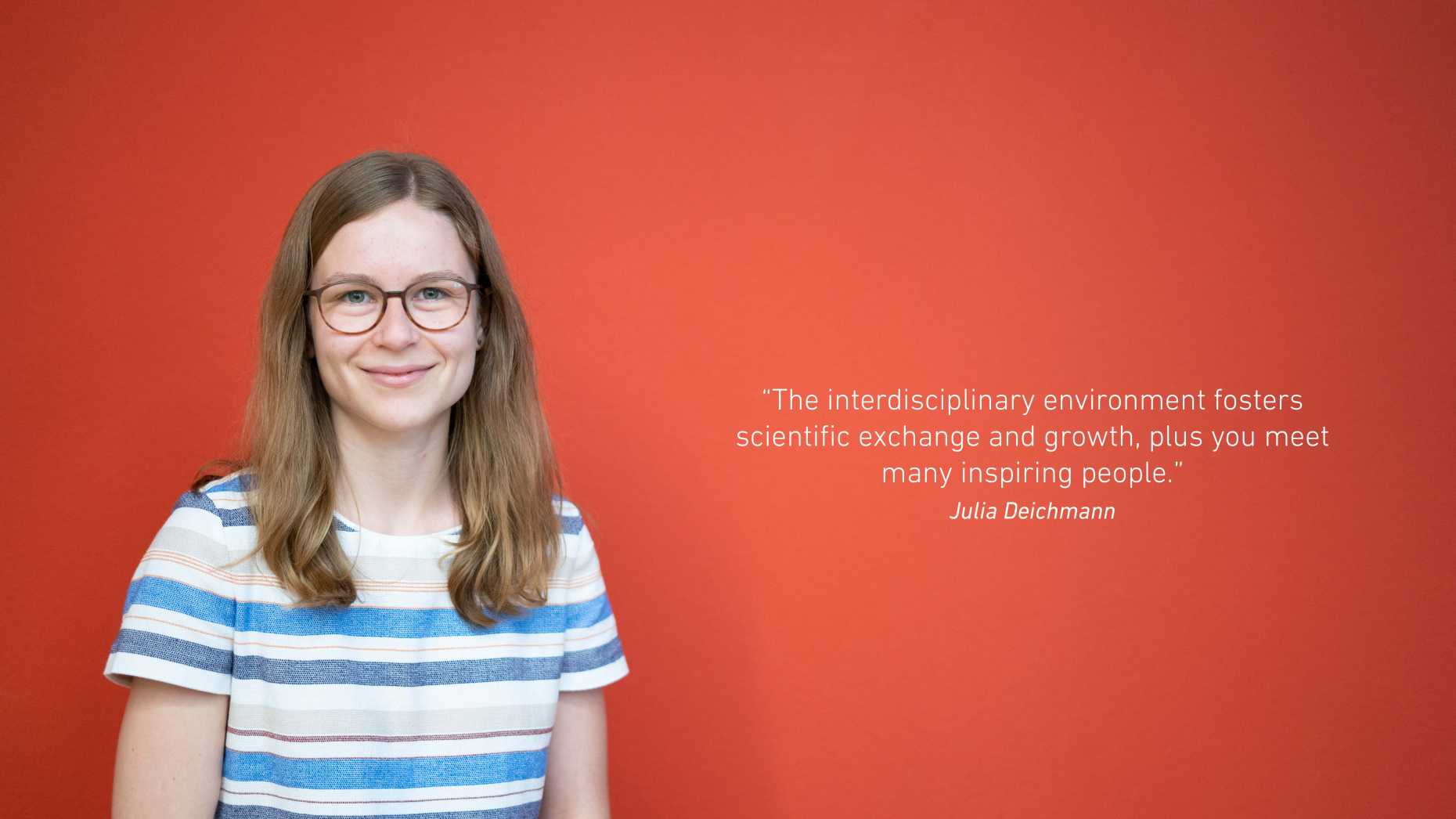
The joint doctoral programme Translational Engineering of Biological Systems of Roche's Institute of Human Biology and the ETH Zurich Department of Biosystems Science and Engineering D-BSSE offers a unique opportunity for scientific training in translational human biology and medicine. Doctoral students will conduct highly innovative and collaborative research projects and become the next generation of researchers working on scientific and technological challenges in translation.
Learn about this joint ETH-Roche doctoral programme .
The aim of this highly interdisciplinary doctoral programme of systems biological researchers of the University of Zurich and ETH in Zurich and Basel is to train students from various disciplines and departments including Computer Science and (Bio)Informatics, Biological Sciences, and Engineering to become future leaders in Systems Biology. The doctoral programme Systems Biology is associated with the Life Science Zurich Graduate School. Several research groups of the D-BSSE are part of the Systems Biology doctoral programme. Further information is provided on the doctoral programmes own website .
- Gradcracker Home
- Graduates & Research Fellowships
Graduates & Research Fellowships
Our Graduate and Postgraduate opportunities are for those who have recently graduated (0-2 years) from their degrees (Undergraduate, Masters, PhD), and want to take their first steps within industry.
Our Research and project based opportunities are available for those wanting to continue research, such as Postdoctoral Fellowships or collaboration on final year projects (such as dissertation projects).
Regulatory Affairs work cross functionally with clinical trail teams to formulate sumbmission for our products, in therapy areas across Onoclogy, Neoroscicence, Immunonogy, Infectious deasiases, & Ophthalmology.
As part of this programme you will gain insight into the work of Regulatory professionals who are responsible for providing the Regulatory input and leadership throughout the life cycle of the product, from initial first-in-human trials all the way to the point at which the product lifecycle comes to an end.
The projects you will work on are real and you will be generating genuine added value.
To broaden your experience across the development value chain you will also have the opportunity to go for a 6-month rotation in another Product Development function.
Putting patients at the forefront of everything we do, we bring our medical and scientific expertise including therapy knowledge to our customer partnerships, ensure access to our products and clinical trials and attend diligently to patient safety and customer enquiries.
In this programme you will participate in development and implementation of the global Medical Information strategy for the assigned therapeutic area/products and/or cross-portfolio activities.
You will support development and delivery of medical information, tools, resources and training so that scientifically accurate, objective and current responses to product enquiries from healthcare professionals (HCPs) and other Roche Pharma customers are consistently provided in a professional, ethical and timely manner.
The digitalisation of healthcare has created a direct link between information technology and patients. In the Roche Start IT programme, you can explore all corners of the field – maybe even surprising yourself with new interests – while making a difference to people’s lives. As a part of the programme you will get exposure to IT core processes as well as our Pharma and/or Diagnostics R&D, Commercial and Corporate Functions business.
Product Development Clinical Science specialises in late stage clinical development of molecules, conducting primarily Phase III clinical trials with a goal of characterising the efficacy and safety profile of a molecule in a specific disease area. The priority of Product Development Clinical Science is to ensure that benefit outweighs risk and we are developing medications that are serving patients with an unmet treatment need. Product Development Clinical Science are involved in key stages of a clinical trial such as study design, start-up, maintenance and final analysis phases.
The purpose of the two year programme is to provide a recent PhD or PharmD graduate (completed or due to complete their post-graduate academic studies) with the opportunity to gain specialised, in-depth training and experience in global drug development.
We combine our strengths in pharmaceuticals and diagnostics to better fit treatments to patients. When genetic differences can be identified, the efficacy and safety of medicines can be improved enormously. To this end we have a companion diagnostic strategy for every molecule we develop.
Our Personalised Healthcare (PHC) Data Science programme, take place at our Headquarters in Basel, Switzerland or at Roche UK Affiliate in Welwyn, United Kingdom for a period of 24 months.
Working side by side with other data scientists to help uncover answers to challenging clinical and business questions.The programme encourages work across innovative projects spanning multiple disease areas leveraging large amounts of healthcare data originating from EMRs (Electronic Medical Records), Disease Registries, Clinical Trials, Insurance Claims, Digital Health, Genomics and Imaging.
In the biopharmaceutical industry, each data point is part of a patient's story and their contribution to developing better medicines and understanding disease. It is our responsibility to maximise the use of this data for answering new questions and enabling larger-scale analyses for generating new insights.
You will work as part of a team to deliver data and insights about our medicines and to provide solutions that aid the collection, databasing and analysis of clinical trial data for medicines in Roche’s diverse pipeline.
Full training in systems, software and programming will be provided for all roles and you will have frequent access to senior staff that provide coaching and oversight on a day-to-day basis.
The Roche Postdoctoral Fellowship (RPF) Programme
Internally known as RPF, this programme aims to build scientific leadership by expanding and strengthening our scientific exchange and collaboration with academia.
Specifically, we offer talented Postdoctoral graduates the opportunity to join us as fellows working on a specific topic, co-mentored by people inside Roche and from external research institutions.
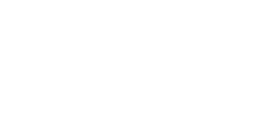
+123 4567 8910
24 fifth st, los angeles, usa, [email protected], summer 2024 coah champion: karen j. bandeen-roche, phd, ms.
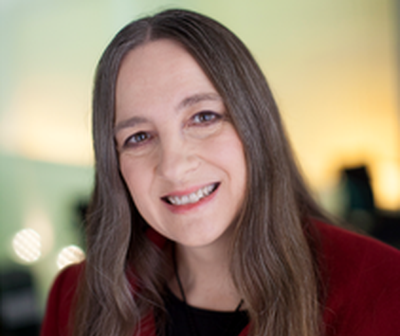
Beyond contributing to COAH as Core Faculty, Dr. Bandeen-Roche has several leadership roles at Johns Hopkins University, including serving as Co-Director of our Claude D. Pepper Older Americans Independence Center (OAIC), Data Core Leader with the Johns Hopkins Alzheimer’s Disease Research Center, Co-Director of our Frailty Science team, and Deputy Director with the Institute for Clinical & Translational Research, where she leads the Biostatistics and Research Design Program. Plus, she directs Hopkins’ Epidemiology and Biostatistics in Aging (EBA) T32 Training Program. Additionally, she serves on the Leadership Committee of the National OAIC Coordinating Center. And at one point in time, Dr. Bandeen-Roche served as the Interim Director for COAH. In fact, she has been a true champion of COAH since our beginning.
We are grateful to her for taking the time to be interviewed for this Summer 2024 COAH Champion feature story.
Tony Teano : Congratulations upon being named COAH’s Summer 2024 Champion! Please tell us about your background, education, and path to COAH.
Dr. Bandeen-Roche: I originate as a serious math geek—the kind whose leisure activities included calculating square roots by hand for fun. This continued through an undergraduate degree in mathematics—but by then I wished for a life activity with more immediate benefit for life on earth than to derive theorems. I sought an applied math field for graduate school—pursuing Operations Research and Industrial Engineering (ORIE) at Cornell, but soon discovered that statistics fascinated me a lot more than any of the other ORIE disciplines. I landed in Biostatistics because Johns Hopkins offered the most vibrant science/statistics interface—by far—among the several statistics and biostatistics faculty positions I was offered as I concluded my doctoral degree. This was 1990.
I stumbled upon aging much as I stumbled upon statistics. Scott Zeger walked me around to meet his research collaborators soon after I arrived at JHU: Two of them were Sheila West and Linda Fried. I was attracted to collaborate with them, first, because their personalities were inviting and their work fit within my interest of complex measurement problems, but before long, I was compelled by the vast opportunity aging science offered to benefit people. Once lit, this fire has never dimmed.
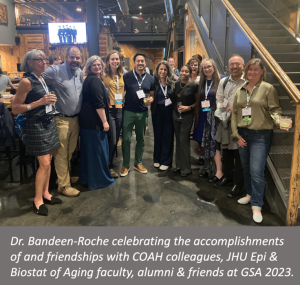
COAH is important in so many ways. It was the University’s first stake—and remains one of the most important—that it’s worth building an interdivisional presence on aging. It leverages the synergies that only can arise in this way. It has an important visioning role for the most important research we can accomplish together, and a key role in establishing bridges and communication pathways across units, disciplines and programs. May it flourish in all these regards and more.
Tony Teano: The purpose of this interview is to learn more about you both as a professional as well as a person. Let’s start with your research. What papers are you most known for or most proud of?
Dr. Bandeen-Roche: I would say there are three papers. In statistics, it’s my paper demonstrating that the specific distributional assumptions of latent variable models can be checked, and how to do it. This debunked a major, mistaken common wisdom about these models. Here’s the reference: Bandeen-Roche, K., Miglioretti, D. L., Zeger, S. L., & Rathouz, P. J. (1997). Latent Variable Regression for Multiple Discrete Outcomes. Journal of the American Statistical Association , 92 (440), 1375–1386. https://doi.org/10.2307/2965407 A more accessible link can be accessed with Johns Hopkins credentials here .
Gerontologically, there are two papers addressing frailty. Here’s the reference for the first: Bandeen-Roche K, Xue QL, Ferrucci L, Walston J, Guralnik JM, Chaves P, Zeger SL, Fried LP. Phenotype of frailty: characterization in the women’s health and aging studies. J Gerontol A Biol Sci Med Sci. 2006 Mar;61(3):262-6. doi: 10.1093/gerona/61.3.262 . PMID: 16567375. This was the first paper, to my knowledge, to attempt a construct validation of frailty (as opposed to content and predictive validation). Physical frailty phenotype measures were shown to behave consistently with a critical mass syndrome, as theorized, using latent class analysis. In the years since, construct validation of frailty measures has become more common. These don’t always agree with my substantive findings, but that’s fine—to pursue this important type of investigation is the thing!
The second gerontological paper to which I am referring established nationally representative estimates of frailty prevalence in the US – an important benchmark in its own right. It also evidenced stunningly large disparities in frailty prevalence for historically under-represented racial/ethnic groups, laying foundation for further research to better understand why and how this might be addressed. Here’s its reference: Bandeen-Roche K, Seplaki CL, Huang J, Buta B, Kalyani RR, Varadhan R, Xue QL, Walston JD, Kasper JD. Frailty in Older Adults: A Nationally Representative Profile in the United States. J Gerontol A Biol Sci Med Sci. 2015 Nov;70(11):1427-34. doi: 10.1093/gerona/glv133 . Epub 2015 Aug 21. PMID: 26297656; PMCID: PMC4723664.
Tony Teano: You recently stepped down from your role as Chair of BSPH/Biostatistics. What were you proud to have accomplished during your time as chair?
Dr. Bandeen-Roche: I’m proud to have helped the department navigate a difficult time as I was taking on the role and then to strengthen its promise to advance science through statistics by broadening its tent of expertise. We now span the whole spectrum from theory to practice and from modeling to data science both as a faculty and in our educational programs. I think we achieved it while maintaining and growing respect for one another. We also have upheld a culture of mutual caring and supportiveness that we believe fosters risk taking and creativity, in addition to enhancing life quality.
Tony Teano: What have you been doing with your additional time since you stepped down?
Dr. Bandeen-Roche: I began by teaching more than usual—rewarding, but not the optimal choice for transitioning. Since my teaching for the year ended, though, I’ve been spending more time in research—everything from thinking to planning to analyzing data, and loving it. The email and meeting time has dropped significantly, and I celebrate this!
Tony Teano: These are major transitions. How do you navigate and prepare for change, either at work or in life in general?
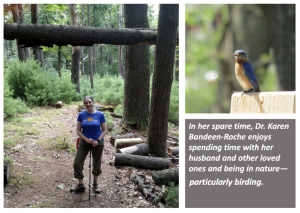
One more specific answer is to create reserve (and hence resilience) to meet change—by ensuring personnel and resource buffers, for example. Another is to keep one’s (personal or organizational) portfolio diversified. Yet one more is to emphasize assets above specific foci. In biostatistics, for example, data science is huge now, but ten years ago it was genomics, and another 10 years before that it was computational statistics, and before that it was modeling for complex data. 10 years from now it may be something else entirely! As long as one has faculty who are intellectually curious, broadly knowledgeable about fundamentals of statistical ideas and practice, span the gamut of strengths from basic knowledge to detailed application, and like each other/work well together, we can pivot to take on whatever comes next.
Tony Teano: Work/Life balance is related to creating reserve. What you do to unwind, such as hobbies or interests you enjoy? What renews you?
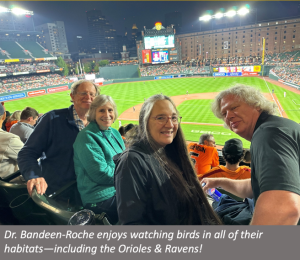
Tony Teano: Tell us more about what in pop culture you escape into—a few of your favorite things.
Dr. Bandeen-Roche: Here’s the short list.
Favorite performer: Rush (the Canadian rock band). The Tragically Hip (another Canadian rock band) is a close second.
Favorite TV show: Probably “Scrubs” for a long-running series; Slings and Arrows (three-year mini-series about a Shakespeare Festival troupe) overall.
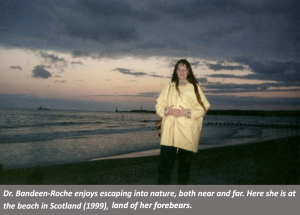
Tony Teano: If you could go anywhere in the world, what place would you like to visit and why?
Dr. Bandeen-Roche: Scotland; land of my forebears. Best trip of my life was here (1999), and I’d love to return. Never yet seen but bucket list includes the Apostle Islands (northern Wisconsin) and UP of Michigan, and Nova Scotia.
Tony Teano: As we wrap up this interview, please share the best advice, personal or professional, you have ever received.
Dr. Bandeen-Roche: Two items:
(1) From my PhD advisor, Dr. David Ruppert: Remember that it’s a marketplace of ideas. It doesn’t matter how good your ideas are if you can’t sell them to the key stakeholders (scientific community… institutional leaders… policymakers… the public).
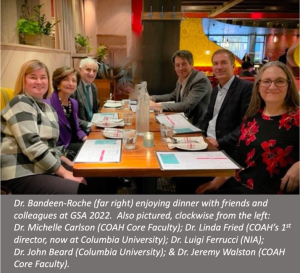
By: Anthony Teano, MLA Communications Specialist
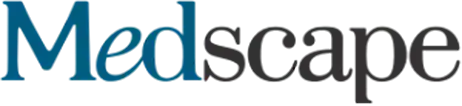
Faculty and Disclosures
Medscape, LLC requires every individual in a position to control educational content to disclose all financial relationships with ineligible companies that have occurred within the past 24 months. Ineligible companies are organizations whose primary business is producing, marketing, selling, re-selling, or distributing healthcare products used by or on patients.
All relevant financial relationships for anyone with the ability to control the content of this educational activity are listed below and have been mitigated. Others involved in the planning of this activity have no relevant financial relationships.
Disclosures for additional planners can be found here .
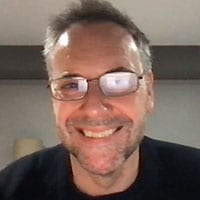
Craig Ritchie, MBChB, PhD, MSc, FRCP(Edin), MRCPsych
Chief Executive Officer Scottish Brain Sciences Edinburgh, Scotland
Craig Ritchie, MBChB, PhD, MSc, FRCP(Edin), MRCPsych, has the following relevant financial relationships: Consultant or advisor for: Actinogen; Biogen; Eisai Co., Ltd.; Lilly; Merck Sharp & Dohme, Corp.; Roche Other: Honoraria: Eisai Co., Ltd.; Roche
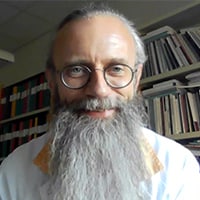
Henrik Zetterberg, MD, PhD
Professor of Neurochemistry University of Gothenburg Gothenburg, Sweden
Henrik Zetterberg, MD, PhD, has the following relevant financial relationships: Consultant or advisor for: AbbVie Inc.; Acumen; Alector; Alzinova; ALZPath; Annexon; Apellis; Artery Therapeutics; AZTherapies; Cognito Therapeutics; CogRx; Denali; Eisai; Merry Life Biomedical Co., Ltd.; Nervgen; Novo Nordisk; Optoceutics; Passage Bio; Pinteon Therapeutics; Prothena; Red Abbrey Labs; reMYND; Roche; Samumed; Siemens Healthcare Diagnostics Inc.; Triplet Therapeutics; Wave
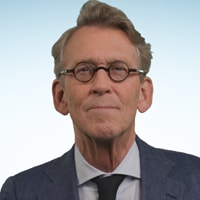
Philip Scheltens, MD, PhD
Professor Emeritus Amsterdam University Medical Center Partner EQT Life Science Head Dementia Fund Amsterdam, The Netherlands
Philip Scheltens, MD, PhD, has the following relevant financial relationships: Consultant or advisor for: Novo Nordisk
Frances McFarland, PhD, MA
Medical Education Director, Medscape, LLC
Frances McFarland, PhD, MA, has no relevant financial relationships.
Compliance Reviewer
Leigh schmidt, msn, rn, cne, chcp.
Associate Director, Accreditation and Compliance, Medscape Education
Leigh Schmidt, MSN, RN, CNE, CHCP, has no relevant financial relationships.
Peer Reviewer
This activity has been peer reviewed and the reviewer has no relevant financial relationships.

CME / ABIM MOC
International perspectives on biomarker testing in alzheimer’s disease diagnosis.
- Authors: Craig Ritchie, MBChB, PhD, MSc, FRCP(Edin), MRCPsych; Henrik Zetterberg, MD, PhD; Philip Scheltens, MD, PhD
CME / ABIM MOC Released: 8/30/2024
Valid for credit through: 8/30/2025 , 11:59 PM EST
Credits Available
Physicians - maximum of 1.00 AMA PRA Category 1 Credit(s)™
ABIM Diplomates - maximum of 1.00 ABIM MOC points
You Are Eligible For
- Letter of Completion
- ABIM MOC points
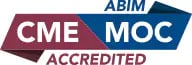
Target Audience and Goal Statement
This activity is intended for neurologists, primary care physicians, and geriatricians (excluding the United Kingdom).
The goal of this activity is for learners to be better able to use and interpret biomarker testing for diagnosing early stages of AD.
Upon completion of this activity, participants will:
- Identify which patients may benefit most from the use of biomarker testing
- Utilize cerebrospinal fluid biomarker tests in patients with cognitive impairment to facilitate timely and differential diagnosis
- Learn how to communicate with patients about biomarker testing
Disclosures
Accreditation statements.
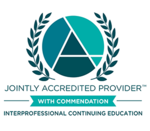
For Physicians
Medscape, LLC designates this enduring material for a maximum of 1.0 AMA PRA Category 1 Credit(s)™ . Physicians should claim only the credit commensurate with the extent of their participation in the activity.
Successful completion of this CME activity, which includes participation in the evaluation component, enables the participant to earn up to 1.0 MOC points in the American Board of Internal Medicine's (ABIM) Maintenance of Certification (MOC) program. Participants will earn MOC points equivalent to the amount of CME credits claimed for the activity. It is the CME activity provider's responsibility to submit participant completion information to ACCME for the purpose of granting ABIM MOC credit. Aggregate participant data will be shared with commercial supporters of this activity.
The European Union of Medical Specialists (UEMS)-European Accreditation Council for Continuing Medical Education (EACCME) has an agreement of mutual recognition of continuing medical education (CME) credit with the American Medical Association (AMA). European physicians interested in converting AMA PRA Category 1 credit ™ into European CME credit (ECMEC) should contact the UEMS (www.uems.eu).
College of Family Physicians of Canada Mainpro+® participants may claim certified credits for any AMA PRA Category 1 credit(s) ™, up to a maximum of 50 credits per five-year cycle. Any additional credits are eligible as non-certified credits. College of Family Physicians of Canada (CFPC) members must log into Mainpro+® to claim this activity.
Through an agreement between the Accreditation Council for Continuing Medical Education and the Royal College of Physicians and Surgeons of Canada, medical practitioners participating in the Royal College MOC Program may record completion of accredited activities registered under the ACCME’s “CME in Support of MOC” program in Section 3 of the Royal College’s MOC Program.
Contact This Provider
For questions regarding the content of this activity, contact the accredited provider for this CME/CE activity noted above. For technical assistance, contact [email protected]
Instructions for Participation and Credit
There are no fees for participating in or receiving credit for this online educational activity. For information on applicability and acceptance of continuing education credit for this activity, please consult your professional licensing board. This activity is designed to be completed within the time designated on the title page; physicians should claim only those credits that reflect the time actually spent in the activity. To successfully earn credit, participants must complete the activity online during the valid credit period that is noted on the title page. To receive AMA PRA Category 1 Credit™ , you must receive a minimum score of 70% on the post-test. Follow these steps to earn CME/CE credit*:
- Read about the target audience, learning objectives, and author disclosures.
- Study the educational content online or print it out.
- Online, choose the best answer to each test question. To receive a certificate, you must receive a passing score as designated at the top of the test. We encourage you to complete the Activity Evaluation to provide feedback for future programming.
You may now view or print the certificate from your CME/CE Tracker. You may print the certificate, but you cannot alter it. Credits will be tallied in your CME/CE Tracker and archived for 6 years; at any point within this time period, you can print out the tally as well as the certificates from the CME/CE Tracker. *The credit that you receive is based on your user profile.
- Commento dell'esperto in italiano
Il presente commento fa parte di un'attività didattica più ampia. Dopo la partecipazione, si prega di tornare a International Perspectives on Biomarker Testing in Alzheimer’s Disease Diagnosis per accedere all'intero contenuto didattico correlato.
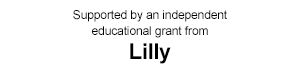
- Activity Transcript
Expert Commentary
- Commentaire d'expert en français
Medscape Education © 2024 Medscape, LLC
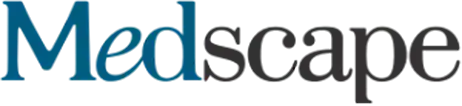
Thank you for visiting nature.com. You are using a browser version with limited support for CSS. To obtain the best experience, we recommend you use a more up to date browser (or turn off compatibility mode in Internet Explorer). In the meantime, to ensure continued support, we are displaying the site without styles and JavaScript.
- View all journals
- Explore content
- About the journal
- Publish with us
- Sign up for alerts
- Open access
- Published: 29 August 2024
Adipocyte deletion of the oxygen-sensor PHD2 sustains elevated energy expenditure at thermoneutrality
- Rongling Wang ORCID: orcid.org/0000-0001-8719-938X 1 ,
- Mario Gomez Salazar 1 ,
- Iris Pruñonosa Cervera 1 ,
- Amanda Coutts ORCID: orcid.org/0000-0002-5005-1864 2 ,
- Karen French 1 ,
- Marlene Magalhaes Pinto 1 ,
- Sabrina Gohlke 3 ,
- Ruben García-Martín ORCID: orcid.org/0000-0003-2049-4960 4 ,
- Matthias Blüher ORCID: orcid.org/0000-0003-0208-2065 5 ,
- Christopher J. Schofield ORCID: orcid.org/0000-0002-0290-6565 6 ,
- Ioannis Kourtzelis ORCID: orcid.org/0000-0003-3006-8885 7 ,
- Roland H. Stimson ORCID: orcid.org/0000-0002-9002-6188 1 ,
- Cécile Bénézech 1 ,
- Mark Christian ORCID: orcid.org/0000-0002-1616-4179 2 ,
- Tim J. Schulz ORCID: orcid.org/0000-0002-8413-3972 3 , 8 ,
- Elias F. Gudmundsson ORCID: orcid.org/0000-0002-7661-4872 9 ,
- Lori L. Jennings ORCID: orcid.org/0000-0001-5130-8417 10 ,
- Vilmundur G. Gudnason ORCID: orcid.org/0000-0001-5696-0084 9 , 11 ,
- Triantafyllos Chavakis ORCID: orcid.org/0000-0002-1869-5141 1 , 8 , 12 , 13 ,
- Nicholas M. Morton 1 , 2 ,
- Valur Emilsson ORCID: orcid.org/0000-0001-9982-0524 9 , 11 &
- Zoi Michailidou ORCID: orcid.org/0000-0003-0085-4842 1 , 2
Nature Communications volume 15 , Article number: 7483 ( 2024 ) Cite this article
2 Altmetric
Metrics details
- Experimental models of disease
Enhancing thermogenic brown adipose tissue (BAT) function is a promising therapeutic strategy for metabolic disease. However, predominantly thermoneutral modern human living conditions deactivate BAT. We demonstrate that selective adipocyte deficiency of the oxygen-sensor HIF-prolyl hydroxylase (PHD2) gene overcomes BAT dormancy at thermoneutrality. Adipocyte-PHD2-deficient mice maintain higher energy expenditure having greater BAT thermogenic capacity. In human and murine adipocytes, a PHD inhibitor increases Ucp1 levels. In murine brown adipocytes, antagonising the major PHD2 target, hypoxia-inducible factor-(HIF)−2a abolishes Ucp1 that cannot be rescued by PHD inhibition. Mechanistically, PHD2 deficiency leads to HIF2 stabilisation and binding of HIF2 to the Ucp1 promoter, thus enhancing its expression in brown adipocytes. Serum proteomics analysis of 5457 participants in the deeply phenotyped Age, Gene and Environment Study reveal that serum PHD2 associates with increased risk of metabolic disease. Here we show that adipose-PHD2-inhibition is a therapeutic strategy for metabolic disease and identify serum PHD2 as a disease biomarker.
Similar content being viewed by others
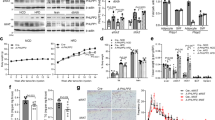
Adipocyte PHLPP2 inhibition prevents obesity-induced fatty liver
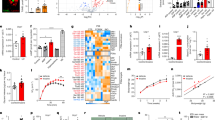
Apoptotic brown adipocytes enhance energy expenditure via extracellular inosine
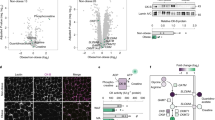
Impaired phosphocreatine metabolism in white adipocytes promotes inflammation
Introduction.
Several elegant studies have identified approaches to activate thermogenic brown adipose tissue (BAT) and enhance metabolism 1 , 2 , 3 . However, the majority of these studies were conducted on rodents at ambient temperature (19–21 °C), a condition where BAT is already active 4 , 5 , 6 . Consequently, the human translation of these studies is questionable, because humans predominantly live in thermoneutral conditions by using clothing or other means to maintain thermal homeostasis 7 . One highly upregulated gene in adipose tissue during cold exposure of rodents is the hypoxia inducible transcription factor (HIF)-2a 8 , suggesting that the hypoxia signalling pathway is involved in BAT activation. The oxygen-sensing HIF-prolyl hydroxylases (PHDs) are central regulators of the hypoxic response 9 , 10 . PHD2 is reported to be the most important oxygen sensor and is a negative regulator of HIF-a activity in normoxia 9 , 10 . PHD2 hydroxylates key proline residues on HIF-a isoforms in normoxia, leading to their proteasomal degradation 9 , 10 .
PHD inhibitors are of increasing medical significance and have been approved for the treatment of renal anaemia in chronic kidney disease (CKD) 11 in China and Japan 12 , 13 and very recently in Europe by the European Medicine Agency 14 . Previously, we and others, have shown that models of whole-body or adipose selective deletion of the dominant, ubiquitously expressed PHD2 isoform, induced protection from metabolic disease, in part by reducing plasma lipid levels 15 , 16 . Here we hypothesized that the lipid-lowering effects of PHD2 deficiency is due to enhanced BAT thermogenesis. We tested this hypothesis, using an adipose tissue (white and brown) selective genetic mouse model of PHD2 deletion ( P2 KO ad ) under the more human translationally aligned thermoneutral housing conditions. To address the relevance of PHD2 in human metabolic disease traits, we interrogated the unique large-scale serum proteomics and deep metabolic and anthropometric phenotyping data from the Age/Gene Environment Susceptibility study (Icelandic Heart Association) 17 , 18 .
Loss of adipocyte Phd2 sustains energy expenditure at thermoneutrality in both male and female mice
Housing of mice at thermoneutrality (TN; ~28–30 °C) results in a marked decrease in energy expenditure and loss of BAT function 19 , 20 . This aligns rodent metabolism more closely with that of humans, providing a better preclinical modelling system 5 , 6 , 7 , 19 , 20 , 21 . We tested whether the metabolic advantage observed with adipose-specific PHD2 deletion previously 16 , 22 , was retained at TN (28–29 °C), thus indicating an effect independent from, the tonic, metabolically protective activation of BAT function found at room temperature (RT;19–21 °C) in mice.
Adiponectin– Cre 23 mice were crossed with PHD2 fl/fl mice 16 to delete PHD2 (referred as P2 KO ad ) in white (mean Phd2 mRNA levels ±SEM: Control:1.8 ± 0.23 arb. units ( n = 5) vs P2 KO ad : 0.14 ± 0.14 arb. units ( n = 6), P < 0.0001) and brown adipocytes (Supplemental Fig. 1A ). This approach targets all white (WAT), beige and BAT adipocytes 24 and thus reflects a generalised adipose tissue deletion not restricted only to the rodent-specific BAT depot. P2 KO ad mice had >85% reduction in Phd2 mRNA levels compared to control littermates, and consequently elevated levels of the validated HIF-target genes, Phd3 (fourfold, P = 0.026) and Vegfa (1.6-fold, P = 0.018) in BAT (Supplemental Fig. 1A ). Vegfa mRNA levels in inguinal white adipose tissue (iWAT, mean ± SEM: Control: 0.38 ± 0.07 arb. units ( n = 5) vs P2 KO ad : 0.73 ± 0.12 arb. units ( n = 6), P = 0.045) of P2 KO ad mice were higher compared to control littermates. In P2 KO ad BAT, HIF-1a and HIF2a levels were stabilised, as expected (Supplemental Fig. 1B ), irrespective of oxygen levels in the tissue. These results are consistent with enhanced vascularization in the adipose tissues in this model as we previously showed in WAT irrespective of ambient temperature 16 . P2 KO ad mice initially housed at room temperature (RT; 20–21 °C) were then examined at acute thermoneutrality (TN; 28–29 °C, 7 days). Unexpectedly, despite similar body weights (Fig. 1A ), P2 KO ad mice had relatively higher energy expenditure (EE), especially in the dark phase (Fig. 1B ) than control littermates. Overall, the P2 KO ad mice showed a skew towards lipid utilization (lower RER) and this was statistically significant at room temperature (during the whole day) and in the dark phase at thermoneutrality (Fig. 1C ). There were no differences in physical activity levels (Fig. 1D ). Presumably to compensate for their higher EE at TN, P2 KO ad mice exhibited higher food intake (Fig. 1E ) compared to their littermate controls. Switching male mice from RT to TN, led to increased fat mass deposition in both genotypes (Fig. 1F ), such that at thermoneutrality, body gross composition, determined by TD-NMR, was similar between the genotypes (lean and fat mass, Fig. 1F ). However, the control littermate mice lost BAT mass at TN, whereas the P2 KO ad mice, maintained BAT mass (Fig. 1G ) and had significantly greater BAT mass (Fig. 1G ). White adipose depots (Fig. 1H ) and liver mass (mean ± SEM: Control:1.33 ± 0.17g vs P2 KO ad : 0.42 ± 0.20 g ( n = 7), P = 0.36) were comparable between genotypes.

A P2 KO ad (KO, red) mice housed at RT (21 °C, n = 15) or acutely switched to TN (28–29 °C, 7 days) sustain similar body weight (BW) to control (C, blue, n = 13) littermates. B P2 KO ad mice have higher energy expenditure (EE) at TN ( n = 6/group) and C lower respiratory exchange ratio (RER, RT: n = 5/group; TN: n = 11/group). D Similar activity levels in both genotypes (RT: n = 5/group; TN: n = 11/group). E KO (RT: n = 6; TN: n = 14) eat more than C (RT: n = 7; TN: n = 14) mice at TN. F Despite similar fat and lean mass in both genotypes measured by time-domain (TD) NMR (RT: n = 8/group; TN control: n = 10; TN KO: n = 11), G P2 KO ad mice have bigger BAT mass (C RT: n = 8; KO RT: n = 11; C TN: n = 7; KO TN: n = 7) but H similar WAT mass (RT: n = 5/group; TN: n = 7/group), biological replicates. Data are presented as mean + /− SEM. * P < 0.05, ** P < 0.01 by Student t test (two-tailed). For EE, ANCOVA was performed with BW as covariance. Source data are provided as a Source Data file.
Energy homeostasis differs between sexes. Rodent and human studies suggest that females have higher capacity to increase mass and thermogenic activity of adipose tissue 25 , 26 . To test whether adipose- Phd2 deletion would be advantageous in both sexes as a target to increase energy expenditure, we set paired experiments, switching from RT to TN housing, in female P2 KO ad ( Egln1 , 90% knock down achieved in female adipose tissue, Supplemental Fig. 1C ) and control littermates. As in males, P2 KO ad female mice had similar body weights at RT and TN (Fig. 2A ) to control littermates but retained higher energy expenditure at thermoneutrality (Fig. 2B ), but no significant differences in substrate utilization (Fig. 2C ) or physical activity (Fig. 2D ). Switching to thermoneutrality led to reduced food intake in the control female mice (Fig. 2E ). Female P2 KO ad mice retained similar food intake in both temperatures (Fig. 2E ), in contrast to P2 KO ad males that increased food intake at TN. Body composition in females (fat and lean mass) measured by TD-NMR was unchanged in thermoneutrality (Fig. 2F ), in contrast to males (Fig. 1F ). However, in females, BAT mass was specifically increased in both genotypes (Fig. 2G ) with no changes observed in WAT mass (Fig. 2H ) or other metabolic tissues like the liver (mean ± SEM: Control:1.2 ± 0.09 g vs P2 KO ad : 1.3 ± 0.04 g ( n = 7–9), P = 0.37). Taken together these data show that both male and female mice lacking adipose- Phd2 sustain higher energy expenditure at thermoneutrality.

A P2 KO ad (KO, purple) mice housed at 20–21 °C (RT, n = 9) when acutely switched to 28–29 °C (TN, 7 days) sustain similar body weight (BW) to control (C, black, n = 7) littermates but B have higher energy expenditure ( n = 6/group) . C Similar respiratory exchange ratio ( n = 6/group) and D activity levels ( n = 6/group) . E Control mice reduce, but KO sustain food intake at TN ( n = 6/group). F Similar fat and lean mass measured by TD-NMR (C: n = 7 and KO: n = 9) in both genotypes . G Similar BAT mass (*** indicates comparisons of RT with TN; RT C: n = 8, KO: n = 6 and TN: n = 6/group) and H WAT mass (i inguinal, p parametrial, m mesenteric, RT: n = 3/group; TN C: n = 7 and KO: n = 9) in both genotypes. n indicates biological replicates. Data are presented as mean + /− SEM. * P < 0.05, ** P < 0.01, *** P < 0.001 by paired ( A – F ) or unpaired ( G , H ) Student t test (two-tailed). For EE, ANCOVA was performed with BW as covariance. Source data are provided as a Source Data file.
Loss of adipose- Phd2 facilitates better metabolic health at thermoneutrality
Poor metabolic health is associated with low BAT activity 27 . Metabolically, the main effect of adipose- Phd2 deletion was on plasma lipids in both sexes. Specifically, P2 KO ad mice had significantly lower circulating glycerol and non-esterified fatty acids (NEFA) levels compared to control mice (Fig. 3A–D ) at RT. Male P2KO ad mice retained lower levels of plasma lipids when housed at TN (Fig. 3A, C ). Glucose levels were not affected by genotype or housing temperature (Fig. 3E, F ). Insulin levels were similar in control and P2KO ad male mice (Fig. 3G ). However, female P2 KO ad mice had lower plasma insulin levels compared to control littermates in both RT and TN (Fig. 3H ), despite similar glucose levels (Fig. 3F ).
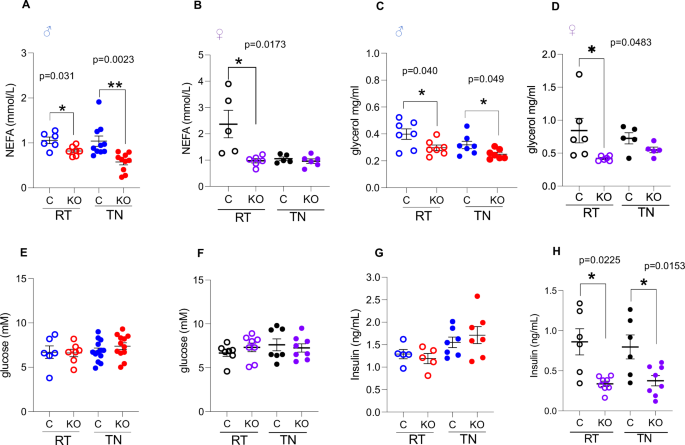
A , B Plasma non-esterified fatty acids, C , D glycerol, E , F glucose and G , H insulin levels. Males, NEFA: control RT n = 6, TN n = 10, KO RT n = 7 TN n = 10, glycerol: n = 7/group, glucose: control RT n = 6 TN n = 13, KO RT n = 7, TN n = 12, insulin: RT n = 5/group, TN n = 7/group). Females, NEFA: control n = 5, KO n = 6; glycerol: control n = 5, KO n = 5; glucose: control n = 7, KO n = 8; insulin: control n = 6, KO n = 8). Data are presented as mean + /− SEM. N represents biological replicates. * P < 0.05, ** P < 0.01 by Student t test (two-tailed). Source data are provided as a Source Data file.
Loss of adipocyte Phd2 mediates retention of BAT function at thermoneutrality
Although energy expenditure was increased in both male and female mice, and irrespective of genotype, females retained BAT mass at thermoneutrality, only male P2 KO ad mice had significantly greater BAT mass than their control littermates. At a molecular level, as expected, switching mice from RT to TN, decreased mRNA levels of the thermogenic gene Ucp1 in BAT (Supplemental Fig. 1D ). However, deletion of adipocyte Phd2 led to sustained (and higher compared to control mice) Ucp1 mRNA levels and the BAT-activating adrenergic receptor, Adrb3 , in BAT (Supplemental Fig. 1E ) and in iWAT (Supplemental Fig. 1F, G ) in male mice at thermoneutrality. There were sex-differences on this response, as female mice retained only higher BAT Adrb3 levels (Supplemental Fig. 1E ) in both housing conditions but only sustained significantly higher Ucp1 mRNA levels in iWAT (Supplemental Fig. 1F ) during TN. As the effects were more pronounced and consistent in the BAT and iWAT of male mice, we continued further investigations in male mice.
The main characteristic during BAT activation in cold exposure or β-adrenergic stimulation is BAT hyperplasia and/or hypertrophy 28 , 29 . To investigate the cellular basis for the unexpected resistance of BAT to regress at TN in male P2 KO ad mice, we performed histological analysis. P2 KO ad mice had bigger adipocytes, in the range of 450–1000 μm 2 , but similar brown adipocyte numbers to control mice (Fig. 4A, B ), suggesting hypertrophy in BAT. To provide additional insights into BAT function, we quantified UCP1+ cells at thermoneutrality using antibody staining as a proxy for functional BAT. P2 KO ad mice exhibited more UCP1 expressing cells (Fig. 4C, D and Supplemental Fig. 2A ) in BAT than control littermates and more ki67+ cells (Fig. 4C, D and Supplemental Fig. 2B ), suggesting higher proliferation rates. Higher protein UCP1 levels in BAT in P2 KO ad mice were confirmed by western blot analysis (Supplemental Fig. 2D ).
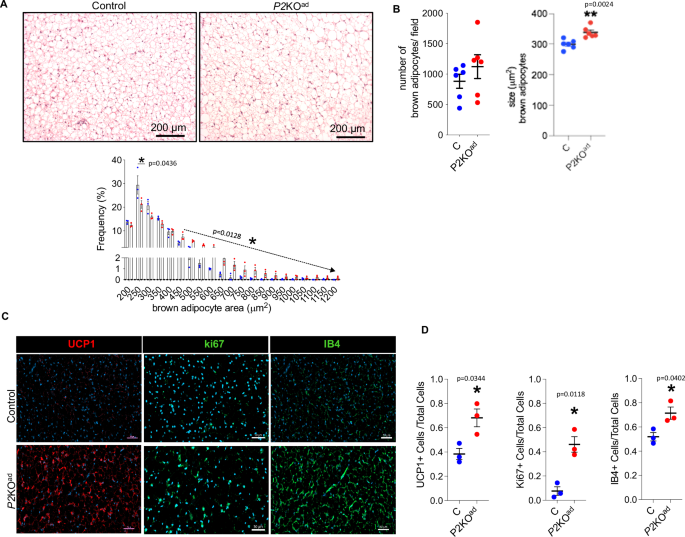
A H&E staining of BAT and frequency distribution of small and large adipocytes ( n = 3/group, biological replicates). B Quantification graphs of number and size of brown adipocytes in P2 KO ad (red) and control littermates (blue). The adipocyte number/size is per microscopic field (total of 6 fields per animal analysed, n = 6/group, biological replicates). C Immunofluorescence images (scale bars 50 µm) and D quantification graphs of BAT stained for UCP1+ (red) and ki67+ and IB4+ (green) cells at TN ( n = 3/group, biological replicates). Nuclei stained with DAPI (blue). Data are presented as mean + /− SEM. * P < 0.05, by Student t test (two-tailed). A Source data are provided as a Source Data file.
Previously, we have shown that deletion of Phd2 leads to better WAT vascularization (increased Vegfa mRNA/CD31 immunoreactivity) 16 at ambient housing conditions (21 °C). We confirmed here that Vegfa mRNA levels remain higher at thermoneutrality in P2 KO ad mice BAT (Supplemental Fig. 1A ). Immunofluorescence for isolectin IB4, a vessel marker, also confirmed more extensive BAT vascularization in P2 KO ad mice (Fig. 4C, D and Supplemental Fig. 2C ). Taken together this data suggest, the loss of adipose- Phd2 permits better BAT remodelling at thermoneutrality.
Loss of adipocyte- Phd2 leads to higher maximal response to β3-adrenergic stimulation
To further examine BAT/beige function we performed a test of pharmacological BAT activation using a β3-adrenergic receptor agonist (CL 316,243; CL) in a paired experiment. P2 KO ad mice had a higher response to adrenergic stimulation when examined at 28 °C (Fig. 5 ). Despite similar body weights (C vs KO: 27.5 ± 0.4 g vs 27.9 ± 0.5 g, P = 0.47), P2 KO ad mice had significantly higher energy expenditure immediately post CL administration that was sustained throughout the rest of the day (Fig. 5A ), and this was not due to higher activity levels (Fig. 5B ). CL induced a switch to utilising lipids in both genotypes with a significantly lower RER in P2 KO ad mice (Fig. 5C ). Additionally, CL-induced significantly higher plasma NEFA release in the P2 KO ad mice (Fig. 5D ) and higher Adrb3 and Ucp1 mRNA expression in both BAT and iWAT (Fig. 5E ). Importantly, this was confirmed at the protein level, as UCP1 was higher in the P2 KO ad BAT after CL stimulation (Fig. 5F ).
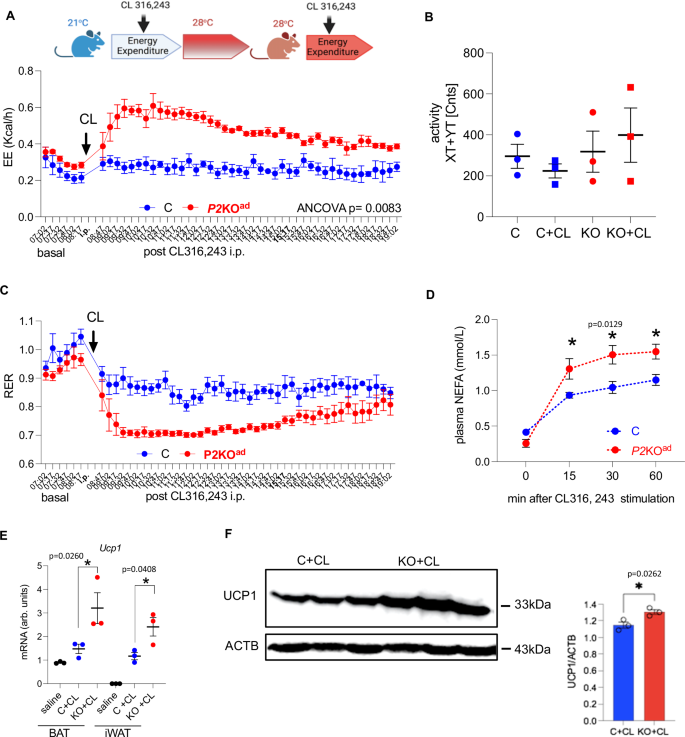
A P2 KO ad (KO, red, n = 3) and control (C, blue, n = 3) mice were challenged with CL316,243 i.p. (CL; 1 mg/g BW) at RT (21 °C). Then switched to TN housing (28 °C) and administered second CL i.p. CL given under TN conditions led to higher energy expenditure in P2 KO ad mice, B similar activity levels in both genotypes, C lower respiratory exchange ratio in P2 KO ad mice, D higher sensitivity to CL-induced NEFA release in P2 KO ad mice, E higher CL-induced Ucp1 mRNA expression in BAT and iWAT in P2KO ad mice and F higher CL-induced UCP1 protein levels in P2 KO ad BAT. Data are presented as mean + /- SEM. * P < 0.05, ** P < 0.01 by Student t test (two-tailed) or one-way ANOVA ( E ). For EE, ANCOVA was performed with body weight as co-variant. A Experimental design image was created with BioRender.com released under a Creative Commons Attribution-Non Commercial-NoDerivs 4.0 International license. Source data and uncropped blots are provided as a Source Data file.
Loss of adipose- Phd2 retains higher EE after diet induced obesity at thermoneutrality
High fat diet (HFD) feeding and thermoneutrality independently reduce energy expenditure and the thermogenic gene expression program in BAT 20 . Feeding HFD at thermoneutrality, as a more translationally aligned model for diet-induced obesity (DIO), further suppresses energy expenditure and BAT function 20 . To test whether the higher energy expenditure at TN seen in P2 KO ad can be sustained during HFD, we fed male mice HFD for 8-weeks while housed at TN. Despite similar body weights (Fig. 6A ) and body weight gain (Supplemental Fig. 3A ) after 8 weeks of HFD in both genotypes, P2 KO ad mice retained higher energy expenditure during HFD compared to their control littermates (Fig. 6B ). No significant differences were detected in substrate utilization (Fig. 6C ) or physical activity (Fig. 6D ) between control and P2 KO ad mice. Unfortunately, we could not accurately record food intake in this experiment as most mice shredded their HFD. Analysis of the food intake data from the first couple of days of HFD did not show any significant differences (Supplemental Fig. 3B ). Fat mass gain after HFD (Fig. 6E ), including fresh WAT (Fig. 6F ) and BAT (Fig. 6G ) weight, change in lean mass (Supplemental Fig. 3C ) and liver weights (Supplemental Fig. 3D ) were similar in both genotypes. Although their glucose levels were similar (Fig. 6H ), P2 KO ad mice retained lower plasma NEFA (Fig. 6I ). Fasting plasma insulin levels were similar, but in the fed state, the P2 KO ad mice did not significantly increase insulin levels in contrast to control littermates (Fig. 6J ).
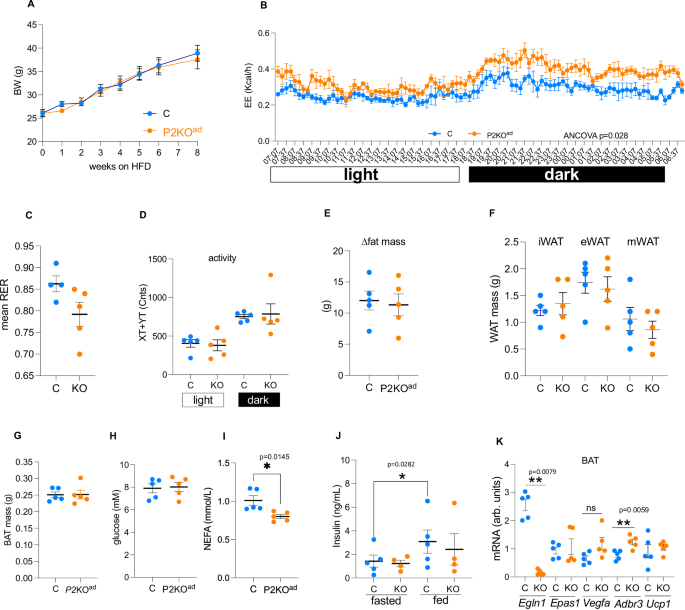
A P2 KO ad (orange, n = 5) and control (C, light blue, n = 5) mice were fed high fat diet (58% kcal from fat) while housed at TN (28–29 °C) for 8 weeks had similar body weight gain. B Higher energy expenditure in high fat fed P2 KO ad at TN. C Similar respiratory exchange ratio, D activity levels , E fat mass gain by TD-NMR , F WAT and G BAT weights after high fat feeding. H Plasma glucose, I NEFA and J insulin levels. K mRNA levels of HIF-target and thermogenic genes in BAT after high fat feeding at TN. Data are presented as mean + /− SEM. * P < 0.05, ** P < 0.01, Student t test (two-tailed). ns; no statistically significant. Source data are provided as a Source Data file.
One of the hallmarks of DIO is monocyte recruitment in adipose tissue and an increase of inflammatory macrophages 30 , therefore we performed analysis of immune cells in blood and WAT (Supplemental Fig. 4A ). Blood and WAT neutrophil numbers were similar in control and P2 KO ad mice (Supplemental Fig. 4B, D ). However, P2 KO ad mice had reduced numbers of blood monocytes (Supplemental Fig. 4C ), and a trend for lower WAT monocytes (Supplemental Fig. 4E ). No significant differences were detected in resident (Supplemental Fig. 4F ) and inflammatory macrophages (Supplemental Fig. 4G ) in WAT. P2 KO ad mice had lower mRNA levels of the monocyte chemotactic protein Ccl2 (Supplemental Fig. 4H ).
It is well established that HFD increases HIF-signalling in adipose tissue 31 , 32 , 33 , therefore it is not surprising that the HFD challenge reduced the differences detected with thermoneutrality alone. Indeed, the mRNA levels of the established HIF-signature genes ( Epas1 and Vegfa ) after HFD are identical between genotypes (Fig. 6K ). This could explain the less pronounced differences between genotypes after HFD at TN. Despite this, P2 KO ad mice still retained modestly higher Adrb3 mRNA, but not Ucp1 levels in the BAT (Fig. 6K ).
Pharmacological PHD inhibition induces UCP1 in murine and human adipocytes in vitro
Clinically, the most advanced developed inhibitors approved by EMA for renal anaemia treatment in chronic kidney disease, are targeting all 3 PHD isoforms (pan-PHD inhibitors; PHDi). PHDi leads to HIF-1a and HIF-2a stabilization in various experimental models and tissues 16 , 22 , 34 , 35 , 36 including adipose tissue 16 , 22 , 35 . Because the PHD-HIF pathway contains several components that makes dissection of major contributors on the observed effect challenging, we interrogated RNA-sequencing data on the expression levels of all the major components of the HIF/PHD pathway ( Phd1-2-3 , Hif1-2 ) in BAT in mice at room temperature (Supplemental Fig. 5A ). This directly comparable data show that in the BAT of 10-week old mice housed at room temperature, Phd2 expression is more than 5-fold higher than Phd1 , or Phd3 . Similarly, Hif2 is 10-fold higher than Hif1 expression. In addition, exposure to acute cold, does not regulate brown adipose tissue Hif1 mRNA levels, in contrast to Hif2 mRNA levels , which are elevated (Supplemental Fig. 6B ). Importantly, we found that in thermoneutrality, P2 KO ad Hif2 levels in BAT are similar to that seen in cold-treated controls (Supplemental Fig. 5B ). As PHD2 and HIF2 are the only 2 components of the HIF pathway highly enriched in BAT, we focused on their effects on UCP1 regulation.
To address clinical significance, we tested whether FG2216 (a small molecule pan PHDi) directly could induce UCP1 expression in vitro as we have seen in the genetic model of adipose-specific PHD2 deletion. In parallel, we determined whether the PHD2 isoform is mainly responsible for the observed effect on UCP1 expression. To do this we treated control and P2 KO ad brown adipocytes with FG2216 (Fig. 7A ). Basally, P2 KO ad brown adipocytes had already higher Ucp1 expression than untreated control. FG2216 increased Ucp1 mRNA levels in control brown adipocytes to similar levels seen in P2 KO ad brown adipocytes (Fig. 7A ). However, FG2216, did not further increase Ucp1 in P2 KO ad brown adipocytes, suggesting that inhibition of Phd1 and Phd3 was not enhancing the effect.

A P2 KO ad (red bars) brown adipocytes have higher Ucp1 mRNA levels than control (blue bars) mice and treatment with PHDi (FG2216, 10 µM, 16 h, n = 4, biological replicates) increases Ucp1 levels only in control brown adipocytes (green bars). The mouse brown adipocyte cell line (WT-1) treated with PHDi (10 µM, 16 h, n = 6; green bars) shows higher B UCP1 protein levels ( n = 4, biological replicates) compared to vehicle (DMSO, n = 4; blue bars). C mRNA levels of Adrb3 and Ucp1 are higher in the PHDi treated (green bars) WT-1 cells. Treatment with the HIF-2a antagonist (PT-2385, 10 µM, 16 h; pink bars) suppresses the effect ( n = 3/group) in contrast to the HIF-1a antagonist (PX-478, 10 µM, 16 h; yellow bars). D Schematic represents mUCP1 promoter region showing relative positions of putative HIF-2a response elements (identified using JASPAR). A–G represent putative HIF2a response elements at A; −4943, B; −4313, C; −3334, D; −2788, E; −1857, F; −120, G; +250, relative to the transcriptional start site ( + 1). ChIP performed on chromatin from WT-1 cells in hypoxia (1% O2, 6 h). PCR was performed using primer sets that amply regions covering putative HIF-2a response elements as outlined in schematic. Lettering represents sites G; +250 (recruitment), B; −4313 (recruitment) and C; −3334 (no recruitment). ns = non-specific control immunoglobulin, IP = immunoprecipitation. Input chromatin shown (1/10 dilution relative to IP PCR). Graph represents HIF-2a fold enrichment on mUCP1 promoter compared to non-specific control immunoglobulin. E , F Human adipocytes isolated from abdominal subcutaneous biopsies treated with PHDi (10 µM, 16 h, n = 3 biopsies; 3 replicates per biopsy) show increased ARB2 and UCP1 mRNA expression. Data are presented as mean + /− SEM. * P < 0.05, ** P < 0.01, *** P < 0.001 by Student t test (two-tailed). G illustrates that adipocyte-PHD2 deletion leads to HIF-2a activation, which in turn regulates the thermogenic pathway by sustained uncoupling protein (UCP)-1 expression and enhanced responsiveness of brown adipose tissue (BAT) under conditions where BAT is normally supressed. G was created with BioRender.com released under a Creative Commons Attribution-Non Commercial-NoDerivs 4.0 International license. Source data are provided as a Source Data file.
To confirm the PHDi effect seen in primary control brown adipocytes, we further tested a mouse immortalized BAT cell line 37 , that has comparable Ucp1 mRNA levels to mice at room temperature conditions (Supplemental Fig. 6 ). Treatment with FG2216, increased both UCP1 protein (Fig. 7B ) and mRNA levels (Fig. 7C ). The HIF-2a, and not the HIF-1a, signalling pathway was implicated as important for BAT function during cold-exposure ( 38 , 39 and Supplemental Fig. 5B ). To confirm we tested whether the PHD-HIF-2a axis underpinned the effects of the PHDi (FG2216). The effect of PHDi on Ucp1 and adrenergic receptor ( Adrb3) mRNA levels was completely abolished when brown adipocytes were treated with the HIF-2a antagonist PT-2385 40 , but not with the HIF1a antagonist, PX-478 (Fig. 7C ), suggesting that the PHD2-HIF-2a axis is a crucial regulator of Ucp1 expression levels. Mechanistically, HIF-2a chromatin immunoprecipitation PCR (CHiP-PCR) indicated that HIF-2a was directly associated with Ucp1 (Fig. 7D ) in brown adipocytes in hypoxia, as HIF-2a enrichment at two regions within mUCP1 (+250, −4300) was identified.
Importantly, PHDi treatment of human abdominal subcutaneous white adipocytes from individuals with obesity increased ADRB2 mRNA levels (Fig. 7E ), a pathway recently shown to be the main target for pharmacological activation of human brown adipocytes 41 . UCP1 mRNA expression in human adipocytes was also increased by PHDi-treatment (Fig. 7F ), suggesting that FG2216 could induce a beiging - like gene signature in human WAT.
Serum PHD2 protein levels are associated with metabolic dysfunction in humans
Our data suggest that cellular oxygen sensing proteins, and PHD2 in particular, regulate key metabolic functions and energy homeostasis when targeted in the adipocyte. We next sought genetic evidence for the potential involvement of PHD2 and downstream hypoxia inducible factor (HIF signalling) genes in the human metabolic phenotypes of the ~500,000 UKBioBANK genome-wide association (GWAS) database (using PhenoScanner) 42 . Genome-wide significant and/or suggestive association signals were found for blood glucose levels ( PHD2 ; rs578226800, P = 9.2E −08 ) and basal metabolic rate ( PHD2 ; rs7534248, P = 3.2E -06 ; HIF2A ; rs11689011, P = 9.5E −06 ; HIF1AN ; rs1054399, P = 6.9E −10 ). Finally, to test whether the most important known human oxygen sensor, PHD2, might serve as a target/biomarker for metabolic disease in humans, we determined if there was any association between serum PHD2 levels and metabolic syndrome traits. We analysed data acquired from a custom version of the SOMAscan proteomic profiling platform screen of serum samples from the large population-based (5457 participants) AGES-Reykjavik cohort 17 , 18 . The AGES Reykjavik study is a prospective study of deeply phenotyped and genotyped individuals older than 65 years of age. We found that PHD2 levels were significantly positively correlated with visceral adipose tissue (VAT via computed tomography), body mass index (BMI, kg/m 2 ), blood markers for type 2 diabetes, HBA1C and insulin, triglycerides and the metabolic syndrome (MetS; odds ratio 1.22) (Table 1 ).
In this study, we have shown that the adipocyte oxygen sensing pathway regulates the thermogenic pathway by sustained β-3-adrenergic signalling and uncoupling protein (UCP)-1 expression at thermoneutrality. We postulate that this is due to enhanced responsiveness of brown adipose tissue (BAT) under conditions where BAT is normally suppressed. This is associated with increased neo-vascularization and maintenance of brown adipose tissue thermogenic capacity that ultimately protects against metabolic dysfunction. The higher energy expenditure and metabolic protection was evident in both sexes and under high fat diet metabolic stress (Fig. 7G , graphical summary). We further provide evidence that human serum protein levels of PHD2 are directly correlated with measures of metabolic disease and could potentially be used as a biomarker.
Adipose tissue remodelling, in response to changing nutrient and environmental conditions, is critical to metabolic flexibility. Vascularization, in particular, is a crucial determinant of the oxygenation level in the tissue as recently confirmed in a human study 43 . Cifarelli et al. showed that adipose VEGFa expression was (a) significantly lower in metabolically unhealthy compared to metabolically healthy individuals with obesity and lean individuals and (b) positively associated with adipose pO 2 levels 43 . Our genetic model thus provides compelling evidence that deletion of Phd2 in adipocytes facilitates metabolic protection through multiple effects in functionally distinct adipocyte populations, including enhanced function/remodelling of BAT and enhanced lipid-retention capacity of WAT. Ultimately, this enhances whole animal energy expenditure even at thermoneutrality. However, as the mice also eat about double the amount of the controls, the outcome is that there is no difference in body weight. Thus, a caveat is-that increased metabolism does not necessarily lead to lower body weight. Metabolically, loss of adipose-PHD2 leads to lower basal lipolysis suggesting metabolic protection. It also promotes higher maximal thermogenic capacity and lipolytic capacity at thermoneutral conditions, suggesting readiness to utilize fatty acids for thermogenesis. Although, in our pan-adipose tissue KO model, BAT activation likely drives the observed phenotype, we cannot exclude that WAT thermogenesis may also contribute to the phenotype, as we did not test a BAT (UCP1cre)-specific KO model.
In this study, we did not observe any other major phenotypic difference resulting from adipose-PHD2 loss, for example in body weight, as reported by others at RT in diet-induced obesity 22 . We postulate that this is because HFD induced the HIF-signalling response in control mice, masking subtle differences between genotypes-in effect making HIF signalling convergent under the double insult of HFD and TN. Indeed, it is well documented that HFD increases HIF1 and HIF2 levels in WAT 31 , 32 and we have previously shown that HFD also reduces PHD2 levels in WAT 33 . It is also evident in our current BAT data that HIF2 ( Epas1) and the HIF-target gene ( Vegfa ) are similarly expressed in control and P2KO ad mice after HFD. Notably, another study, using a whole–body PHD2 inhibition, showed reduced body weight, WAT mass and higher energy expenditure at room temperature in mice kept on a diet that specifically induced fatty liver 35 . The authors suggested that improved beigeing of WAT could explain their higher heat production 35 . In WAT, beige adipogenesis may contribute to some degree to increased thermogenesis 44 , 45 , but it is unlikely to be the sole explanation. Pan-tissue PHD2 reduction, likely affected other tissues with a greater contribution to energy expenditure such as the skeletal muscle or brown adipose. Intriguingly, whole-body PHD2 inhibition exhibited reduced BAT mass 35 in contrast to our adipose-specific Phd2 deletion mouse model. Effects on skeletal muscle although not studied, could potentially explain enhanced thermogenesis. For example, a recent study showed that global or skeletal muscle deletion of the PHD3 isoform led to enhanced exercise endurance capacity and a small increase in maximum oxygen consumption rate (VO2) due to increased fatty acid oxidation in muscle 46 . We cannot directly compare the phenotype of the whole-body PHD2 inhibition to our study as they were conducted under different environmental temperatures (RT vs TN) and disease models (NAFLD vs HFD). However, a consistent finding between the studies is that targeting the PHD2 isoform, and specifically in adipose tissue, may have beneficial lipid lowering effects that could be explored further in humans.
Pan-PHD1-3 inhibitors have been developed to promote the production of endogenous erythropoietin and are effective in the treatment of renal anaemia in CKD patients. The most advanced PHDi, Roxadustat, was approved for marketing in China 13 and recently also authorised for use (under the marketing name Evrenzo, Astellas Pharma Ltd/FibroGen Inc) in the European Union by the European Medicines Agency 14 . Phase 3 clinical trials in CKD patients reported that alongside the primary outcomes to increase haemoglobin and erythropoietin levels, at least some PHDi (i.e. roxadustat, dabrodustat) can lower cholesterol and low-density lipoprotein cholesterol levels 47 , 48 . The lowering effects on plasma fatty acids 16 and cholesterol 15 , 35 have also been shown in animal models treated with PHDi 49 , 50 . Targeting BAT activation to alleviate metabolic dysfunction, especially to lower circulating lipid levels, a hallmark of metabolically unhealthy individuals with obesity 43 , is a promising new treatment line, as in both humans and mice activated BAT results in lowering of fasted and postprandial plasma triglyceride levels 51 , 52 .
Although PHD inhibition activates both HIF1 and HIF2, HIF isoforms enact non-overlapping functions and have dominant roles in different cell types 30 . Mechanistically, our data and others 8 , 38 , 50 suggest that the PHD2-HIF2 pathway, and not HIF1, is key to brown adipose tissue regulation. Together our data supports the hypothesis that HIF2 drives transcription of mUCP1 during hypoxia, likely via direct recruitment to HIF2 response elements within the mUCP1 gene.
It is conceivable that PHD inhibition could be used to enhance energy expenditure. Our human adipocytes from individuals with obesity and mouse BAT cells in vitro using an earlier analogue (FG2216) closely related to roxadustat (FG4592) showed induction of β3-adrenergic signalling and UCP1 levels. These are promising findings and suggest PHDi-dependent beiging effects. However, targeting isoform specificity would be more efficacious to enhancing BAT thermogenic capacity.
Finally, our human proteomics data provide unique insight on the role of PHD2 in age-related metabolic disease. The cell/tissue origin of serum PHD2 remains to be determined, however its presence suggests that metabolic dysfunction in ageing is associated with higher PHD2 levels and possibly altered oxygen sensing. This fits with our contention that PHD2 inhibition would have beneficial metabolic effects in humans. In a timely manner, as PHD inhibitors are completing Phase 3 trials or are now approved our findings suggest that this class of drugs, if optimised for isoform and tissue targeting, could be repurposed for treatment of certain metabolic diseases.
Limitations
We acknowledge that is not feasible to study mice strictly “at thermoneutrality”. This is because the thermoneutral point changes diurnally in mice 53 , 54 and thus both the metabolic rate and body temperature are not constant, making it complex for assessing physiological responses in “true” mouse thermoneutral conditions. However, we chose housing at 28–29 °C to facilitate better extrapolation of our finding to human translation. The effects of increased energy expenditure and reduced blood lipids in the adipose-specific PHD2 deletion is overall consistent in both sexes housed at thermoneutral conditions, which is encouraging to consider as a target to increase energy expenditure in humans. Finally, although we identified key new evidence that HIF2 binds to the Ucp1 promoter, providing at least one unequivocal mechanism whereby PHD2 deficiency leads to increased thermogenic gene expression, we do acknowledge that additional alternative mechanisms may also exist.
Study approvals
Animal study.
All animal experiments were performed under the project licence PP5702478, appropriate PILs granted under the Home Office Scientific Procedures (Animals) Act 1983 and after full ethical review by the University of Edinburgh Biological Sciences Services. Male and female mice were used for the experiments and were maintained single-housed in either standard or individually ventilated cages with ad libitum access to food (CRM E, Special Diets Services) and water and maintained with a 12-h light/dark cycle with lights on at 7 am, humidity 45–60%. Termination of experimental animals was done by (schedule 1 euthanasia) dislocation.
Human study
The Age, Gene/Environment Susceptibility Reykjavik Study (AGES-RS) was approved by the National Bioethics Committee (NBC) in Iceland (approval number VSN-00-063), which serves as the Icelandic Heart Association’s institutional review board, in accordance with the Helsinki Declaration, and by the National Institute on Aging Intramural Institutional Review Board, and the Data Protection Authority in Iceland, with all participants providing written informed consent. No compensation was provided for study participation.
Animal studies
The Phd2 conditional allele (PHD2 f/f ) 16 on a congenic C57BL/6 background (10 backcrosses), was crossed with the adiponectin–Cre allele (B6;FVB-Tg (Adipoq-cre)1Evdr/J, the Jackson Laboratories) to achieve adipose-specific conditional knockout mice (referred as P2 KO ad ). Genotyping PCRs were performed according to the established Jackson laboratories protocol ( https://www.jax.org/Protocol?stockNumber=028020&protocolID=25488 ). In all experiments described, control littermates were used for comparisons. Body weight, lean and fat mass were determined by time domain–nuclear magnetic resonance (TD-NMR) (Bruker LF50; http://www.bruker.com ) at RT (20–21 °C) and after 3 days at thermoneutrality (28–29 °C; TN). For the basal phenotyping control, chow diet with ad libitum access (CRM E, Special Diets Services) male and female mice (8–10 weeks old) were housed for 7 days (4 days acclimation and 3 days experimental measurements for example EE, RER, TD-NMR) in TN. Mice were single housed in indirect calorimetry cages (PhenoMaster, TSE Systems), at TN with free access to food and water, with a 12-h light and 12-h dark cycle (7 a.m. to 7 p.m.). Indirect calorimetry was used to measure energy expenditure (W), respiratory exchange ratio (RER: VCO 2 /VO 2 ), O 2 consumption, CO 2 production and physical activity (Counts/hour with X-Y-Z plane infra-red beam breaking). The EE ANCOVA analysis done for this work was provided by the NIDDK Mouse Metabolic Phenotyping Centers (MMPC, www.mmpc.org ) using their Energy Expenditure Analysis page ( http://www.mmpc.org/shared/regression.aspx ) and supported by grants DK076169 and DK115255. For the diet induced obesity experiments, male mice (10 week old) were fed a high fat diet (58% calories as fat with sucrose; D12331, full formula can be found https://www.researchdiets.com/formulas/d12331 (Research Diets Inc, Supplementary Table S1 ) for 8 weeks while housed at TN. To directly test BAT function, mice were given 2× CL316, 243 (1 μg/gr BW i.p., Merck), one injection at RT and another i.p. injection after switched to TN and culled 24 h later for tissue collection. For NEFA release, mice were fasted for 4 h, a single CL316, 243 (1 μg/gr BW) i.p. was given and blood collected basally (prior to CL) 15, 30, 60 min post CL. Paired experiments were designed (as indicated in figure legends) to allow direct phenotyping comparisons of the same mouse at different temperatures. All experiments/measurements were operator/animal handler blinded with data generated by a second individual blinded to treatment until code breaking.
BAT RNA-sequencing experiment, male C57BL/6J mice were housed under controlled conditions at 22 ± 2 °C in an 12/12-h light/dark cycle maintained on a standard chow diet (Ssniff, Soest, Germany). The animals were culled for tissue collection at 10 weeks of age. Adipose tissue RNA was isolated from approximately 50 mg of ground tissue. RNA-sequencing was conducted using Illumina HiSeq 2000 with 2 ×100 bp reads (LGC Genomics, Berlin, Germany). For data processing and analysis, FASTQC v0.11.8 was used for quality assessment and trim galore v0.6.4 was applied for filtering adapter sequences and small reads. Clean reads were mapped to a reference genome (Genome Reference Consortium Mouse Build 38 mm10) using the alignment tools HISAT2 v2.1.0 and Bowtie2 v2.3.5.1 Expression levels were quantified Cufflinks v.2.2.1.
Blood parameters
For non-esterified fatty acids (NEFA), glycerol, glucose and insulin measurements, blood was collected after a 4 h fast. Glucose concentration was measured by a blood glucose monitoring system (OneTouchUltra2, Lifescan, Milpitas). NEFA (Wako), and glycerol (Sigma Aldrich) was measured in plasma prepared from blood collected in EDTA-coated microtubes (Sarsted). Insulin concentration was measured by a commercial Elisa kit (Crystal Chem Inc.).
Mouse brown adipocyte culture
The WT—1 cell line was established from primary brown pre-adipocytes isolated from newborn mouse pups and immortalized with Simian virus 40 (SV40) large T antigen (38; Sigma). Briefly preadipocytes were grown in DMEM high glucose (20% FBS). A day after reaching confluence, medium was changed to induction media (Complete DMEM + IBMX 0.5 mM + Insulin 20 nM + Dexamathasone 5 µM + Indometacin 125 µM + T3 1 nM) for 2 days. Thereafter, cells were maintained in the differentiation media (Complete DMEM + Insulin 20 nM + T3 1 nM) for 5–6 days. All chemicals were from Sigma-Aldrich. Fully differentiated brown adipocytes were treated with 10 μM of 2-(1-chloro-4-hydroxyisoquinoline-3-carboxamido) acetic acid (FG-2216, Selleck Chemicals), a potent small molecule inhibitor of the PHD enzymes (PHDi) that has been shown to activate HIFα in adipose tissue 16 and/or the HIF-2a antagonist PT2385 (10 μM, MedChemExpress) and/or the HIF-1a antagonist PX-478 (10 μM, Cayman Chemical) for 16 h. Isolation of primary brown adipocytes from P2KO ad and control littermates was performed by collagenase digestion 16 and exposed to FG2216 (10 μM, 16 h). Adipocytes were lysed with TRI zol (Invitrogen) on ice and immediately frozen for further analysis.
Total RNA was extracted from cells and tissue using TRI zol (Invitrogen) and treated with DNase I (Invitrogen). 1 μg total RNA was used for first-strand DNA synthesis using Superscript III cDNA Synthesis system (Invitrogen), and qPCR was performed with the Lightcycler 480 (Roche), using mouse-Taqman assays (Life Technologies) for all genes measured. A standard curve was constructed for each gene measured using a serial dilution of cDNA pooled from all samples. Results were normalized to the expression of Ppia . A list of the TaqMan gene expression assays (ThermoFisher Scientific, UK) used can be found in Supplementary Table S2 .
Chromatin immunoprecipitation (ChIP)-PCR
WT-1 cells were fully differentiated into brown adipocytes and exposed to 1% O 2 for 6 h prior to fixation with 1% formaldehyde for 10 min (37 °C under 1% O 2 ) followed by quenching with 0.125 M glycine. Cells were lysed in lysis I (5 mM Tris-HCl pH 8.0, 85 mM KCl, 0.5% NP-40 plus protease inhibitors) for 20 min on ice and manually disrupted using 10 strokes with Dounce homogenizer before nuclei were pelleted by centrifugation (300 × g , 5 min, 4 °C). Nuclei were resuspended in nuclear lysis buffer (50 mM Tris-HCl pH 8.1, 10 mM EDTA, 1% SDS, plus protease inhibitors) on ice followed by sonication using Diagenode Bioruptor (30 cycles of 30 s on 30 s off). Samples were diluted in IP dilution buffer (0.01% SDS, 1.1% Triton x-100, 1.2 mM EDTA, 16.7 mM Tris-HCl pH 8.1, 167 mM NaCl) to achieve 0.1% SDS final, precleared for 30 min at 4 °C with blocked protein A/G (50/50 slurry of IP dilution buffer with 1 mg/mL BSA in TE, 400 μg/mL herring sperm DNA) before immunoprecipitation overnight with either HIF-2α antibody (1:250; NB100-122, Novus, Biologicals, Centennial, CO, USA) or rabbit immunoglobulin (1:250; Sigma, I5006) control. Immunoprecipitated complexes were collected using 25 μL protein A/G slurry. Samples were washed 2× each with: wash I (20 mM Tris-HCl pH 8.1, 500 mM NaCl, 2 mM EDTA, 0.1% SDS, 1% Triton x-100), wash II (0.25 M LiCl, 1% NP-40, 1% Na-deoxycholate, 1 mM EDTA, 10 mM Tris pH 8.1), TE buffer pH 8.0. Complexes were eluted (1% SDS, 0.1 M NaHCO 3 ) for 15 min at room temperature before undergoing reverse cross-linking and RNAse digestion (65 °C for 3 h followed by overnight at 55 °C). DNA was isolated using Qiagen columns according to manufacturer’s instructions. ChIP samples along with inputs (1/10 dilution) were analyzed by standard PCR using PAQ5000 hot start master mix (Agilent; Santa Clara, CA, USA, see primers in Supplementary Table S3 ). Quantification was performed using ImageJ/Fiji using the Gels plugin to generate lane profile plots. Results were exported to Excel and fold recruitment was calculated (IP/Ig control IP).
Immunoblot assays
Whole tissue or cell lysates were prepared in ice-cold buffer (5 mmol/L HEPES, 137 mmol/L NaCl, 1 mmol/L MgCl 2 , 1 mmol/L CaCl 2 , 10 mmol/L NaF, 2 mmol/L EDTA, 10 mmol/L Na pyrophosphate, 2 mmol/L Na 3 VO 4 , 1% Nonidet P-40, and 10% glycerol) containing protease inhibitors (Complete Mini; Roche Diagnostics Ltd). Blots were probed with HIF-1α (1:200; 10006421; Cayman), UCP1 (1:800; PA1-24894, ThermoFisher) antibodies. HRP-conjugated anti-rabbit (1:1000; Dako) secondary antibody was used. Signal was detected using ECL Plus (GE Healthcare Life Sciences). Blots were re-probed with an HRP-conjugated anti-actin antibody (1:10,000; ab49900, abcam). Densitometry was performed using the ImageJ software.
Histomorphological assessment of brown adipose
Formalin-fixed, paraffin-embedded brown adipose sections (4μm) were used. Images were acquired using a Zeiss microscope (Welwyn Garden City, Hertfordshire, UK) equipped with a Kodak DCS330 camera (Eastman Kodak, Rochester, NY). Six randomly selected fields per BAT section in each mouse stained with H&E were captured with 10x objective. Adiposoft software (ImageJ, 1.53c) was used to determine mean adipocyte size (μm 2 ) and mean adipocyte count.

Immunofluorescence in BAT
Paraffin-embedded BAT samples (sections 4 μm) were dewaxed by sequential incubations with xylene for 10 min, 100% ethanol for 1 min, 95% ethanol for 1 min, 80% ethanol for 1 min and 70% ethanol for 1 min. After dewaxing, slides went through an antigen retrieval step by boiling the slides in citrate buffer (0.05% Tween, pH 6) for 5 min. After fixation/dewaxing, slides were washed three times with PBS for 10 min then blocked with 10% goat serum for 1 h at RT. Sections were then incubated overnight at 4 °C with the following primary antibody: UCP1 (1:500, ab10983, Abcam), KI67 (1:50, NB500-170SS, Novus Biologicals) and isolectin B4 (1:200, B-1205, Vector Laboratories). Next day, slides were washed three times with PBS for 5 min. Subsequently, sections were incubated for 1 h at room temperature with species‐specific secondary antibodies diluted 1:300. The following fluorochrome‐conjugated secondary antibodies were used: anti‐rabbit‐Alexa 555 IgG, anti‐rabbit‐Alexa 647 IgG, and streptavidin conjugated 488 (all from Life Technologies). After incubation slides were washed three times with PBS for 10 min. Slides were mounted using Fluoramount G (SouthernBiotech) and images were acquired using a fluorescence microscope (Zeiss Observer, Zeiss) or a slide scanner (Zeiss, Axio Scan). Images were processed using ZEN Blue lite version (Zeiss). For quantification, random images were taken from different areas of BAT sections. The images were then split into different fluorescent channels (DAPI, AF488, AF555 or AF647) and analyzed with CellProfiler 55 to measure the median intensity of the proteins of interest (UCP1, IB4, Ki67). Data obtained from CellProfiler was then analyzed with Flowjo software or excel to obtain the number of positive cells. Negative controls images were used to gate the positive population. UCP1+ and IB4+ are the mean of n = 2–3 sections/animal from three biological samples.
Murine tissue and blood preparation for flow cytometry
Murine epididymal adipose tissue were enzymatically digested with 1 mg/ml Collagenase D (Roche) for 35 min at 37 °C in RPMI 1640 (Sigma) containing 1% foetal bovine serum (FBS) (Sigma). Blood was collected in EDTA-coated microtubes and 100 μl of blood was stained prior to red blood cell lysis with FACS lysing solution (BD). For flow-cytometry, murine cells were stained with LIVE/DEAD (Invitrogen), blocked with mouse serum and anti-murine CD16/32 (clone 2.4G2, Biolegend, 1:300) and stained for cell surface markers (Supplementary Table S4 ). DAPI was added to the cells prior to acquisition. All samples were acquired using a BD Fortessa LS6 and analyzed with FlowJo software (Tree Star).
Isolation of mature human adipocytes
Ethical approval (reference number 15/ES/0094) for the collection, storage and subsequent use of human adipose tissue was granted by The Human Tissue (Scotland) Act 2006 and informed written consent was obtained from each participant. No compensation was provided for study participation. Abdominal subcutaneous adipose biopsies were collected from 3 “self-reported” females (48±4 years old) undergoing elective surgery for hernia repair or laparoscopic cholecystectomy in the Royal Infirmary of Edinburgh. Anthropometric characteristics include BMI (37.8±2.5 kg/m 2 ), waist to hip ratio (WHR: 0.88±0.023), %fat (43.3±1.8), fat mass (43.5±9 kg). Mature adipocytes were prepared from adipose tissue by collagenase digestion. Briefly, adipose tissue was immediately placed in warm KREBS buffer (Sigma-Aldrich) with 1% antibiotic/antimycotic solution, 0.5% BSA (Sigma-Aldrich) and 2 mg/ml Collagenase Type 1 (CLS-1, Worthington Biochemical) and cut into small pieces using sterile scissors and forceps and incubated for 35–45 min in shaking water bath at 37 °C. The digested samples were centrifuged at 300 × g for 5 min at 22 °C, the mature adipocytes were collected from the top portion of the centrifuge tube and transferred to a Falcon tube, washed 3× with PBS and the mature packed volume was carefully collected from the upper layer into Eppendorf tubes for immediate culture and treatment. Mature adipocytes were exposed to FG2216 (10 μM, 16 h), and immediately lysed for RNA extraction and RT-qPCR as described above.
Human study population
Participants aged 66 through 96 are from the AGES-RS cohort 11 . AGES-RS is a single-centre prospective population-based study of deeply phenotyped subjects (5764, mean age 75 ± 6 years, 57% female “self-reported”) and survivors of the 40-year-long prospective Reykjavik study ( n ~ 18,000), an epidemiologic study aimed to understand aging in the context of gene/environment interaction by focusing on four biologic systems: vascular, neurocognitive (including sensory), musculoskeletal, and body composition/metabolism. Descriptive statistics of this cohort as well as detailed definition of the various disease relevant phenotypes measured have been published 17 , 18 . Definition of outcomes used in the present study: body mass index was estimated as kg/m 2 . Visceral fat area was estimated from computed tomography images at the L4/L5 vertebrae. Area was calculated from single 10 mm trans axial images using specialized software. Blood samples were drawn after overnight fasting and centrifuged within 2 h at room temperature. Triglyceride, fasting glucose and HbA1c were analysed in serum using a Hitachi 912 analyzer (Roche Diagnostics) with reagents from Roche Diagnostics. Serum insulin was measured with a Roche Elecsys 2010 instrument. Type 2 diabetes was defined as fasting serum glucose >7.0 mmol/L or self-reported history of diabetes or the use of insulin or oral glucose-lowering drugs. Impaired fasting glucose was defined in the range 5.6–6.9 mmol/L, excluding individuals with known type II diabetes at baseline. Metabolic syndrome was defined as meeting three of the following criteria: (i) waist/abdominal circumference >102 cm for men and >88 cm for women, (ii) triglycerides >1.69 mmol/L, (iii) high density lipoprotein <1.04 mmol/L for men and <1.30 mmol/L for women, (iv) fasting glucose >6.1 mmol/L or treated diabetes (use of antidiabetic medications—ATC group A10), (v) systolic blood pressure greater than or equal to 130 or diastolic blood pressure greater than or equal to 85 or treated hypertension (use of antihypertensive medications).
Human serum protein measurements
Blood samples were collected at the AGES-Reykjavik baseline visit after an overnight fast, and serum prepared using a standardized protocol and stored in 0.5 mL aliquots at −80 °C. As previously described 56 , 57 , 58 , a customized version of the SOMApanel proteomics platform was developed to include proteins known or predicted to be found in the extracellular milieu measuring 4137 unique proteins. Here for instance, PHD2 had its own detection reagent selected from chemically modified DNA libraries, referred to as Slow Off-rate Modified Aptamers (SOMAmers). The SOMAmer-based platform measures proteins with femtomole (fM) detection limits and a broad detection range or >8 logs of concentration. To avoid batch or time of processing biases, the order of sample collection and separately sample processing for protein measurements were randomized, and all samples run as a single set at SomaLogic Inc. (Boulder, CO, USA). All SOMAmers that passed quality control had median intra-assay and inter-assay coefficient of variation, CV < 5%. Hybridization controls were used to correct for systematic variability in detection and calibrator samples of three dilution sets (40%, 1% and 0.005%) were included so that the degree of fluorescence was a quantitative reflection of protein concentration. Consistent target specificity across the platform was indicated by direct (through mass spectrometry) and/or indirect validation of the SOMAmers 56 . Prior to the association analysis the protein data was centred, scaled and BoxCox transformed 59 , and extreme outlier values excluded, defined as values above the 99.5th percentile of the distribution of 99th percentile cutoffs across all proteins after scaling, resulting in the removal of an average 11 samples per SOMAmer. Analyses were conducted using R version 4.2.1. and R RStudio (1.1.456).
All data are shown as the mean ± SEM, and a two-tailed Student’s t test was used to compare two groups. When paired design was performed (i.e., switching housing of the same animal form RT to TN), significance was tested by paired Student’s t test. Comparisons of 3 or more groups by one-way ANOVA. Data sets were analysed using GraphPad Prism version 8 (San Diego, California). The number of biological replicates is indicated as (n). Unpaired t test with Welch’s correction was used for the immunofluorescence data. For the inhibitor in vitro experiments, a paired Student’s test was performed to compare effects before and after drug administration in adipocytes from the same individual. A P value of less than 0.05 was considered statistically significant. For the associations of PHD2 to different human phenotypic measures we used linear or logistic regression depending on the outcome being continuous or binary and adjusted for age and sex in our regression analyses. Summary statistics for continuous outcome variables are listed as mean (standard deviation) and median and interquartile range (IQR) for skewed variables. For categorical variables as number and percentages n (%): Body mass index 27.1 (4.4), visceral fat area 172.8 (80.2), triglycerides 1.0 IQR [0.78,1.43], fasting glucose 5.8 (1.2), HbA1c 0.5 (0.1), insulin 1.2 IQR [0.79,1.78], type II diabetes 658 (12.1%), impaired fasting glucose 1982(41.4%), metabolic syndrome 1677(30.8%).
Reporting summary
Further information on research design is available in the Nature Portfolio Reporting Summary linked to this article.
Data availability
The data that support this study are available in the Source data file. Uncropped western blot images are available also in the Source data file. The custom-design proteomics SOMAscan is available through a collaboration agreement with the Novartis Institutes for BioMedical Research ([email protected]). Data from the AGES Reykjavik study are available through collaboration ([email protected]) under a data usage agreement with the IHA. All access to data is controlled via the use of a subject-signed informed consent authorization. The time it takes to respond to requests varies depending on their nature and circumstances of the request, but it will not exceed 14 working days. Summary statistics data for each protein’s genetic determinants, i.e., protein quantitative trait loci (QTLs), have been released to a public repository (GWAS catalogue), with accession numbers detailed in Gudjonsson et al. (PMID: 35078996). Mass spectrometry data (DDA or MRM) were deposited to the ProteomeXchange Consortium with the dataset identifiers PXD008819 to PXD008823, as well as the dataset identifier PASS01145 , to determine the specificity of aptamers binding to target proteins (PMID: 30072576). The mass spectrometry-based validation data for many of the aptamers included in the custom SomaScan panel used in this study are available from the PRIDE database ( https://www.ebi.ac.uk/pride/ ) under accessions PXD008819 , PXD008820 , PXD008821 , PXD008822 , and PXD008823 . The PASSEL repository ( https://peptideatlas.org/passel/ ) under accession PASS01145 . The RNA-sequencing raw data have been uploaded to NCBIs’ Gene Expression Omnibus (GEO) database and can be downloaded with GEO accession number GSE269003 . Source data are provided with this paper.
Chen, K. Y. et al. Opportunities and challenges in the therapeutic activation of human energy expenditure and thermogenesis to manage obesity. J. Biol. Chem. 295 , 1926–1942 (2020).
Article CAS PubMed Google Scholar
Marlatt, K. L. & Ravussin, E. Brown adipose tissue: an update on recent findings. Curr. Obes. Rep. 4 , 389–396 (2017).
Article Google Scholar
Kajimura, S., Spiegelman, B. & Seale, P. Brown and beige fat: physiological roles beyond heat generation. Cell Metab. 22 , 546–559 (2015).
Article CAS PubMed PubMed Central Google Scholar
Overton, J. M. Phenotyping small animals as models for the human metabolic syndrome: thermoneutrality matters. Intern. J. Obes. 34 , S53–S58 (2010).
Speakman, J. R. & Keiher, J. Not so hot: optimal housing temperatures for mice to mimic the thermal environment of humans. Mol. Metab. 2 , 5–9 (2013).
Article CAS Google Scholar
Fischer, A. W., Cannon, B. & Nedergaard, J. Optimal housing temperatures for mice to mimic the thermal environment of humans: an experimental study. Mol. Metab. 7 , 161–170 (2018).
Gordon, C. J. The mouse thermoregulatory system: its impact on translating biomedical data to humans. Physiol. Behav. 179 , 55–66 (2017).
Xue, Y. et al. Hypoxia-independent angiogenesis in adipose tissues during cold acclimation. Cell Metab. 9 , 99–109 (2009).
Ivan, M. et al. HIFalpha targeted for VHL-mediated destruction by proline hydroxylation: implications for O 2 sensing. Science 292 , 464–468 (2001).
Article ADS CAS PubMed Google Scholar
Jaakkola, P. et al. Targeting of HIF-alpha to the von Hippel-Lindau ubiquitylation complex by O 2 -regulated prolyl hydroxylation. Science 292 , 468–472 (2001).
Semenza, G. L. Pharmacological targeting of hypoxia-inducible factors. Annu. Rev. Pharm. Toxicol. 59 , 379–403 (2019).
Dhillon, S. Roxadustat: first global approval. Drugs 79 , 563–572 (2019).
Dhillon, S. Daprodustat. First approval. Drugs 80 , 1491–1497 (2020).
European Medicine Agency. Evrenzo approval (August 2021). https://www.ema.europa.eu/en/medicines/human/EPAR/evrenzo (2021).
Rahtu-Korpela, L. et al. HIF prolyl 4-hydroxylase-2 inhibition improves glucose and lipid metabolism and protects against obesity and metabolic dysfunction. Diabetes 63 , 3324–3333 (2014).
Michailidou, Z. et al. Adipocyte pseudohypoxia suppresses lipolysis and facilitates benign adipose tissue expansion. Diabetes 64 , 733–745 (2015).
Harris, T. M. et al. Age, Gene/Environment Susceptibility-Reykjavik Study: multidisciplinary applied phenomics. Am. J. Epidemiol. 165 , 1076–1087 (2007).
Article PubMed Google Scholar
Emilsson, V. et al. Co-regulatory networks of human serum proteins link genetics to disease. Science 361 , 769–773 (2018).
Article ADS CAS PubMed PubMed Central Google Scholar
Cannon, B. & Nedergaard, J. Nonshivering thermogenesis and its adequate measurement in metabolic studies. J. Exp. Biol. 214 , 242–253 (2011).
Cui, X. et al. Thermoneutrality decreases thermogenic program and promotes adiposity in high‐fat diet‐fed mice. Physiol. Rep. 4 , e12799 (2016).
Article PubMed PubMed Central Google Scholar
Hussain, M. F., Roesler, A. & Kozak, L. Regulation of adipocyte thermogenesis: mechanisms controlling obesity. FEBS J. 16 , 3370–3385 (2020).
Matsuura, H. et al. Prolyl hydroxylase domain protein 2 plays a critical role in diet-induced obesity and glucose intolerance. Circulation 127 , 2078–2087 (2013).
Eguchi, J. et al. Transcriptional control of adipose lipid handling by IRF4. Cell Metab. 13 , 249–259 (2011).
Lee, K. Y. et al. Lessons on conditional gene targeting in mouse adipose tissue. Diabetes 62 , 864–874 (2013).
Kaikaew, K., Grefhorst, A. & Visser, J. A. Sex differences in brown adipose tissue function: sex hormones, glucocorticoids, and their crosstalk. Front. Edocrinol. 12 , 652444 (2021).
Keuper, M. & Jastroch, M. The good and the BAT of metabolic sex differences in thermogenic human adipose tissue. Mol. Cell Endocrinol. 533 , 111337 (2021).
Wibmer, A. G. et al. Brown adipose tissue is associated with healthier body fat distribution and metabolic benefits independent of regional adiposity. Cell Rep. Med. 2 , 100332 (2021).
Bukowiecki, L. J. et al. Proliferation and differentiation of brown adipocytes from interstitial cells during cold acclimation. Am. J. Physiol. 250 , C880–C887 (1986).
Ramseyer, V. D. & Granneman, J. G. Adrenergic regulation of cellular plasticity in brown, beige/brite and white adipose tissues. Adipocyte 5 , 119–112 (2016).
Michailidou, Z., Gomez-Slazar, M. & Alexaki, V. I. Innate immune cells in the adipose tissue in health and metabolic disease. J. Innate Immun . 14 , 4–30 (2022).
Shimba, S., Wada, T., Hara, S. & Tezuka, M. EPAS1 promotes adipose differentiation in 3T3-L1 cells. J. Biol. Chem. 279 , 40946–40953 (2004).
Halberg, N. et al. Hypoxia-inducible factor 1alpha induces fibrosis and insulin resistance in white adipose tissue. Mol. Cell Biol. 29 , 4467–4483 (2009).
Michailidou, Z. et al. Increased angiogenesis protects against adipose hypoxia and fibrosis in metabolic disease-resistant 11β-hydroxysteroid dehydrogenase type 1 (HSD1)-deficient mice. J. Biol. Chem. 287 , 4188–4197 (2012).
Yeh, T. L. et al. Molecular and cellular mechanisms of HIF prolyl hydroxylase inhibitors in clinical trials. Chem. Sci. 8 , 7651–7668 (2017).
Laitakari, A. et al. HIF-P4H-2 inhibition enhances intestinal fructose metabolism and induces thermogenesis protecting against NAFLD. J. Mol. Med. 98 , 719–731 (2020).
Frost, J., Ciulli, A. & Rocha, S. RNA-seq analysis of PHD and VHL inhibitors reveals differences and similarities to the hypoxia response. Wellcome Open Res. 4 , 17 (2019).
Fasshauer, M. et al. Essential role of insulin receptor substrate-2 in insulin stimulation of Glut4 translocation and glucose uptake in brown adipocytes. J. Biol. Chem. 275 , 25494–25501 (2000).
Garcia-Martin, R. et al. adipocyte-specific hypoxia inducible factor 2a deficiency exacerbates obesity-induced brown adipose dysfunction and metabolic dysregulation. Mol. Cell Biol. 36 , 376–393 (2015).
Krishnan, J. et al. Dietary obesity-associated Hif1a activation in adipocytes restricts fatty acid oxidation and energy expenditure via suppression of Sirt2-NAD+ system. Genes Dev. 26 , 259–270 (2012).
Wallace, E. M. et al. A small-molecule antagonist of HIF2a is efficacious in preclinical models of renal cell carcinoma. Cancer Res. 76 , 5491–5500 (2016).
Blondin, D. P. et al. Human brown adipocyte thermogenesis is driven by β2-AR stimulation. Cell Metab. 32 , 287–300 (2020).
Staley, J. R. et al. PhenoScanner: a database of human genotype-phenotype associations. Bioinformatics 32 , 3207–3209 (2016).
Cifarelli, V. et al. Decreased adipose tissue oxygenation associates with insulin resistance in individuals with obesity. J. Clin. Investig. 130 , 6688–6699 (2020).
Cohen, J. W. & Speigelman, B. C. Adaptive thermogenesis in adipocytes. Is beige the new brown? Genes Dev. 27 , 234–250 (2013).
Ikeda, K. et al. UCP1-independent signalling involving SERCA-2b mediated calcium cycling regulates beige fat thermogenesis and systemic glucose homeostasis. Nat. Med. 23 , 1454–1465 (2017).
Yoon, H. et al. PHD3 loss promotes exercise capacity and fat oxidation in skeletal muscle. Cell Met 32 , 215–228 (2020).
Chen, N. et al. Roxadustat for anemia in patients with kidney disease not receiving dialysis. New Engl. J. Med. 381 , 1001–1010 (2019).
Baker, K. A. & Jones, J. J. An emerging treatment alternative for anemia in chronic kidney disease patients: a review of daprodustat. Adv. Ther. 35 , 5–11 (2018).
Sugahara, M. et al. Prolyl hydroxylase domain inhibitor protects against metabolic disorders and associated kidney disease in obese type 2 diabetic mice. J. Am. Soc. Nephrol. 31 , 560–577 (2020).
Zhang et al. Adipocyte hypoxia-inducible factor 2α suppresses atherosclerosis by promoting adipose ceramide catabolism. Cell Metab. 30 , 937–951 (2019).
Bartlet, A. et al. Brown adipose tissue activity controls triglyceride clearance. Nat. Med. 17 , 200–205 (2011).
Berbée, J. F. et al. Brown fat activation reduces hypercholesterolaemia and protects from atherosclerosis development. Nat. Commun. 6 , 6356 (2015).
Article ADS PubMed Google Scholar
Gordon, C. J. Thermal physiology of laboratory mice: defining thermoneutrality. J. Therm. Biol. 37 , 654–685 (2012).
Škop, V. et al. Mouse thermoregulation: introducing the concept of the thermoneutral point. Cell Rep. 31 , 107501 (2020).
McQuin, C. et al. CellProfiler 3.0: next-generation image processing for biology. PLoS Biol. 16 , e2005970 (2018).
Gudjonsson, A. et al. A genome-wide association study of serum proteins reveals shared loci with common diseases. Nat. Commun. 13 , 480 (2022).
Emilsson, V. et al. A proteogenomic signature of age-related macular degeneration in blood. Nat. Commun. 13 , 3401 (2022).
Emilsson, V. et al. Coding and regulatory variants are associated with serum protein levels and disease. Nat. Commun. 13 , 481 (2022).
Max Kuhn, K. J. Applied Predictive Modeling (Springer, 2013).
Download references
Acknowledgements
This work was supported by the British Heart Foundation/University of Edinburgh Centre of Research Excellence Award (RE/13/3/30183 to Z.M. & RE/18/5/34216 to Z.M., T.C. and N.M.M.), Wellcome Trust ISSF3 (IS3-R3.13 21/22 to Z.M.) and a Nottingham Trent University quality related research fund (RA932 to Z.M.). C.J.S. and N.M.M. (New Investigator award 100981/Z/13/Z) thank the Wellcome Trust for funding. I.K. is funded by the Academy of Medical Sciences (R2429101) and Rosetrees Trust (R2449101). Human adipose tissue collections were supported by a grant from the Chief Scientist Office (SCAF/17/02 to RHS) and the authors acknowledge the financial support of NHS Research Scotland (NRS), through the Edinburgh Clinical Research Facility. The authors are grateful to Professor Sir Peter Ratcliffe (Oxford University) for providing the Phd2 flox/flox mice, valuable discussions and advice on the manuscript. The graphical summary in Fig. 7g and schematic in Fig. 5a were created with BioRender.com .
Author information
Authors and affiliations.
Centre for Cardiovascular Sciences, Queen’s Medical Research Institute, University of Edinburgh, Edinburgh, UK
Rongling Wang, Mario Gomez Salazar, Iris Pruñonosa Cervera, Karen French, Marlene Magalhaes Pinto, Roland H. Stimson, Cécile Bénézech, Triantafyllos Chavakis, Nicholas M. Morton & Zoi Michailidou
Department of Biosciences, School of Science and Technology, Nottingham Trent University, Clifton, Nottingham, UK
Amanda Coutts, Mark Christian, Nicholas M. Morton & Zoi Michailidou
Department of Adipocyte Development and Nutrition, German Institute of Human Nutrition, Potsdam-Rehbrücke, Nuthetal, Germany
Sabrina Gohlke & Tim J. Schulz
Department of Immunology and Oncology, Centro Nacional de Biotecnología/CSIC (CNB-CSIC), Campus-UAM, Madrid, Spain
Ruben García-Martín
Helmholtz Institute for Metabolic, Obesity and Vascular Research (HI-MAG) of the Helmholtz Zentrum München at the University of Leipzig and University Hospital Leipzig, Leipzig, Germany
Matthias Blüher
Chemistry Research Laboratory, Department of Chemistry and the Ineos Oxford Institute for Antimicrobial Research University of Oxford, Oxford, UK
Christopher J. Schofield
Hull York Medical School, York Biomedical Research Institute, University of York, York, UK
Ioannis Kourtzelis
German Center for Diabetes Research (DZD), München-Neuherberg, Germany
Tim J. Schulz & Triantafyllos Chavakis
Icelandic Heart Association, Kopavogur, Iceland
Elias F. Gudmundsson, Vilmundur G. Gudnason & Valur Emilsson
Novartis Institutes for Biomedical Research, Cambridge, MA, USA
Lori L. Jennings
Faculty of Medicine, University of Iceland, Reykjavik, Iceland
Vilmundur G. Gudnason & Valur Emilsson
Institute for Clinical Chemistry and Laboratory Medicine, Faculty of Medicine, Technische Universität Dresden, Dresden, Germany
Triantafyllos Chavakis
Paul Langerhans Institute Dresden, Helmholtz Zentrum München, University Hospital and Faculty of Medicine Technische Universität Dresden, Dresden, Germany
You can also search for this author in PubMed Google Scholar
Contributions
R.W., M.G.S., I.P.C., A.C., K.F., C.B., I.K., M.M.P., and M.C. performed experiments/analysed data. R.G.M., M.B., C.J.S., R.H.S., and T.C. provided resources and/or supported with data interpretation. T.J.S. and S.G. contributed the brown adipose tissue RNA-seq data. N.M.M. designed/performed experiments. E.F.G., L.L.J., V.G., and V.E. contributed the human proteomics data. Z.M. conceived the study, acquired funding, performed experiments, supervised research and wrote the manuscript, which was reviewed by all authors.
Corresponding author
Correspondence to Zoi Michailidou .
Ethics declarations
Competing interests.
L.L.J. is an employee and stockholder of Novartis. V.E. V.G. C.J.S., N.M.M, R.H.S, M.G.S, T.C., R.G.M., I.P.C, K.F., R.W., C.B., M.C., A.C., T.J.S, S.G., M.M.P., I.K., S.G., T.J.S., and Z.M. declare no competing interests.
Peer review
Peer review information.
Nature Communications thanks the anonymous reviewer(s) for their contribution to the peer review of this work. A peer review file is available.
Additional information
Publisher’s note Springer Nature remains neutral with regard to jurisdictional claims in published maps and institutional affiliations.
Supplementary information
Supplementary information, peer review file, reporting summary, source data, source data, rights and permissions.
Open Access This article is licensed under a Creative Commons Attribution 4.0 International License, which permits use, sharing, adaptation, distribution and reproduction in any medium or format, as long as you give appropriate credit to the original author(s) and the source, provide a link to the Creative Commons licence, and indicate if changes were made. The images or other third party material in this article are included in the article's Creative Commons licence, unless indicated otherwise in a credit line to the material. If material is not included in the article's Creative Commons licence and your intended use is not permitted by statutory regulation or exceeds the permitted use, you will need to obtain permission directly from the copyright holder. To view a copy of this licence, visit http://creativecommons.org/licenses/by/4.0/ .
Reprints and permissions
About this article
Cite this article.
Wang, R., Gomez Salazar, M., Pruñonosa Cervera, I. et al. Adipocyte deletion of the oxygen-sensor PHD2 sustains elevated energy expenditure at thermoneutrality. Nat Commun 15 , 7483 (2024). https://doi.org/10.1038/s41467-024-51718-7
Download citation
Received : 09 April 2022
Accepted : 14 August 2024
Published : 29 August 2024
DOI : https://doi.org/10.1038/s41467-024-51718-7
Share this article
Anyone you share the following link with will be able to read this content:
Sorry, a shareable link is not currently available for this article.
Provided by the Springer Nature SharedIt content-sharing initiative
By submitting a comment you agree to abide by our Terms and Community Guidelines . If you find something abusive or that does not comply with our terms or guidelines please flag it as inappropriate.
Quick links
- Explore articles by subject
- Guide to authors
- Editorial policies
Sign up for the Nature Briefing: Translational Research newsletter — top stories in biotechnology, drug discovery and pharma.

- Harvard Business School →
- Faculty & Research →
Maria P. Roche
Assistant professor of business administration.
Maria Roche is an Assistant Professor of Business Administration in the Strategy Unit at Harvard Business School. She teaches Strategy in the MBA required curriculum. Professor Roche studies the commercialization of specialized knowledge and the specific role of micro-geography in innovation. Her findings indicate that organizations can unlock significant innovation and performance benefits by leveraging micro-geographic nuances in the sourcing, production, and diffusion of knowledge.
Her work, published at Management Science , The Review of Economics and Statistics , Organization Science and Research Policy , has been featured in The Atlantic , the WSJ , and the Handelsblatt . She received best dissertation awards from the Technology and Innovation Management division at the Academy of Management, and from the Society for the Advancement of Management Studies (EGOS). She serves on the editorial review boards of Organization Science , and Strategy Science .
Professor Roche earned her PhD in Management (Strategy and Innovation) at the Scheller College of Business, Georgia Institute of Technology, where she was a recipient of a NSF Science of Science and Innovation Policy Doctoral Dissertation Research Improvement Grant. She earned an MS in Business Administration and a BA in International Cultural and Business Studies at the University of Passau, Germany. Professor Roche has lived and worked in five countries gathering professional experience in various industries such as venture capital and film.

We examine the influence of physical proximity on between-start-up knowledge spillovers at one of the largest technology coworking hubs in the United States. Relying on the exogenous assignment of office space to the hub’s 251 start-ups, we find that proximity positively influences knowledge spillovers as proxied by the likelihood of adopting an upstream web technology already used by a peer start-up. This effect is largest for start-ups within close proximity of each other and quickly decays; start-ups more than 20 meters apart on the same floor are indistinguishable from start-ups on different floors. The main driver of the effect appears to be social interactions. Although start-ups in close proximity are most likely to participate in social coworking space events together, knowledge spillovers are greatest between start-ups that socialize but are dissimilar. Ultimately, start-ups that are embedded in environments that have neither too much nor too little diversity perform better but only if they socialize.
Featured in WSJ , NBER Bulletin , HBS Working Knowledge , HBS Alumni Stories , and the Handelsblatt

In this paper, I analyze how the physical layout of cities affects innovation by influencing the organization of knowledge exchange. I exploit a novel data set covering all census block groups in the contiguous United States with information on innovation outcomes, street infrastructure, as well as population and workforce characteristics. To deal with concerns of omitted variable bias, I apply commuting zone fixed effects and construct instruments based on historic city planning. The results suggest that variation in street network density may explain regional innovation differentials beyond the traditional location externalities found in the literature. Featured in Bloomberg

We propose a framework to guide managers on identifying the optimal work configuration for maximizing the effectiveness of their knowledge-driven organization. These insights draw upon over a dozen large-sample empirical projects and case studies we’ve conducted both before and during the pandemic, across different industries.
Featured in HBS Alumni Stories

Set in 2022, this case describes the considerations involved in organizing the physical relocation of the Rowland Institute at Harvard (RIH), a research institute established in 1980 by Edwin H. Land, the founder of the Polaroid Corporation, for the advancement of science. After 43 years at 100 Edwin H. Land Boulevard, the RIH needs a new home. As she evaluates available options, Leigh Needleman, the executive director of the Institute, not only has a long legacy to consider but must also decide where to relocate, whether to buy or rent the new space, and how to assign new laboratory and office spaces to staff to best foster breakthrough science and innovation.
- Roche, Maria P., Alexander Oettl, and Christian Catalini. "Proximate (Co-)Working: Knowledge Spillovers and Social Interactions." Management Science (forthcoming). (Pre-published online February 14, 2024.) View Details
- Roche, Maria P. "Academic Entrepreneurship: Entrepreneurial Advisors and Their Advisees' Outcomes." Organization Science 34, no. 2 (March, 2023): 959–986. View Details
- Roche, Maria P., and Andy Wu. "What's the Optimal Workplace for Your Organization?" Harvard Business Review Digital Articles (February 9, 2022). View Details
- Conti, Annamaria, and Maria P. Roche. "Lowering the Bar? External Conditions, Opportunity Costs, and High-Tech Startup Outcomes." Organization Science 32, no. 4 (July–August 2021): 965–986. View Details
- Roche, Maria P., Annamaria Conti, and Frank T. Rothaermel. "Different Founders, Different Firms: A Comparative Analysis of Academic and Non-academic Startups." Special Issue on Innovative Start-Ups and Policy Initiatives. Research Policy 49, no. 10 (December 2020). View Details
- Roche, Maria P. "Taking Innovation to the Streets: Micro-geography, Physical Structure and Innovation." Review of Economics and Statistics 102, no. 5 (December 2020): 912–928. View Details
- Gross, Daniel P., and Maria P. Roche. "Coordinated R&D Programs and the Creation of New Industries." Harvard Business School Working Paper, No. 24-027, April 2023. View Details
- Boudou, Justine, and Maria Roche. "Bringing Science to Market: Knowledge Foundations and Performance." Harvard Business School Working Paper, No. 24-021, October 2023. (Revised May 2024.) View Details
- Conti, Annamaria, Vansh Gupta, Jorge Guzman, and Maria P. Roche. "Incentivizing Innovation in Open Source: Evidence from the GitHub Sponsors Program." Harvard Business School Working Paper, No. 24-014, September 2023. (NBER Working Paper Series, No. 31668, September 2023.) View Details
- Brown, Daniel Jay, and Maria P. Roche. "Learning to Use: Stack Overflow and Technology Adoption." Harvard Business School Working Paper, No. 24-001, July 2023. View Details
- Marinoni, Astrid, and Maria P. Roche. "You've Got Mail! The Late 19th-Century U.S. Postal Service Expansion, Firm Creation, and Firm Performance." Harvard Business School Working Paper, No. 23-022, October 2022. (Revised July 2024.) View Details
- Conti, Annamaria, Christian Peukert, and Maria Roche. "Beefing IT Up for Your Investor? Open Sourcing and Startup Funding: Evidence from GitHub." Harvard Business School Working Paper, No. 22-001, July 2021. (Revised August 2023.) View Details
- Thursby, Jerry, Marie Thursby, Karim R. Lakhani, Kyle R. Myers, Nina Cohodes, Sarah Bratt, Dennis Byrski, Hannah Cohoon, and Maria Roche. "Scientific Production: An Exploration into Organization, Resource Allocation, and Funding." Working Paper, May 2020. View Details
- Roche, Maria P., Carlota Moniz, and Daniela Beyersdorfer. "ZEISS: Commercializing Science." Harvard Business School Case 725-359, July 2024. View Details
- Nagle, Frank, and Maria P. Roche. "CoPilot(s): Generative AI at Microsoft and GitHub." Harvard Business School Teaching Note 724-452, March 2024. View Details
- Nagle, Frank, Shane Greenstein, Maria P. Roche, Nataliya Langburd Wright, and Sarah Mehta. "Copilot(s): Generative AI at Microsoft and GitHub." Harvard Business School Case 624-010, November 2023. View Details
- Zuzul, Tiona, Maria P. Roche, and Exequiel Hernandez. "The Globalization of Manchester City Football Group." Harvard Business School Teaching Note 724-451, February 2024. View Details
- Roche, Maria P. "Moving Science: The Rowland Institute at Harvard." Harvard Business School Case 724-441, February 2024. (Revised May 2024.) View Details
- Roche, Maria P. "Accelerating AI Adoption in the U.S. Air Force." Harvard Business School Teaching Note 724-386, September 2023. (Revised May 2024.) View Details
- Roche, Maria P., and Alexander Farrow. "Accelerating AI Adoption in the U.S. Air Force." Harvard Business School Case 723-429, March 2023. (Revised March 2024.) View Details
- Roche, Maria P., Tiona Zuzul, Exequiel Hernandez, and Amy Klopfenstein. "The Globalization of Manchester City Football Group." Harvard Business School Case 723-391, November 2022. (Revised February 2024.) View Details
- Roche, Maria P. "Walmart USA—Searching for Growth." Harvard Business School Teaching Note 723-390, November 2022. (Revised May 2024.) View Details
- Montgomery, Cynthia A., and Maria Roche. "Assets, Capabilities, and Other Resources." Harvard Business School Background Note 722-392, March 2022. View Details
- Oberholzer-Gee, Felix, and Maria P. Roche. "Walmart USA—Searching for Growth." Harvard Business School Case 722-395, January 2022. View Details
Runner-up for the Best Paper in Innovation and Entrepreneurship at the 2024 Industry Studies Association Annual Conference for “"You've Got Mail! The Late 19th Century US Postal Service Expansion, Firm Creation, and Firm Performance” with Astrid Marinoni.
Recipient of the 2023 Outstanding Reviewer Award from Organization Science .
Winner of the 2022 Strategy Science Conference Best Paper Award for “Beefing IT Up for Your Investor? Open Sourcing and Startup Funding: Evidence from GitHub” with Annamaria Conti and Christian Peukert.
Winner of the 2021 Grigor McClelland Doctoral Dissertation Award from the Society for the Advancement of Management Studies and the Journal of Management Studies .
Winner of the 2021 Best Dissertation Award from the Technology and Innovation Management (TIM) Division of the Academy of Management.
Recipient of a NSF Science of Science and Innovation Policy Doctoral Dissertation Research Improvement Grant, National Science Foundation, 2019–2021.
Winner of the Ewing Marion Kauffman Best Student Paper Award at the 19th Annual Roundtable for Engineering Entrepreneurship Research (REER) Conference in 2019.
Winner of the 2019 Ashford Watson Stalnaker Memorial Award from the Scheller College of Business at Georgia Tech.
Curriculum Vitae
- entrepreneurship
- knowledge management
- human resource management
- technology strategy
- biotechnology
- education industry
- high technology
In The News
- 26 Jul 2024
- HBS Working Knowledge
Why Great Ideas Get Stuck in Universities
- 17 Jul 2024
- MIT Sloan School of Management
Close Proximity Is the Key to Knowledge Spillovers Among Startups
- 09 Jul 2024
Chance Encounters: What's at Stake in Return-to-Office Decisions
- 02 Jul 2024
- The Associated Press
AI Is Learning From What You Said on Reddit, Stack Overflow or Facebook. Are You Ok With That?
- 01 Jun 2024
- Harvard Business School
The Exchange: Chance Encounters
Maria p. roche in the news.
26 Jul 2024 HBS Working Knowledge Why Great Ideas Get Stuck in Universities
17 Jul 2024 MIT Sloan School of Management Close Proximity Is the Key to Knowledge Spillovers Among Startups
09 Jul 2024 HBS Working Knowledge Chance Encounters: What's at Stake in Return-to-Office Decisions
02 Jul 2024 The Associated Press AI Is Learning From What You Said on Reddit, Stack Overflow or Facebook. Are You Ok With That?
01 Jun 2024 Harvard Business School The Exchange: Chance Encounters
03 May 2024 HBS Working Knowledge How Much Does Proximity Influence Startup Innovation? 20 Meters' Worth to Be Exact
02 May 2024 NBER Bulletin on Entrepreneurship Open-Source Software Creators: It’s Not Just About the Money
30 Jan 2024 HBS Working Knowledge ‘Intrinsic Joy’ Sparks Ideas Better than Cash
24 Oct 2023 Cold Call How the United States Air Force Accelerated AI Adoption
16 Nov 2022 Harvard Business School Behind the Research: Maria Roche
01 Oct 2022 NBER Bulletin on Entrepreneurship Startup Chemistry and the Coworking Environment
16 Sep 2022 Handelsblatt Global Der ungehobene Schatz: Warum gute Ideen in Unternehmen oft untergehen
24 Aug 2022 WSJ What's News Podcast The War in Ukraine, Six Months Later
21 Aug 2022 Wall Street Journal How Co-Working Spaces Lead to More Innovation for Tech Startups
17 Aug 2022 Matt Clancy Innovation at the Office
09 Feb 2022 Harvard Business Review What’s the Optimal Workplace for Your Organization?
06 Dec 2021 HBS Alumni Bulletin Research Brief: Launching Into a Downturn
19 Sep 2021 Scientific Sense Prof. Maria Roche of Harvard Business School on the Impact of Inter-Personal Exchange on Innovation
02 Feb 2021 Matt Clancy Cities as a Platform to Mix Up Knowledge
22 Sep 2020 HBS Working Knowledge Recessions Push Some Entrepreneurs to Launch Too Soon
01 Nov 2019 Bloomberg The Particular Creativity of Dense Urban Neighborhoods
Areas of Interest
Additional topics.
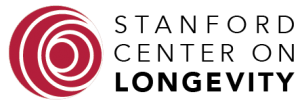
LIFESTYLE MEDICINE TEAM
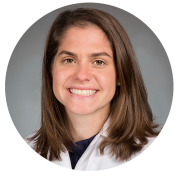
Megan Roche, MD PhD Candidate, Epidemiology Stanford University School of Medicine Fellow, Stanford Center on Longevity
Megan Roche received her medical degree from Stanford University in 2018. She is completing a PhD in Epidemiology and Population Health at Stanford with a clinical research focus on bone health in athletes and genetic predictors of sports injury. In addition, Megan is a post-doctoral research fellow at the Stanford Center on Longevity and is helping launch Stanford’s Lifestyle Medicine Initiative.
Megan is a five-time national trail running champion, a North American Mountain Running Champion, and a six-time member of Team USA. She is a co-author of the book “The Happy Runner” and a co-founder of Some Work All Play, a coaching group centered around finding long-term fulfillment in the process of running. Megan completed her undergraduate degree in neuroscience at Duke University and competed in varsity field hockey, cross country, and track and field.
“I became interested in lifestyle medicine as a result of working with patients who were motivated to start fitness routines. Fitness can be complex, and I realized that breaking fitness routines down into smaller goals centered around daily movement can be empowering for patients. I am also inspired by how a small change in one area in life can motivate change in other areas. Fitness progress can cascade into sleep improvements, which can optimize stress management. The cascade effect is possible across any number of lifestyle behaviors!”

Get the Reddit app
- About Roche
- Sustainability
- Focus areas
- Pharma solutions
- Diagnostic solutions
- Team & structure
- Innovation process
- Ethical standards
Future cures are in our genes
This is the present – and promise – of gene therapy. But gene-based therapy opens even more possibilities, and almost unlimited potential, beyond rare genetic disorders.
“It’s a new frontier,” says James Sabry, Global Head of Roche Pharma Partnering. “In many ways, the limitations are only what scientists can think of, and that’s really where the value of collaboration across Roche and with our current and future partners comes in.”
This mindset led to the acquisition of Spark Therapeutics, the Philadelphia-based gene therapy center of excellence within Roche.
“Imagine a world where no life is limited by genetic disease and the ambition of gene therapy to treat or possibly prevent disease is realized for the millions of people living with an inherited condition,” says Jeff Marrazzo, CEO and co-founder of Spark Therapeutics. “By addressing some diseases at a genetic level, entire lives, families and our global healthcare system could be transformed by unlocking the full potential of gene therapy.”
The next generation of pioneering medicines, with gene therapy front and centre, is blazing a path towards truly personalised global healthcare. It’s a proactive approach, strengthened by partnering and collaboration, that seeks better healthcare at a lower cost to society.
First, a bit about genes
To understand gene therapy, we should start with genes. Cells are the basic units of life, and each cell contains a full set of instructions, or genes. Each gene encodes for a different protein, or the “business ends” of the cell and the regulatory instructions of when to use this gene. Cells in our body are different, and each cell uses only some of the genes based on its needs – immune cells will need genes for proteins like antibodies to fight off infections, brain cells will need the genes that encode neurotransmitters, and so on.
But sometimes our genes have a glitch in the code, either one that we inherit from our parents at birth, or one that arises within our own bodies over time, and that can cause disease, because the cells cannot work properly. In some inherited cases, the error is major, and will cause a severe disease like haemophilia or Huntington’s disease. In other cases, the differences we inherit are part of the natural variation between humans, but can increase our risk of common diseases, like heart disease or type 2 diabetes. But genes can also mutate during our lifetime, especially due to errors accrued over the lifespan, resulting in illnesses, especially cancer.
In the future, instead of a series of traditional medical treatments that only manage some diseases – or a lifetime of injections, infusions, monitoring, adjustments and ongoing doctor appointments – patients might instead receive gene therapy treatment to fix that glitch.
Learn more about genes and gene therapy in this video
How do genes in our cells instruct the body to work? Imagine our genes as “recipes” in a cookbook. Learn more!
A tough trail ahead
While the prospects of gene therapy are awe-inspiring, it won’t be easy or instantaneous. Before we ride off into a sunset with great therapies for genetic disease, there are challenges to overcome. Some aspects of the science behind gene therapy are uncharted; there will be stumbles, disappointments and setbacks.
Even when solutions are found in a laboratory, in the form of novel vectors or “messengers” that carry new genetic instructions to block, take down or take over for malfunctioning ones, those breakthroughs must then be meticulously translated to humans and into large-scale production processes that can repeatedly and reliably produce the same medicines in manufacturing facilities.
In some cases, the future is now. In 2019 Spark Therapeutics, located in Philadelphia, became the gene therapy center of excellence within Roche. The Roche Group’s bold commitment to gene therapy collaborations across the organisation and industry are a stake in the ground: the possibility and potential of using genes to treat or prevent disease now and in the future is one clear path to where the Roche Group and healthcare are headed.
Oftentimes, the many remaining challenges can be solved by bringing together different scientists and approaches in a partnership. For example, Roche entered into two collaborations in 2020 that focus on solving two parts of the puzzle. Roche and Spark Therapeutics are working with Dyno Therapeutics to engineer optimised viral capsids (think of tiny packages that protect, transport and deliver genetic solutions,) with the help of machine learning applied to biology. And a partnership with CEVEC Pharmaceuticals will leverage their expertise in high-performance viral vector production. Viral vectors are tools that deliver genetic material into cells, and this partnership could enable large-scale biopharmaceutical manufacturing of those vectors.
Making our vision a reality
“I believe that within 20 or 30 years from now, gene therapy will represent the most important class of pioneering medicines in our industry,” James says. “The reason for that is clear: if you can go in and do genetic surgery – by removing malfunctioning or abnormally regulated genes and replacing them with genes that are normally regulated – then you can get to the basis of what causes disease and fix it. It's version 1.0 right now for gene therapy, but we're very excited about the future, and our partnership with trailblazing companies like Spark make this vision a reality.”
Already today, there is initial evidence that gene therapy could change the lives of patients with certain diseases by introducing a working gene to step in and do the job of a malfunctioning one. But in versions 2.0 or 3.0, gene therapy would involve taking out one gene and replacing it with another, like surgery at a genetic level, or by adding a new gene to produce a protein that is therapeutic, like a vectorised antibody medicine against a target that causes disease.
Spark Therapeutics was the first company in the U.S. to launch a gene therapy, backed by its robust research, commercial and manufacturing capabilities. After a long courtship, Roche and Spark aligned their . After the December 2019 acquisition, Spark remains autonomous in Philadelphia, helmed by Jeff, who co-founded the company eight years ago. Chief Scientific Officer Federico Mingozzi leads further discovery and pre-clinical development of novel gene therapies.
Ultimately, the future of medicine will be a combination of complementary approaches, Federico says, including small molecules, large molecules, and programmable therapies that include gene therapy, cell therapy, bacterial therapies and RNA-based therapies. Spark Therapeutics is an integral part of that within Roche.
“There was enormous excitement in the company after the acquisition,” Federico says. “We're still working hard to develop gene therapies, but we are also collaborating more and more with our colleagues across Roche. This is fantastic, because it creates novel opportunities to develop life-changing medicines. Combining capabilities is a great way to speed up the development of new therapies to bring to patients. That's the part of my job that I love, and that is what brings me to work every day.”
A glimpse of the future
Our scientists share their vision and hope for the unlimited possibilities and potential of gene therapy
Azad Bonni, Global Head of Neuroscience and Rare Diseases, Roche pRED
“With Huntington’s disease, for instance, gene therapy might lead to faster and wider access to the target – the root cause of that specific disease. And that speed and accuracy in getting to the target might result in slowing the disease. The potential of slowing a disease early, or before it even begins, is indeed exciting.”
Aviv Regev, Global Head, Genentech gRED
“Gene therapy is a ‘programmable’ therapy that allows us to rewrite the cellular program. For this we need great targets, and a great understanding of which cells in which tissue to target in a disease. The scientists at Genentech Research and Early Development (gRED) leverage strengths across all these areas, and powerful computational approaches like machine learning to help us get there faster.”
Sylke Poehling, SVP, Global Head, Therapeutic Modalities, Roche pRED
“Gene therapy is a cornerstone of our vision for the future, and it creates almost unlimited possibilities for our scientists to deliver new transformative medicines. Combining our strengths with partners and collaborators who share our vision and passion of targeting disease at a genetic level, we will hopefully change healthcare – and global health – for the better.”
Barbara Lueckel, Global Head of Research Technologies, Roche Pharma Partnering
"To advance science we need to think about the impossible. By connecting the brightest minds in Roche and in the biotechs we partner with, we are continuously pushing the boundaries to find innovative solutions to the challenges we need to overcome to hopefully make gene therapy a reality for a broad range of patients."
Federico Mingozzi, Chief Scientific Officer, Spark Therapeutics
“The life-changing goal with gene therapies is to create a single therapeutic intervention that is curative. That is absolutely transformative when it comes to the potential impact on the lives of patients. Emerging data on the long-term safety and efficacy of gene therapy patients is promising, as it shows that it is possible to achieve a durable effect over time. The potential of curing and preventing disease, versus treating or maintenance, is our passion and what drives us forward.”
How gene therapy can help
Today’s healthcare uses traditional drugs that target proteins to manage or treat disease. But gene therapy impacts the genes that code the proteins, and in this way uses genes to treat or someday even prevent disease.
Genes can be delivered to cells in different ways. From the beginning, Spark Therapeutics has focused on adeno-associated virus (AAV) vectors, which are derived from a virus found in nature. Viral vectors harness the innate ability of a virus to enter cells, carrying the therapeutic gene with them. AAVs are an attractive option as a “messenger” to deliver genes, as they are considered safe, stable and keep cells intact.
One day, gene therapy could become a common approach to use the human body itself as a manufacturing site for therapeutic proteins that we design. Instead of companies like Roche manufacturing proteins in a large-scale, high-tech production plant, protein therapies would go hyper-local and could be “manufactured” in your liver, for instance, or in the glial cells in your brain.
“What gene therapy allows us to do, potentially, is to use the human body, as our own specialised biofactory – that is, we can use programmed genes for the production of protein and antibody treatments against chronic diseases,” says Sylke Poehling, Senior Vice President, Global Head, Therapeutic Modalities, Roche Pharma Research & Early Development. “These are the ways we're thinking about how we can all come together to complement and push the boundaries of what we're currently doing.”
Prevention as an investment
Beyond the push toward next-level science, pioneering medicines also require a shift in mindset, in terms of costs, to move from reactive healthcare solutions to a proactive one, like gene therapy. Most health systems today diagnose and treat patients in later stages of disease and are built on treatments for chronic conditions.
“This happens if you have a coronary artery bypass graft, or have your appendix removed via surgery, but in the future it will be genetic surgery and it can happen earlier,” says William Pao, Global Head, Roche Pharma Research & Early Development. “That expands what we think of as a drug, and has huge implications for the healthcare system in terms of ease of use and access to the therapy, and also in terms of economics of the therapy.”
The cost of healthcare – not only financial but also in quality of life for the patient – is traditionally borne over years (sometimes decades) of treatments or anatomic surgery. Gene therapy can potentially flip that script, putting the cost of diagnosis, cure or prevention up-front, as an investment in fewer chronic diseases and better quality of life in the future. This will require collaboration with health systems across the globe to allow for innovative payment models that ensure the promising science of gene therapy can ultimately reach patients.
"We envision a world where no life is limited by genetic disease, and reaching that point requires us to unlock the full potential of gene therapy,” Jeff says.
“To do that, we're exploring ways to broaden our current pipeline, which is focused on monogenic diseases – diseases caused by a variation in a single gene – and on three main target tissues: the eye, the liver and the central nervous system,” Federico says.
The goal for the future? Expansion not only to other therapeutic areas, but also to more complex diseases, where multiple genes each contribute a small portion of risk, based on variants common in the human population. The bottom line, Federico says, is to bring more gene therapies to more patients to transform clinical outcomes, and ultimately transform healthcare for the better.
Gene therapy center of excellence
Through an integrated gene therapy platform, is a fully integrated, commercial company that works to turn genes into medicines for patients with inherited diseases, including retinal diseases and liver-directed diseases such as hemophilia and lysosomal storage disorders, and neurodegenerative diseases, using adeno-associated viral vectors. Spark has four programs in clinical trials and is creating the path to a world where no life is limited by genetic disease.
Pioneering partnerships
Meet some of our partners who share our vision for transforming healthcare through gene therapy.
: Using artificial intelligence to engineer next-generation adeno-associated virus vectors, and partnering across Roche and Spark to allow development of gene therapy for larger patient populations.
: A global biotechnology company, partnering with Roche on an urgent mission: to engineer precision genetic medicine for rare diseases that devastate lives and cut futures short, including Duchenne muscular dystrophy.
Be part of the future
Roche is committed to driving groundbreaking scientific and technological advances that have the potential to transform the lives of patients worldwide. But we Learn why we are the No. 1 partner of choice, and what we look for in our partners and our , including reduction/elimination of immunogenicity, cell-specific promoters, circuits for cell-specific expression, gene regulation technologies, non-viral delivery, and gene therapy assets, especially in neuroscience, ophthalmology and rare diseases.
Discover more
More stories of.
This website contains information on products which is targeted to a wide range of audiences and could contain product details or information otherwise not accessible or valid in your country. Please be aware that we do not take any responsibility for accessing such information which may not comply with any legal process, regulation, registration or usage in the country of your origin.
Welcome to our S tudent and Graduate page
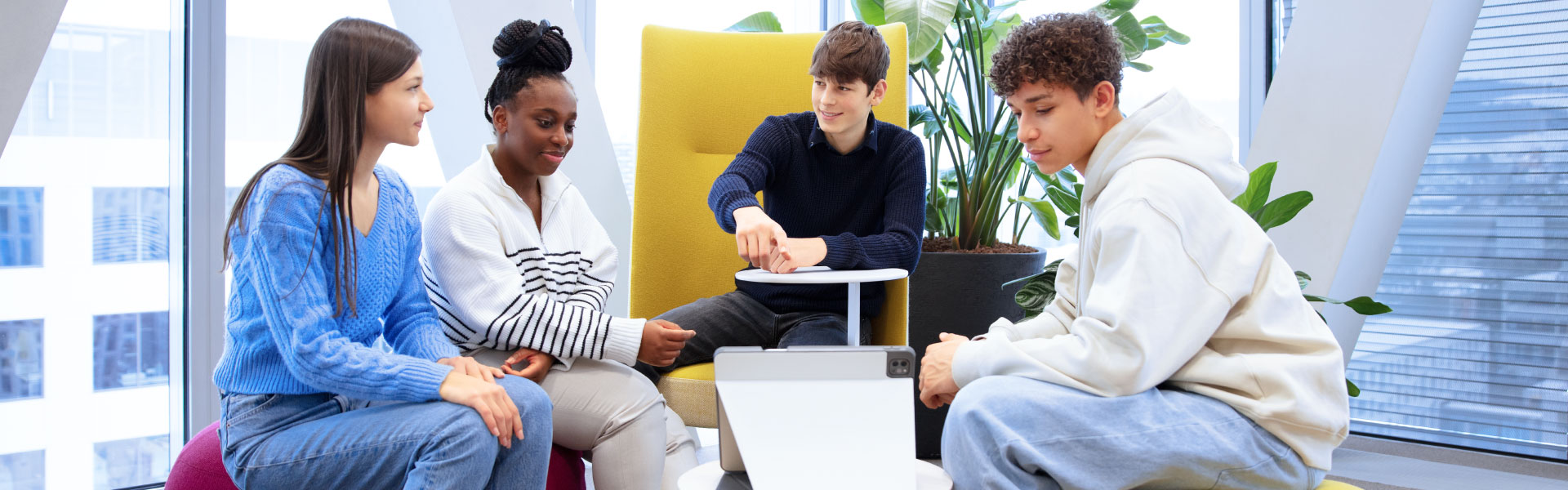
Are you curious to know more about the different options to start your career at Roche?
We offer a large variety of programmes, whether you are looking for an apprenticeship after leaving school, an internship during studies or a traineeship after your degree course, you have a place at roche, choose your area of interest below and check the available opportunities, this page provides information on our structured early in career programmes. some programmes are global and some of them are location specific, please see programme details for more information in the drop-down section. however, we do hire early in career talent all year round, please click here to view our current openings. , vocational educational training, we prioritize vocational education and training to empower our success. choose from a range of apprenticeship programmes and dual study opportunities in various fields. explore the possibilities in your country below, are you a talented university student who is interested in gaining professional experience and insights into a global healthcare company during your studies or gap year roche has an array of internship programmes and part-time or ad-hoc intern opportunities for students like you. explore the possibilities at roche today, recent graduates, are you a soon-to-be graduate looking for an exciting career in the healthcare industry roche has the perfect opportunities for you with traineeships, rotational programmes, part-time roles, and direct-entry positions tailored for recent graduates, you can dive into the heart of a global healthcare company and set the stage for a promising future. explore the diverse opportunities at roche and launch your career with us today, experienced graduates, roche's transformative programmes for experienced graduates: international rotations, dynamic roles, and impactful projects. connect with influential leaders, receive tailored training, and thrive in the multinational realm. ignite your career with roche today, you don’t find what you are looking for now are you not ready to start your career yet then join our talent community learn more about how to join by scrolling to the bottom of the page., apprenticeships, dual studies, internship programmes, ad-hoc internships and part time opportunities, recent graduates, graduate programmes, doctoral thesis / phd, postdoc fellowship, entry-level positions, perspectives programmes.
- Develop a comprehensive understanding of Roche during two years
- Immerse yourself in four exciting assignments around the world
- Collaborate with inspiring senior leaders throughout the organization
- Intentionally craft your journey within the programme and beyond

IMAGES
VIDEO
COMMENTS
Graduate Programmes. Level up your career with Roche's trainee programmes! Whether you have a bachelor's, master's, or PhD degree, we offer practical work experience for 2-3 years. Discover a world of opportunities with national and international rotations, functional role shifts, and entry-level positions. Dive into diverse, challenging, and ...
The Roche Postdoctoral Fellowship programme was established within Roche to build and maintain the Scientific Leadership of the future. The programme provides leading internal scientists with the opportunity to collaborate with talented postdoctoral researchers, and leading academic research groups, from around the world. More specifically, the ...
Psychological causes and factors relevant to the development, maintenance and improvement of formal thought disorder in psychosis (Project ID SHSC0057) Thought disorder is a commonly occurring symptom in individuals diagnosed with a psychotic illness. It can take many forms, all of which profoundly affect the individual's ability to ...
The joint doctoral programme Translational Engineering of Biological Systems of Roche's Institute of Human Biology and the ETH Zurich Department of Biosystems Science and Engineering D-BSSE offers a unique opportunity for scientific training in translational human biology and medicine. Doctoral students will conduct highly innovative and collaborative research projects and become the next ...
The Roche Postdoctoral Fellowship (RPF) Programme. Internally known as RPF, this programme aims to build scientific leadership by expanding and strengthening our scientific exchange and collaboration with academia. Specifically, we offer talented Postdoctoral graduates the opportunity to join us as fellows working on a specific topic, co ...
Roche partnered with ETH Zurich to enable PhD students and postdoctoral scientist from developing countries to attend the 14th ICOBTE held in Zurich. ... At Roche we are committed to creating and maintaining a healthy and safe environment for all. Protecting people and the environment isn't just a legal or social obligation; it is integral to ...
Modeling the future. Roche launches the Institute of Human Biology to pioneer new approaches for drug discovery and development. Discovering and developing new medicines is critical to benefit society — but unfortunately, it's also challenging, costly, and slow. Many great ideas that do well in early stages of testing don't pan out in ...
Ira Mellman, PhD - Vice President, Cancer Immunology, Roche - is a scientist, a member of the National Academy of Sciences and former Yale Professor. Dan Chen, MD, PhD - Vice President, Global Head of Cancer Immunotherapy Development, Roche - is an oncologist and former Howard Hughes Medical Institute Associate who ran the Stanford ...
PURPOSE Immune gene expression signatures are emerging as potential biomarkers for immunotherapy (IO). VIGex is a 12-gene expression classifier developed in both nCounter (Nanostring) and RNA sequencing (RNA-seq) assays and analytically validated across laboratories. VIGex classifies tumor samples into hot, intermediate-cold (I-Cold), and cold subgroups. VIGex-Hot has been associated with ...
We are delighted to share that COAH Core Faculty member Dr. Karen Bandeen-Roche has been named our 2024 Champion. She is a Professor and Emeritus Chair of the Department of Biostatistics at the Bloomberg School of Public Health. Also, Dr. Bandeen-Roche holds joint appointments in Medicine and Nursing. Dr.
Academic minds for innovation wanted! Solving real business challenges around data, gaining insights into the world of Roche, realizing ideas together that improve patients' lives: this is Healthcare Xplorers, our new innovation platform for undergraduate and graduate students in bioinformatics, business mathematics, physics and engineering.
Target Audience and Goal Statement. This activity is intended for neurologists, primary care physicians, and geriatricians (excluding the United Kingdom).
In human and murine adipocytes, a PHD inhibitor increases Ucp1 levels. In murine brown adipocytes, antagonising the major PHD2 target, hypoxia-inducible factor-(HIF)−2a abolishes Ucp1 that ...
Syllabus/Timetable/Learning Goals/Rationale and Objective are here. Organic 1 lecture course is 4 credits (lab is still 1 credit). All ORGANIC LAB questions should be directed to Ms. Bailey Blessing ( [email protected]) Clickable Lecture Notes are based on the excellent books "Organic Chemistry" by L.G. Wade, Jr., 4th…9th eds.
Senior Scientist - PhD with 6 years postdoc experience - Senior Scientist Roche Employee Review. See All Reviews See All . 5.0. Mar 21, 2022. Senior Scientist. Current Employee, less than 1 year. Basel. Recommend. CEO Approval. Business Outlook. Pros. Benefits are plus. So many benefits.
What: Perform your own exploratory research embedded in a research, clinical or analytical team within drug development programmes. Duration: 9 to 12 months. Location: Roche Innovation Center Basel, Switzerland. Therapeutic areas: Neuroscience and Rare Diseases here. Career benefits: immersed in a global pharmaceutical research environment you ...
Paul Strijbos, Neurotech Innovation Leader, Product Development at Roche is one of many scientists using breakthrough technology to reduce patient burden and more accurately collect data for people living with this rare disease. This novel technology is "a real breakthrough in terms of the way we work with patients and is changing the way ...
Professor Roche earned her PhD in Management (Strategy and Innovation) at the Scheller College of Business, Georgia Institute of Technology, where she was a recipient of a NSF Science of Science and Innovation Policy Doctoral Dissertation Research Improvement Grant. She earned an MS in Business Administration and a BA in International Cultural ...
Megan Roche received her medical degree from Stanford University in 2018. She is completing a PhD in Epidemiology and Population Health at Stanford with a clinical research focus on bone health in athletes and genetic predictors of sports injury. In addition, Megan is a post-doctoral research fellow at the Stanford Center on Longevity and is ...
List of La Roche University graduate programs by size and degree. Browse popular PhD programs at La Roche University. Find on-campus and online graduate programs at La Roche University.
Graduates & Research Fellowships. Our Graduate and Postgraduate opportunities are for those who have recently graduated (0-2 years) from their degrees (Undergraduate, Masters, PhD), and want to take their first steps within industry. Our Research and project based opportunities are available for those wanting to continue research, such as ...
In the meantime I applied to both PhDs and industry roles and got an offer for a PhD in Amsterdam (Netherlands has a surprisingly good salary for PhD students in comparison with other European countries) as well as another one for the role of RA at a big pharma in Switzerland - Roche.
Spark Therapeutics was the first company in the U.S. to launch a gene therapy, backed by its robust research, commercial and manufacturing capabilities. After a long courtship, Roche and Spark aligned their. After the December 2019 acquisition, Spark remains autonomous in Philadelphia, helmed by Jeff, who co-founded the company eight years ago.
Graduates. Are you about to complete your Bachelor or Master degree, are you looking for a PhD position, or has your PhD thesis been defended and you are looking for a post-doctoral position? Whether in a trainee or post-doc programme or as a direct entrant, together we can ensure a successful start of your career.
Explore our tech opportunities today! Roche is at the forefront of technological advancements in healthcare. Elevate your career with us by joining our tech team. Whether you're a software developer, data analyst, or IT expert, Roche provides a platform for you to innovate and make a lasting impact on global healthcare.
Dr. Roche (she/her/hers) specializes in treating and supporting busy professionals and their families who expect a sophisticated approach. She has over 30 years of experience providing a broad range of high quality services to adults, couples, children, adolescents, and families. Roche + Associates was established in 2012.
Roche's Perspectives Programmes are a career accelerator and a springboard for talents with future-relevant skills to tackle the world's most formidable healthcare challenges.