- Search Menu
- Sign in through your institution
- Volume 12, Issue 1, 2024 (In Progress)
- Volume 11, Issue 1, 2023
- Advance articles
- Editor's Choice
- Virtual Issues
- Clinical Briefs
- ISEMPH Prizes
- Why Publish
- Author Guidelines
- Submission Site
- Open Access
- Calls for Papers
- About Evolution, Medicine, and Public Health
- About the International Society for Evolution, Medicine and Public Health
- Editorial Board
- Advertising and Corporate Services
- Journals Career Network
- Self-Archiving Policy
- For Reviewers
- Journals on Oxford Academic
- Books on Oxford Academic
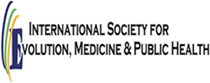

Article Contents
Introduction, human enhancement, genetic engineering, conclusions.
- < Previous
Human enhancement: Genetic engineering and evolution
- Article contents
- Figures & tables
- Supplementary Data
Mara Almeida, Rui Diogo, Human enhancement: Genetic engineering and evolution, Evolution, Medicine, and Public Health , Volume 2019, Issue 1, 2019, Pages 183–189, https://doi.org/10.1093/emph/eoz026
- Permissions Icon Permissions
Genetic engineering opens new possibilities for biomedical enhancement requiring ethical, societal and practical considerations to evaluate its implications for human biology, human evolution and our natural environment. In this Commentary, we consider human enhancement, and in particular, we explore genetic enhancement in an evolutionary context. In summarizing key open questions, we highlight the importance of acknowledging multiple effects (pleiotropy) and complex epigenetic interactions among genotype, phenotype and ecology, and the need to consider the unit of impact not only to the human body but also to human populations and their natural environment (systems biology). We also propose that a practicable distinction between ‘therapy’ and ‘enhancement’ may need to be drawn and effectively implemented in future regulations. Overall, we suggest that it is essential for ethical, philosophical and policy discussions on human enhancement to consider the empirical evidence provided by evolutionary biology, developmental biology and other disciplines.
Lay Summary: This Commentary explores genetic enhancement in an evolutionary context. We highlight the multiple effects associated with germline heritable genetic intervention, the need to consider the unit of impact to human populations and their natural environment, and propose that a practicable distinction between ‘therapy’ and ‘enhancement’ is needed.
There are countless examples where technology has contributed to ameliorate the lives of people by improving their inherent or acquired capabilities. For example, over time, there have been biomedical interventions attempting to restore functions that are deficient, such as vision, hearing or mobility. If we consider human vision, substantial advances started from the time spectacles were developed (possibly in the 13th century), continuing in the last few years, with researchers implanting artificial retinas to give blind patients partial sight [ 1–3 ]. Recently, scientists have also successfully linked the brain of a paralysed man to a computer chip, which helped restore partial movement of limbs previously non-responsive [ 4 , 5 ]. In addition, synthetic blood substitutes have been created, which could be used in human patients in the future [ 6–8 ].
The progress being made by technology in a restorative and therapeutic context could in theory be applied in other contexts to treat non-pathological conditions. Many of the technologies and pharmaceutical products developed in a medical context to treat patients are already being used by humans to ‘enhance’ some aspect of their bodies, for example drugs to boost brain power, nutritional supplements, brain stimulating technologies to control mood or growth hormones for children of short stature. Assistive technology for disabled people, reproductive medicine and pharmacology, beside their therapeutic and restorative use, have a greater potential for human ‘enhancement’ than currently thought. There are also dual outcomes as some therapies can have effects that amount to an enhancement as for example, the artificial legs used by the South African sprinter Oscar Pistorius providing him with a competitive advantage.
This commentary will provide general ethical considerations on human enhancement, and within the several forms of so-called human biomedical enhancement, it will focus on genetic engineering, particularly on germline (heritable) genetic interventions and on the insights evolutionary biology can provide in rationalizing its likely impact. These insights are a subject often limited in discussions on genetic engineering and human enhancement in general, and its links to ethical, philosophical and policy discussions, in particular [ 9 ]. The rapid advances in genetic technology make this debate very topical. Moreover, genes are thought to play a very substantial role in biological evolution and development of the human species, thus making this a topic requiring due consideration. With this commentary, we explore how concepts based in evolutionary biology could contribute to better assess the implications of human germline modifications, assuming they were widely employed. We conclude our brief analysis by summarizing key issues requiring resolution and potential approaches to progress them. Overall, the aim is to contribute to the debate on human genetic enhancement by looking not only at the future, as it is so often done, but also at our evolutionary past.
The noun ‘enhancement’ comes from the verb ‘enhance’, meaning ‘to increase or improve’. The verb enhance can be traced back to the vulgar Latin inaltiare and late Latin inaltare (‘raise, exalt’), from ‘ altare ’ (‘make high’) and altus (‘high’), literally ‘grown tall’. For centuries human enhancement has populated our imagination outlined by stories ranging from the myths of supernormal strengths and eternal life to the superpowers illustrated by the 20th century comic books superheroes. The desire of overcoming normal human capacities and the transformation to an almost ‘perfect’ form has been part of the history of civilization, extending from arts and religion to philosophy. The goal of improving the human condition and health has always been a driver for innovation and biomedical developments.
In the broadest sense, the process of human enhancement can be considered as an improvement of the ‘limitations’ of a ‘natural version’ of the human species with respect to a specific reference in time, and to different environments, which can vary depending on factors such as, for example, climate change. The limitations of the human condition can be physical and/or mental/cognitive (e.g. vision, strength or memory). This poses relevant questions of what a real or perceived human limitation is in the environment and times in which we are living and how it can be shifted over time considering social norms and cultural values of modern societies. Besides, the impact that overcoming these limitations will have on us humans, and the environment, should also be considered. For example, if we boost the immune system of specific people, this may contribute to the development/evolution of more resistant viruses and bacteria or/and lead to new viruses and bacteria to emerge. In environmental terms, enhancing the longevity of humans could contribute to a massive increase in global population, creating additional pressures on ecosystems already under human pressure.
Two decades ago, the practices of human enhancement have been described as ‘biomedical interventions that are used to improve human form or functioning beyond what is necessary to restore or sustain health’ [ 10 ]. The range of these practices has now increased with technological development, and they are ‘any kind of genetic, biomedical, or pharmaceutical intervention aimed at improving human dispositions, capacities, or well-being, even if there is no pathology to be treated’ [ 11 ]. Practices of human enhancement could be visualized as upgrading a ‘system’, where interventions take place for a better performance of the original system. This is far from being a hypothetical situation. The rapid progress within the fields of nanotechnology, biotechnology, information technology and cognitive science has brought back discussions about the evolutionary trajectory of the human species by the promise of new applications which could provide abilities beyond current ones [ 12 , 13 ]. If such a possibility was consciously embraced and actively pursued, technology could be expected to have a revolutionary interference with human life, not just helping humans in achieving general health and capabilities commensurate with our current ones but helping to overcome human limitations far beyond of what is currently possible for human beings. The emergence of new technologies has provided a broader range of potential human interventions and the possibility of transitioning from external changes to our bodies (e.g. external prosthesis) to internal ones, especially when considering genetic manipulation, whose changes can be permanent and transmissible.
The advocates of a far-reaching human enhancement have been referred to as ‘transhumanists’. In their vision, so far, humans have largely worked to control and shape their exterior environments (niche construction) but with new technologies (e.g. biotechnology, information technology and nanotechnology) they will soon be able to control and fundamentally change their own bodies. Supporters of these technologies agree with the possibility of a more radical interference in human life by using technology to overcome human limitations [ 14–16 ], that could allow us to live longer, healthier and even happier lives [ 17 ]. On the other side, and against this position, are the so-called ‘bioconservatives’, arguing for the conservation and protection of some kind of ‘human essence’, with the argument that it exists something intrinsically valuable in human life that should be preserved [ 18 , 19 ].
There is an ongoing debate between transhumanists [ 20–22 ] and bioconservatives [ 18 , 19 , 23 ] on the ethical issues regarding the use of technologies in humans. The focus of this commentary is not centred on this debate, particularly because the discussion of these extreme, divergent positions is already very prominent in the public debate. In fact, it is interesting to notice that the ‘moderate’ discourses around this topic are much less known. In a more moderate view, perhaps one of the crucial questions to consider, independently of the moral views on human enhancement, is whether human enhancement (especially if considering germline heritable genetic interventions) is a necessary development, and represents an appropriate use of time, funding and resources compared to other pressing societal issues. It is crucial to build space for these more moderate, and perhaps less polarized voices, allowing the consideration of other positions and visions beyond those being more strongly projected so far.
Ethical and societal discussions on what constitutes human enhancement will be fundamental to support the development of policy frameworks and regulations on new technological developments. When considering the ethical implications of human enhancement that technology will be available to offer now and in the future, it could be useful to group the different kinds of human enhancements in the phenotypic and genetic categories: (i) strictly phenotypic intervention (e.g. ranging from infrared vision spectacles to exoskeletons and bionic limbs); (ii) somatic, non-heritable genetic intervention (e.g. editing of muscle cells for stronger muscles) and (iii) germline, heritable genetic intervention (e.g. editing of the C–C chemokine receptor type 5 (CCR5) gene in the Chinese baby twins, discussed later on). These categories of enhancement raise different considerations and concerns and currently present different levels of acceptance by our society. The degree of ethical, societal and environmental impacts is likely to be more limited for phenotypic interventions (i) but higher for genetic interventions (ii and iii), especially for the ones which are transmissible to future generations (iii).
The rapid advances in technology seen in the last decades, have raised the possibility of ‘radical enhancement’, defined by Nicholas Agar, ‘as the improvement of human attributes and abilities to levels that greatly exceed what is currently possible for human beings’ [ 24 ]. Genetic engineering offers the possibility of such an enhancement by providing humans a profound control over their own biology. Among other technologies, genetic engineering comprises genome editing (also called gene editing), a group of technologies with the ability to directly modify an organism’s DNA through a targeted intervention in the genome (e.g. insertion, deletion or replacement of specific genetic material) [ 25 ]. Genome editing is considered to achieve much greater precision than pre-existing forms of genetic engineering. It has been argued to be a revolutionary tool due to its efficiency, reducing cost and time. This technology is considered to have many applications for human health, in both preventing and tackling disease. Much of the ethical debate associated with this technology concerns the possible application of genome editing in the human germline, i.e. the genome that can be transmitted to following generations, be it from gametes, a fertilized egg or from first embryo divisions [ 26–28 ]. There has been concern as well as enthusiasm on the potential of the technology to modify human germline genome to provide us with traits considered positive or useful (e.g. muscle strength, memory and intelligence) in the current and future environments.
Genetic engineering: therapy or enhancement and predictability of outcomes
To explore some of the possible implications of heritable interventions we will take as an example the editing (more specifically ‘deletion’ using CRISPR genome editing technology) of several base pairs of the CCR5 gene. Such intervention was practised in 2018 in two non-identical twin girls born in China. Loss of function mutations of the CCR5 had been previously shown to provide resistance to HIV. Therefore, the gene deletion would be expected to protect the twin baby girls from risk of transmission of HIV which could have occurred from their father (HIV-positive). However, the father had the infection kept under control and the titre of HIV virus was undetectable, which means that risk of transmission of HIV infection to the babies was negligible [ 29 ].
From an ethical ground, based on current acceptable practices, this case has been widely criticized by the scientific community beside being considered by many a case of human enhancement intervention rather than therapy [ 29 , 30 ]. One of the questions this example helps illustrate is that the ethical boundary between a therapy that ‘corrects’ a disorder by restoring performance to a ‘normal’ scope, and an intervention that ‘enhances’ human ability outside the accepted ‘normal’ scope, is not always easy to draw. For the sake of argument, it could be assumed that therapy involves attempts to restore a certain condition of health, normality or sanity of the ‘natural’ condition of a specific individual. If we take this approach, the question is how health, normality and sanity, as well as natural per se, are defined, as the meaning of these concepts shift over time to accommodate social norms and cultural values of modern societies. It could be said that the difficulty of developing a conceptual distinction between therapy and enhancement has always been present. However, the potential significance of such distinction is only now, with the acceleration and impact of technological developments, becoming more evident.
Beyond ethical questions, a major problem of this intervention is that we do not (yet?) know exactly the totality of the effects that the artificial mutation of the CCR5 may have, at both the genetic and phenotypic levels. This is because we now know that, contrary to the idea of ‘one gene-one trait’ accepted some decades ago, a gene—or its absence—can affect numerous traits, many of them being apparently unrelated (a phenomenon also known as pleiotropy). That is, due to constrained developmental interactions, mechanisms and genetic networks, a change in a single gene can result in a cascade of multiple effects [ 31 ]. In the case of CCR5, we currently know that the mutation offers protection against HIV infection, and also seems to increase the risk of severe or fatal reactions to some infectious diseases, such as the influenza virus [ 32 ]. It has also been observed that among people with multiple sclerosis, the ones with CCR5 mutation are twice as likely to die early than are people without the mutation [ 33 ]. Some studies have also shown that defective CCR5 can have a positive effect in cognition to enhance learning and memory in mice [ 34 ]. However, it’s not clear if this effect would be translated into humans. The example serves to illustrate that, even if human enhancement with gene editing methods was considered ethically sound, assessing the totality of its implications on solid grounds may be difficult to achieve.
Genetic engineering and human evolution: large-scale impacts
Beyond providing the opportunity of enhancing human capabilities in specific individuals, intervening in the germline is likely to have an impact on the evolutionary processes of the human species raising questions on the scale and type of impacts. In fact, the use of large-scale genetic engineering might exponentially increase the force of ‘niche construction’ in human evolution, and therefore raise ethical and practical questions never faced by our species before. It has been argued that natural selection is a mechanism of lesser importance in the case of current human evolution, as compared to other organisms, because of advances in medicine and healthcare [ 35 ]. According to such a view, among many others advances, natural selection has been conditioned by our ‘niche-construction’ ability to improve healthcare and access to clean water and food, thus changing the landscape of pressures that humans have been facing for survival. An underlying assumption or position of the current debate is that, within our human species, the force of natural selection became minimized and that we are somehow at the ‘end-point’ of our evolution [ 36 ]. If this premise holds true, one could argue that evolution is no longer a force in human history and hence that any human enhancement would not be substituting itself to human evolution as a key driver for future changes.
However, it is useful to remember that, as defined by Darwin in his book ‘On the Origin of the Species’, natural selection is a process in which organisms that happen to be ‘better’ adapted to a certain environment tend to have higher survival and/or reproductive rates than other organisms [ 37 ]. When comparing human evolution to human genetic enhancement, an acceptable position could be to consider ethically sound those interventions that could be replicated naturally by evolution, as in the case of the CCR5 gene. Even if this approach was taken, however, it is important to bear in mind that human evolution acts on human traits sometimes increasing and sometimes decreasing our biological fitness, in a constant evolutionary trade-off and in a contingent and/or neutral—in the sense of not ‘progressive’—process. In other worlds, differently from genetic human enhancement, natural selection does not ‘ aim ’ at improving human traits [ 38 ]. Human evolution and the so-called genetic human enhancement would seem therefore to involve different underlying processes, raising several questions regarding the implications and risks of the latter.
But using genetic engineering to treat humans has been proposed far beyond the therapeutic case or to introduce genetic modifications known to already occur in nature. In particular, when looking into the views expressed on the balance between human evolution and genetic engineering, some argue that it may be appropriate to use genetic interventions to go beyond what natural selection has contributed to our species when it comes to eradicate vulnerabilities [ 17 ]. Furthermore, when considering the environmental, ecological and social issues of contemporary times, some suggest that genetic technologies could be crucial tools to contribute to human survival and well-being [ 20–22 ]. The possible need to ‘engineer’ human traits to ensure our survival could include the ability to allow our species to adapt rapidly to the rate of environmental change caused by human activity, for which Darwinian evolution may be too slow [ 39 ]. Or, for instance, to support long-distance space travel by engineering resistance to radiation and osteoporosis, along with other conditions which would be highly advantageous in space [ 40 ].
When considering the ethical and societal merits of these propositions, it is useful to consider how proto-forms of enhancement has been approached by past human societies. In particular, it can be argued that humans have already employed—as part of our domestication/‘selective breeding’ of other animals—techniques of indirect manipulation of genomes on a relatively large scale over many millennia, albeit not on humans. The large-scale selective breeding of plants and animals over prehistoric and historic periods could be claimed to have already shaped some of our natural environment. Selective breeding has been used to obtain specific characteristics considered useful at a given time in plants and animals. Therefore, their evolutionary processes have been altered with the aim to produce lineages with advantageous traits, which contributed to the evolution of different domesticated species. However, differently from genetic engineering, domestication possesses inherent limitations in its ability to produce major transformations in the created lineages, in contrast with the many open possibilities provided by genetic engineering.
When considering the impact of genetic engineering on human evolution, one of questions to be considered concerns the effects, if any, that genetic technology could have on the genetic pool of the human population and any implication on its resilience to unforeseen circumstances. This underlines a relevant question associated with the difference between ‘health’ and biological fitness. For example, a certain group of animals can be more ‘healthy’—as domesticated dogs—but be less biologically ‘fit’ according to Darwin’s definition. Specifically, if such group of animals are less genetically diverse than their ancestors, they could be less ‘adaptable’ to environmental changes. Assuming that, the human germline modification is undertaken at a global scale, this could be expected to have an effect, on the distribution of genetically heritable traits on the human population over time. Considering that gene and trait distributions have been changing under the processes of evolution for billions of years, the impact on evolution will need to be assessed by analysing which genetic alterations have been eventually associated with specific changes within the recent evolutionary history of humans. On this front, a key study has analysed the implications of genetic engineering on the evolutionary biology of human populations, including the possibility of reducing human genetic diversity, for instance creating a ‘biological monoculture’ [ 41 ]. The study argued that genetic engineering will have an insignificant impact on human diversity, while it would likely safeguard the capacity of human populations to deal with disease and new environmental challenges and therefore, ensure the health and longevity of our species [ 41 ]. If the findings of this study were considered consistent with other knowledge and encompassing, the impact of human genetic enhancements on the human genetic pool and associated impacts could be considered secondary aspects. However, data available from studies on domestication strongly suggests that domestication of both animals and plans might lead to not only decreased genetic diversity per se, but even affect patterns of variation in gene expression throughout the genome and generally decreased gene expression diversity across species [ 42–44 ]. Given that, according to recent studies within the field of biological anthropology recent human evolution has been in fact a process of ‘self-domestication’ [ 45 ], one could argue that studies on domestication could contribute to understanding the impacts of genetic engineering.
Beyond such considerations, it is useful to reflect on the fact that human genetic enhancement could occur on different geographical scales, regardless of the specific environment and geological periods in which humans are living and much more rapidly than in the case of evolution, in which changes are very slow. If this was to occur routinely and on a large scale, the implications of the resulting radical and abrupt changes may be difficult to predict and its impacts difficult to manage. This is currently highlighted by results of epigenetics studies, and also of the microbiome and of the effects of pollutants in the environment and their cumulative effect on the development of human and non-human organisms alike. Increasingly new evidence indicates a greater interdependence between humans and their environments (including other microorganisms), indicating that modifying the environment can have direct and unpredictable consequences on humans as well. This highlight the need of a ‘systems level’ approach. An approach in which the ‘bounded body’ of the individual human as a basic unit of biological or social action would need to be questioned in favour of a more encompassing and holistic unit. In fact, within biology, there is a new field, Systems Biology, which stresses the need to understand the role that pleiotropy, and thus networks at multiple levels—e.g. genetic, cellular, among individuals and among different taxa—play within biological systems and their evolution [ 46 ]. Currently, much still needs to be understood about gene function, its role in human biological systems and the interaction between genes and external factors such as environment, diet and so on. In the future if we do choose to genetically enhance human traits to levels unlikely to be achieved by human evolution, it would be crucial to consider if and how our understanding of human evolution enable us to better understand the implications of genetic interventions.
New forms of human enhancement are increasingly coming to play due to technological development. If phenotypic and somatic interventions for human enhancement pose already significant ethical and societal challenges, germline heritable genetic intervention, require much broader and complex considerations at the level of the individual, society and human species as a whole. Germline interventions associated with modern technologies are capable of much more rapid, large-scale impacts and seem capable of radically altering the balance of humans with the environment. We know now that beside the role genes play on biological evolution and development, genetic interventions can induce multiple effects (pleiotropy) and complex epigenetics interactions among genotype, phenotype and ecology of a certain environment. As a result of the rapidity and scale with which such impact could be realized, it is essential for ethical and societal debates, as well as underlying scientific studies, to consider the unit of impact not only to the human body but also to human populations and their natural environment (systems biology). An important practicable distinction between ‘therapy’ and ‘enhancement’ may need to be drawn and effectively implemented in future regulations, although a distinct line between the two may be difficult to draw.
In the future if we do choose to genetically enhance human traits to levels unlikely to be achieved by human evolution, it would be crucial to consider if and how our understanding of humans and other organisms, including domesticated ones, enable us to better understand the implications of genetic interventions. In particular, effective regulation of genetic engineering may need to be based on a deep knowledge of the exact links between phenotype and genotype, as well the interaction of the human species with the environment and vice versa .
For a broader and consistent debate, it will be essential for technological, philosophical, ethical and policy discussions on human enhancement to consider the empirical evidence provided by evolutionary biology, developmental biology and other disciplines.
This work was supported by Fundação para a Ciência e a Tecnologia (FCT) of Portugal [CFCUL/FIL/00678/2019 to M.A.].
Conflict of interest : None declared.
Pham P , Roux S , Matonti F et al. Post-implantation impedance spectroscopy of subretinal micro-electrode arrays, OCT imaging and numerical simulation: towards a more precise neuroprosthesis monitoring tool . J Neural Eng 2013 ; 10 : 046002 .
Google Scholar
Maghami MH , Sodagar AM , Lashay A et al. Visual prostheses: the enabling technology to give sight to the blind . J Ophthal Vis Res 2014 ; 9 : 494 – 505 .
Weitz AC , Nanduri D , Behrend MR et al. Improving the spatial resolution of epiretinal implants by increasing stimulus pulse duration . Sci Transl Med 2015 ; 7 : 318ra203.
Bouton CE , Shaikhouni A , Annetta NV et al. Restoring cortical control of functional movement in a human with quadriplegia . Nature 2016 ; 533 : 247 – 50 .
Geddes L. First paralysed person to be ‘reanimated’ offers neuroscience insights. Technique moves man’s arm by decoding his thoughts and electrically stimulating his own muscles . Nat News 2016 ; 533 .
Squires JE. Artificial blood . Science 2002 ; 295 : 1002 – 5 .
Lowe KC. Blood substitutes: from chemistry to clinic . J Mater Chem 2006 ; 16 : 4189 – 96 .
Moradi S , Jahanian-Najafabadi A , Roudkenar MH. Artificial blood substitutes: first steps on the long route to clinical utility . Clin Med Insights Blood Disord 2016 ; 9 : 33 – 41 .
Powell R , Kahane G , Savulescu J. Evolution, genetic engineering, and human enhancement . Philos Technol 2012 ; 25 : 439 – 58 .
Parens E (ed.). Enhancing Human Traits: Ethical and Social Implications . Washington, DC : Georgetown University Press , 1998 .
Google Preview
Giubilini A , Sanyal S. Challenging human enhancement. In: Clarke S , Savulescu J , Coady T et al. (eds). The Ethics of Human Enhancement: Understanding the Debate . Oxford : Oxford University Press , 2016 .
Elliott C. Better Than Well: American Medicine Meets the American Dream . New York, NY : WWW Norton & Company, Inc ., 2003 .
Kramer P. Listening to Prozac . London : Fourth Estate , 1994 .
Moravec H. Mind Children: The Future of Robot and Human Intelligence . Cambridge : Harvard University Press , 1990 .
Bostrom N. Human genetic enhancements: a transhumanist perspective . J Value Inq 2003 ; 37 : 493 – 506 .
Kurzweil R. The Singularity is Near: When Humans Transcend Biology . New York, NY : Viking , 2005 .
Harris J. Enhancing Evolution: The Ethical Case for Making Better People . Princeton, NJ : Princeton University Press , 2010 .
Fukuyama F. Our Posthuman Future: Consequences of the Biotechnology Revolution . New York, NY : Picador , 2002 .
Sandel M. The Case Against Perfection: Ethics in the Age of Genetic Engineering . Cambridge : The Belknap Press of Harvard University Press , 2007 .
Savulescu J , Persson I. The perils of cognitive enhancement and the urgent imperative to enhance the moral character of humanity . J Appl Philos 2008 ; 25 : 162 – 77 .
Buchanan A. Beyond Humanity . Oxford : Oxford University Press , 2011 .
Persson I , Savulescu J. Moral enhancement, freedom, and the god machine . Monist 2012 ; 95 : 399 – 421 .
Leon K. Ageless bodies, happy souls: biotechnology and the pursuit of perfection . New Atlantis 2003 ; 1 : 9 – 28 .
Agar N. Humanity’s End: Why We Should Reject Radical Enhancement . Cambridge : MIT Press , 2010 .
Gaj T , Gersbach CA , Barbas CF III ,. ZFN, TALEN, and CRISPR/Cas based methods for genome engineering . Trends Biotechnol 2013 ; 3 : 397 – 405 .
Baltimore D , Berg P , Botchan M et al. Biotechnology. A prudent path forward for genomic engineering and germline gene modification . Science 2015 ; 348 : 36 – 8 .
Otieno MO. CRISPR/Cas9 human genome editing: challenges, ethical concerns and implications . J Clin Res Bioeth 2015 ; 6 : 253 .
Ishii T. Germline genome-editing research and its socio-ethical implications . Trends Mol Med 2015 ; 21 : 473 – 81 .
Bionews.org.uk. First Genome-edited Babies: A Very Different Perception of Ethics , 2018 . https://www.bionews.org.uk/page_140060 (27 August 2019, date last accessed).
Cyranoski D. CRISPR-baby scientist fails to satisfy his critics . Nat News 2018 ; 564 : 13 – 4 .
Galis F , Metz JA. Evolutionary novelties: the making and breaking of pleiotropic constraints . Integr Comp Biol 2007 ; 47 : 409 – 19 .
Falcon A , Cuevas MT , Rodriguez-Frandsen A et al. CCR5 deficiency predisposes to fatal outcome in influenza virus infection . J Gen Virol 2015 ; 96 : 2074 – 8 .
Gade-Andavolu R , Comings DE , MacMurray J et al. Association of CCR5 Δ32 deletion with early death in multiple sclerosis . Genet Med 2004 ; 6 : 126 – 31 .
Zhou M , Greenhill S , Huang S et al. CCR5 is a suppressor for cortical plasticity and hippocampal learning and memory . eLife 2016 ; 5 : e20985 .
Tibayrenc M , Ayala FJ (eds). On Human Nature: Biology, Psychology, Ethics, Politics, and Religion . London : Academic Press , 2017 .
Baldi P. The Shattered Self: The End of Natural Evolution . Cambridge : MIT Press , 2001 .
Darwin C. On the Origin of Species by Means of Natural Selection, or, the Preservation of Favoured Races in the Struggle for Life . London : J. Murray , 1859 .
Gould SJ. The Structure of Evolutionary Theory . Belknap, NY : Harvard University Press , 2002 .
Rees M. Our Final Century: Will the Humans Race Survive the Twenty-first Century? Eastbourne : Gardners Books , 2003 .
Nuffield Council on Bioethics. Genome Editing: An Ethical Review . London : Nuffield Council on Bioethics , 2016 .
Powell R. The evolutionary biological implications of human genetic engineering . J Med Philos 2012 ; 37 : 204 – 26 .
Liu W , Chen L , Zhang S et al. Decrease of gene expression diversity during domestication of animals and plants . BMC Evol Biol 2019 ; 19 : 1 – 11 .
Fages A , Hanghøj K , Khan N et al. Tracking five millennia of horse management with extensive ancient genome time series . Cell 2019 ; 177 : 1419 – 35 .
Zhang J , Wang X , Yao J et al. Effect of domestication on the genetic diversity and structure of Saccharina japonica populations in China . Sci Rep 2017 ; 7 : 42158 .
Theofanopoulou C , Gastaldon S , O’Rourke T et al. Self-domestication in Homo sapiens : insights from comparative genomics . PLoS One 2018 ; 13 : e0196700 .
Capra F , Luisi PL. The Systems View of Life . Cambridge : Cambridge University Press , 2014
- genetic engineering
Month: | Total Views: |
---|---|
September 2019 | 51 |
October 2019 | 571 |
November 2019 | 512 |
December 2019 | 474 |
January 2020 | 492 |
February 2020 | 712 |
March 2020 | 680 |
April 2020 | 615 |
May 2020 | 609 |
June 2020 | 599 |
July 2020 | 605 |
August 2020 | 632 |
September 2020 | 842 |
October 2020 | 1,322 |
November 2020 | 1,583 |
December 2020 | 1,688 |
January 2021 | 1,536 |
February 2021 | 2,019 |
March 2021 | 3,077 |
April 2021 | 2,893 |
May 2021 | 2,223 |
June 2021 | 1,434 |
July 2021 | 906 |
August 2021 | 911 |
September 2021 | 1,393 |
October 2021 | 2,158 |
November 2021 | 2,377 |
December 2021 | 1,736 |
January 2022 | 1,315 |
February 2022 | 1,783 |
March 2022 | 2,522 |
April 2022 | 1,946 |
May 2022 | 1,552 |
June 2022 | 789 |
July 2022 | 675 |
August 2022 | 486 |
September 2022 | 919 |
October 2022 | 1,462 |
November 2022 | 1,427 |
December 2022 | 1,216 |
January 2023 | 1,372 |
February 2023 | 1,740 |
March 2023 | 2,320 |
April 2023 | 2,195 |
May 2023 | 1,646 |
June 2023 | 1,066 |
July 2023 | 940 |
August 2023 | 1,070 |
September 2023 | 1,283 |
October 2023 | 1,953 |
November 2023 | 1,886 |
December 2023 | 1,684 |
January 2024 | 1,871 |
February 2024 | 2,312 |
March 2024 | 2,729 |
April 2024 | 2,752 |
May 2024 | 2,034 |
June 2024 | 1,117 |
July 2024 | 854 |
August 2024 | 697 |
Email alerts
Citing articles via, affiliations.
- Online ISSN 2050-6201
- Copyright © 2024 International Society for Evolution, Medicine, and Public Health
- About Oxford Academic
- Publish journals with us
- University press partners
- What we publish
- New features
- Open access
- Institutional account management
- Rights and permissions
- Get help with access
- Accessibility
- Advertising
- Media enquiries
- Oxford University Press
- Oxford Languages
- University of Oxford
Oxford University Press is a department of the University of Oxford. It furthers the University's objective of excellence in research, scholarship, and education by publishing worldwide
- Copyright © 2024 Oxford University Press
- Cookie settings
- Cookie policy
- Privacy policy
- Legal notice
This Feature Is Available To Subscribers Only
Sign In or Create an Account
This PDF is available to Subscribers Only
For full access to this pdf, sign in to an existing account, or purchase an annual subscription.

Biotech potatoes: A case study of how genetic engineering can improve our food supply
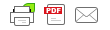
To help demonstrate the power of biotechnology, consider the following analogy: Imagine you have two decks of cards, one red and one blue, and each deck contains all the genes of a potato. The red deck makes a great potato, but lacks resistance to late blight disease. The blue deck has late blight resistance …. but these potatoes are unmarketable.
To get the blue ace of spades (LB resistance) together with the rest of the red deck (good potatoes), you could shuffle the two together and divide the deck in two …. You can keep shuffling this new deck with more red cards, but imagine how many times you would have to shuffle the cards to get a perfect deck ….
Compare this with simply picking out the blue ace of spades and placing it into the red deck. Wouldn’t that be easier? …. This is essentially the difference between using traditional breeding (shuffling) and biotechnology (stacking the deck). …
Together with new breeding technologies …. genetic modification remains a useful tool in the genetic improvement of potatoes. The 100-plus wild species relatives of potato provide a virtually endless source of traits that can be incorporated into elite varieties relatively easily and quickly.
Read the original post
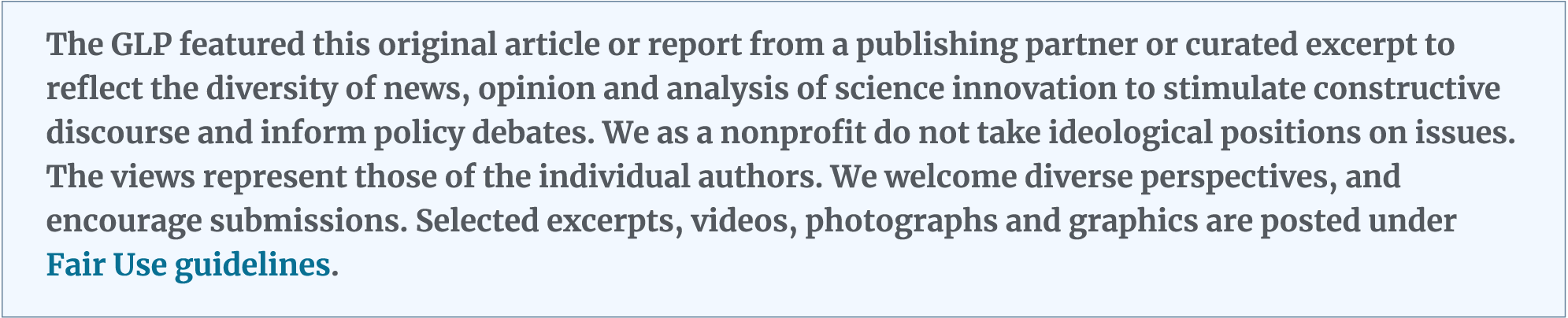
GLP Podcasts & Podcast Videos More...
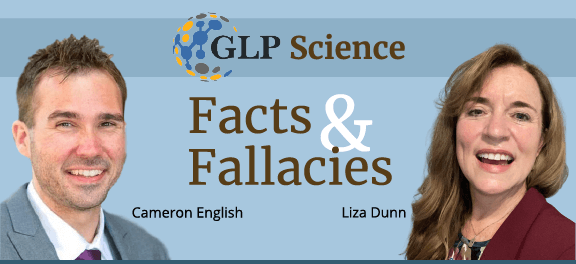
GLP podcast: Hot dogs probably don’t cause dementia; Subsidize plant burgers? Drinking fathers might damage fetal brain development
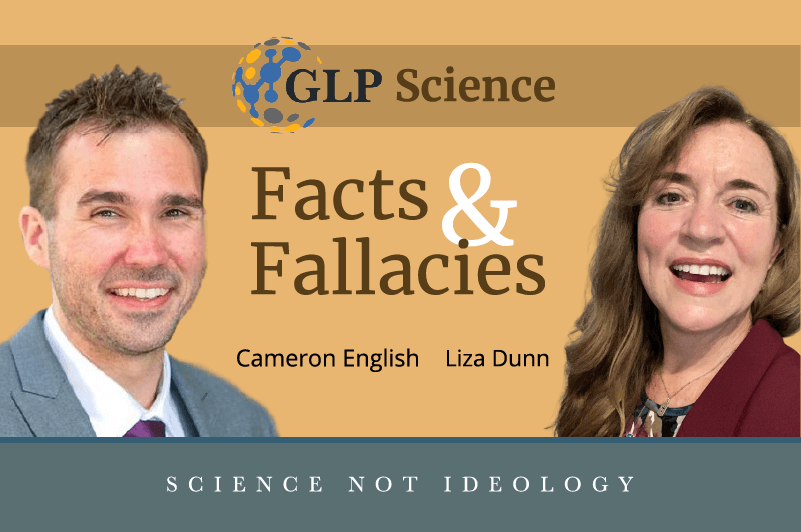
GLP podcast: Treating brain diseases with parasites; FDA rejects ecstasy treatment for PTSD; $6 billion—the cost of baby powder lawsuits
Videos more....

Video: Organic, non-gmo, gluten-free, keto? How food labels mislead us about health benefits
Bees & pollinators more....
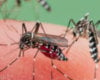
Mosquito massacre: Can we safely tackle malaria with a CRISPR gene drive?
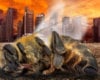
Are we facing an ‘Insect Apocalypse’ caused by ‘intensive, industrial’ farming and agricultural chemicals? The media say yes; Science says ‘no’
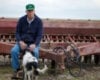
Dissecting claims about Monsanto suing farmers for accidentally planting patented seeds
Infographics more....
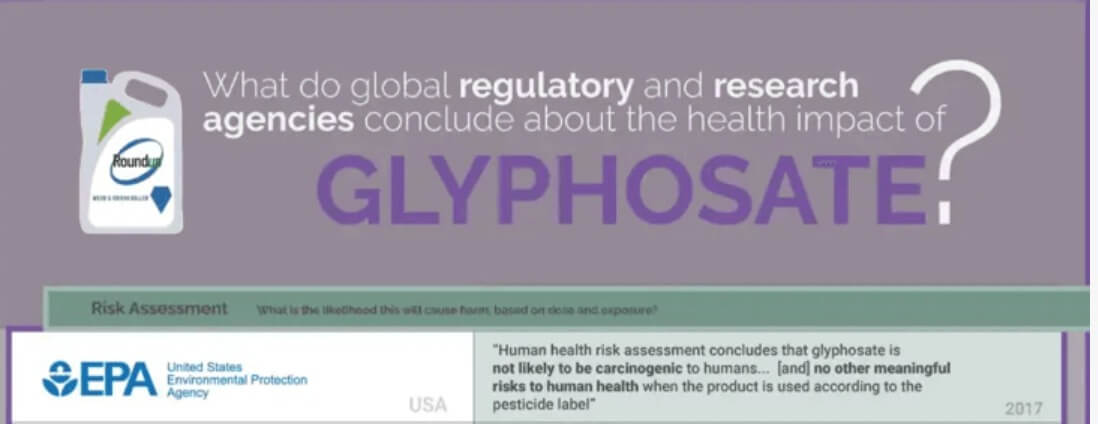
Infographic: Global regulatory and health research agencies on whether glyphosate causes cancer
Gmo faqs more....
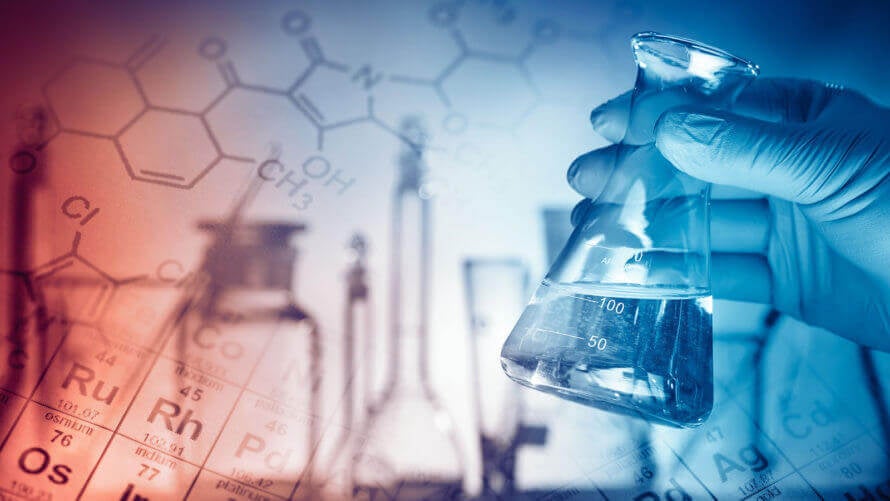
Why is there controversy over GMO foods but not GMO drugs?
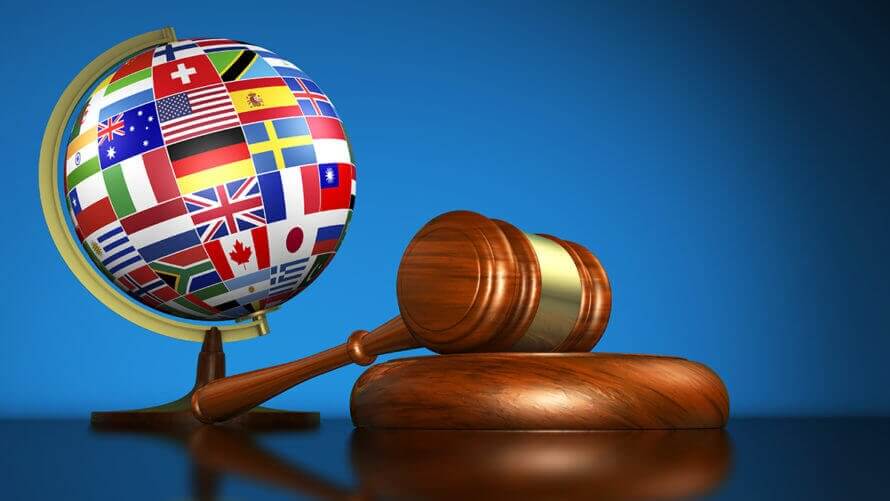
How are GMOs labeled around the world?
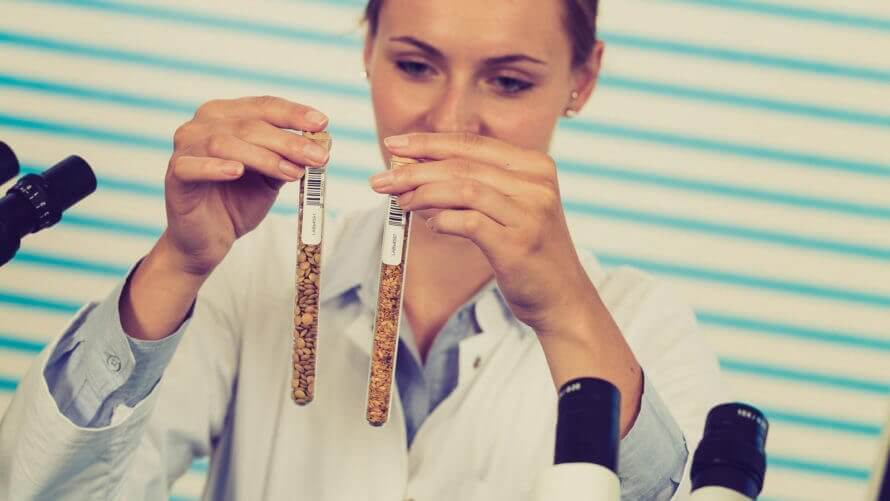
How does genetic engineering differ from conventional breeding?
GLP Profiles |

Alex Jones: Right-wing conspiracy theorist stokes fear of GMOs, pesticides to sell ‘health supplements’

IARC (International Agency for Research on Cancer): Glyphosate cancer determination challenged by world consensus
Most popular.

Newsletter Subscription
- Weekly Newsletter (Wed)
- Daily Digest (Mon, Tue, Thu, Fri)
- Weekly Top Six (Sun)
- Featured Articles Only
- Human Articles Only
- Agriculture Articles Only
- All Types of Content
Get news on human & agricultural genetics and biotechnology delivered to your inbox.
November 1, 2021
Four Success Stories in Gene Therapy
The field is beginning to fulfill its potential. These therapies offer a glimpse of what’s to come
By Jim Daley
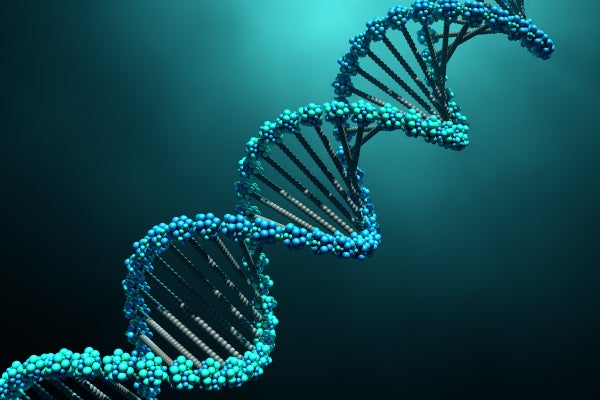
Design Cells Getty Images
After numerous setbacks at the turn of the century, gene therapy is treating diseases ranging from neuromuscular disorders to cancer to blindness. The success is often qualified, however. Some of these therapies have proved effective at alleviating disease but come with a high price tag and other accessibility issues: Even when people know that a protocol exists for their disease and even if they can afford it or have an insurance company that will cover the cost—which can range from $400,000 to $2 million—they may not be able to travel to the few academic centers that offer it. Other therapies alleviate symptoms but don’t eliminate the underlying cause.
“Completely curing patients is obviously going to be a huge success, but it’s not [yet] an achievable aim in a lot of situations,” says Julie Crudele, a neurologist and gene therapy researcher at the University of Washington. Still, even limited advances pave the way for ongoing progress, she adds, pointing to research in her patients who have Duchenne muscular dystrophy: “In most of these clinical trials, we learn important things.”
Thanks to that new knowledge and steadfast investigations, gene therapy researchers can now point to a growing list of successful gene therapies. Here are four of the most promising.
Gene Swaps to Prevent Vision Loss
Some babies are born with severe vision loss caused by retinal diseases that once led inevitably to total blindness. Today some of them can benefit from a gene therapy created by wife-and-husband team Jean Bennett and Albert Maguire, who are now ophthalmologists at the University of Pennsylvania.
When the pair first began researching retinal disease in 1991, none of the genes now known to cause vision loss and blindness had been identified. In 1993 researchers identified one potential target gene, RPE65 . Seven years later Bennett and Maguire tested a therapy targeting that gene in three dogs with severe vision loss—it restored vision for all three.
In humans, the inherited condition that best corresponds with the dogs’ vision loss is Leber congenital amaurosis (LCA). LCA prevents the retina, a layer of light-sensitive cells at the back of the eye, from properly reacting or sending signals to the brain when a photon strikes it. The condition can cause uncontrolled shaking of the eye (nystagmus), prevents pupils from responding to light and typically results in total blindness by age 40. Researchers have linked the disease to mutations or deletions in any one of 27 genes associated with retinal development and function. Until gene therapy, there was no cure.
Mutations in RPE65 are just one cause of inherited retinal dystrophy, but it was a cause that Bennett and Maguire could act on. The researchers used a harmless adeno-associated virus (AAV), which they programmed to find retinal cells and insert a healthy version of the gene, and injected it into a patient’s eye directly underneath the retina. In 2017, after a series of clinical trials, the Food and Drug Administration approved voretigene neparvovecrzyl (marketed as Luxturna) for the treatment of any heritable retinal dystrophy caused by the mutated RPE65 gene, including LCA type 2 and retinitis pigmentosa, another congenital eye disease that affects photoreceptors in the retina. Luxturna was the first FDA-approved in vivo gene therapy, which is delivered to target cells inside the body (previously approved ex vivo therapies deliver the genetic material to target cells in samples collected from the body, which are then reinjected).
Spark Therapeutics, the company that makes Luxturna, estimates that about 6,000 people worldwide and between 1,000 and 2,000 in the U.S. may be eligible for its treatment—few enough that Luxturna was granted “orphan drug” status, a designation that the FDA uses to incentivize development of treatments for rare diseases. That wasn’t enough to bring the cost down. The therapy is priced at about $425,000 per injection, or nearly $1 million for both eyes. Despite the cost, Maguire says, “I have not yet seen anybody in the U.S. who hasn’t gotten access based on inability to pay.”
Those treated show significant improvement: Patients who were once unable to see clearly had their vision restored, often very quickly. Some reported that, after the injections, they could see stars for the first time.
While it is unclear how long the effects will last, follow-up data published in 2017 showed that all 20 patients treated with Luxturna in a phase 3 trial had retained their improved vision three years later. Bennett says five-year follow-up with 29 patients, which is currently undergoing peer review, showed similarly successful results. “These people can now do things they never could have dreamed of doing, and they’re more independent and enjoying life.”
Training the Immune System to Fight Cancer
Gene therapy has made inroads against cancer, too. An approach known as chimeric antigen receptor (CAR) T cell therapy works by programming a patient’s immune cells to recognize and target cells with cancerous mutations. Steven Rosenberg, chief of surgery at the National Cancer Institute, helped to develop the therapy and published the first successful results in a 2010 study for the treatment of lymphoma.
“That patient had massive amounts of disease in his chest and his belly, and he underwent a complete regression,” Rosenberg says—a regression that has now lasted 11 years and counting.
CAR T cell therapy takes advantage of white blood cells, called T cells, that serve as the first line of defense against pathogens. The approach uses a patient’s own T cells, which are removed and genetically altered so they can build receptors specific to cancer cells. Once infused back into the patient, the modified T cells, which now have the ability to recognize and attack cancerous cells, reproduce and remain on alert for future encounters.
In 2016 researchers at the University of Pennsylvania reported results from a CAR T cell treatment, called tisagenlecleucel, for acute lymphoblastic leukemia (ALL), one of the most common childhood cancers. In patients with ALL, mutations in the DNA of bone marrow cells cause them to produce massive quantities of lymphoblasts, or undeveloped white blood cells, which accumulate in the bloodstream. The disease progresses rapidly: adults face a low likelihood of cure, and fewer than half survive more than five years after diagnosis.
When directed against ALL, CAR T cells are ruthlessly efficient—a single modified T cell can kill as many as 100,000 lymphoblasts. In the University of Pennsylvania study, 29 out of 52 ALL patients treated with tisagenlecleucel went into sustained remission. Based on that study’s results, the FDA approved the therapy (produced by Novartis as Kymriah) for treating ALL, and the following year the agency approved it for use against diffuse large B cell lymphoma. The one-time procedure costs upward of $475,000.
CAR T cell therapy is not without risk. It can cause severe side effects, including cytokine release syndrome (CRS), a dangerous inflammatory response that ranges from mild flulike symptoms in less severe cases to multiorgan failure and even death. CRS isn’t specific to CAR T therapy: Researchers first observed it in the 1990s as a side effect of antibody therapies used in organ transplants. Today, with a combination of newer drugs and vigilance, doctors better understand how far they can push treatment without triggering CRS. Rosenberg says that “we know how to deal with side effects as soon as they occur, and serious illness and death from cytokine release syndrome have dropped drastically from the earliest days.”
Through 2020, the remission rate among ALL patients treated with Kymriah was about 85 percent. More than half had no relapses after a year. Novartis plans to track outcomes of all patients who received the therapy for 15 years to better understand how long it remains effective.
Precision Editing for Blood Disorders
One new arrival to the gene therapy scene is being watched particularly closely: in vivo gene editing using a system called CRISPR, which has become one of the most promising gene therapies since Jennifer Doudna and Emmanuelle Charpentier discovered it in 2012—a feat for which they shared the 2020 Nobel Prize in Chemistry. The first results from a small clinical trial aimed at treating sickle cell disease and a closely related disorder, called beta thalassemia, were published this past June.
Sickle cell disease affects millions of people worldwide and causes the production of crescent-shaped red blood cells that are stickier and more rigid than healthy cells, which can lead to anemia and life-threatening health crises. Beta thalassemia, which affects millions more, occurs when a different mutation causes someone’s body to produce less hemoglobin, the iron-rich protein that allows red blood cells to carry oxygen. Bone marrow transplants may offer a cure for those who can find matching donors, but otherwise treatments for both consist primarily of blood transfusions and medications to treat associated complications.
Both sickle cell disease and beta thalassemia are caused by heritable, single-gene mutations, making them good candidates for gene-editing therapy. The method, CRISPR-Cas9, uses DNA sequences from bacteria (clustered regularly interspaced short palindromic repeats, or CRISPR) and a CRISPR-associated enzyme (Cas for short) to edit the patient’s genome. The CRISPR sequences are transcribed onto RNA that locates and identifies DNA sequences to blame for a particular condition. When packaged together with Cas9, transcribed RNA locates the target sequence, and Cas9 snips it out of the DNA, thereby repairing or deactivating the problematic gene.
At a conference this past June, Vertex Pharmaceuticals and CRISPR Therapeutics announced unpublished results from a clinical trial of beta thalassemia and sickle cell patients treated with CTX001, a CRISPR-Cas9-based therapy. In both cases, the therapy does not shut off a target gene but instead delivers a gene that boosts production of healthy fetal hemoglobin—a gene normally turned off shortly after birth. Fifteen people with beta thalassemia were treated with CTX001; after three months or more, all 15 showed rapidly improved hemoglobin levels and no longer required blood transfusions. Seven people with severe sickle cell disease received the same treatment, all of whom showed increased levels of hemoglobin and reported at least three months without severe pain. More than a year later those improvements persisted in five subjects with beta thalassemia and two with sickle cell. The trial is ongoing, and patients are still being enrolled. A Vertex spokesperson says it hopes to enroll 45 patients in all and file for U.S. approval as early as 2022.
Derailing a Potentially Lethal Illness
Spinal muscular atrophy (SMA) is a neurodegenerative disease in which motor neurons—the nerves that control muscle movement and that connect the spinal cord to muscles and organs—degrade, malfunction and die. It is typically diagnosed in infants and toddlers. The underlying cause is a genetic mutation that inhibits production of a protein involved in building and maintaining those motor neurons.
The four types of SMA are ranked by severity and related to how much motor neuron protein a person’s cells can still produce. In the most severe or type I cases, even the most basic functions, such as breathing, sitting and swallowing, prove extremely challenging. Infants diagnosed with type I SMA have historically had a 90 percent mortality rate by one year.
Adrian Krainer, a biochemist at Cold Spring Harbor Laboratory, first grew interested in SMA when he attended a National Institutes of Health workshop in 1999. At the time, Krainer was investigating how RNA mutations cause cancer and genetic diseases when they disrupt a process called splicing, and researchers suspected that a defect in the process might be at the root of SMA. When RNA is transcribed from the DNA template, it needs to be edited or “spliced” into messenger RNA (mRNA) before it can guide protein production. During that editing process, some sequences are cut out (introns), and those that remain (exons) are strung together.
Krainer realized that there were similarities between the defects associated with SMA and one of the mechanisms he had been studying—namely, a mistake that occurs when an important exon is inadvertently lost during RNA splicing. People with SMA were missing one of these crucial gene sequences, called SMN1 .
“If we could figure out why this exon was being skipped and if we could find a solution for that, then presumably this could help all the [SMA] patients,” Krainer says. The solution he and his colleagues hit on, antisense therapy, employs single strands of synthetic nucleotides to deliver genetic instructions directly to cells in the body [see “ The Gene Fix ”]. In SMA’s case, the instructions induce a different motor neuron gene, SMN2 , which normally produces small amounts of the missing motor neuron protein, to produce much more of it and effectively fill in for SMN1 . The first clinical trial to test the approach began in 2010, and by 2016 the FDA approved nusinersen (marketed as Spinraza). Because the therapy does not incorporate itself into the genome, it must be administered every four months to maintain protein production. And it is staggeringly expensive: a single Spinraza treatment costs as much as $750,000 in the first year and $375,000 annually thereafter.
Since 2016, more than 10,000 people have been treated with it worldwide. Although Spinraza can’t restore completely normal motor function (a single motor neuron gene just can’t produce enough protein for that), it can help children with any of the four types of SMA live longer and more active lives. In many cases, Spinraza has improved patients’ motor function, allowing even those with more severe cases to breathe, swallow and sit upright on their own. “The most striking results are in patients who are being treated very shortly after birth, when they have a genetic diagnosis through newborn screening,” Krainer says. “Then, you can actually prevent the onset of the disease—for several years and hopefully forever.”
This article is part of “ Innovations In: Gene Therapy ,” an editorially independent special report that was produced with financial support from Pfizer .

Gene Drives on the Horizon: Advancing Science, Navigating Uncertainty, and Aligning Research with Public Values (2016)
Chapter: 3 case studies to examine questions about gene-drive modified organisms, 3 case studies to examine questions about gene-drive modified organisms.
To examine the questions surrounding gene drive research, this report relies heavily on an extended, iterative exploration of a set of plausible case studies. The case studies are first described in a preliminary fashion in this chapter. Other chapters build on these case studies with deeper discussion of issues pertinent to value-based concerns, scientific techniques to mitigate harms, risk assessment, public engagement, and governance.
The case studies offer practical scenarios on which to base the report’s analysis and recommendations and to provide a sound foundation for the further discussions that will necessarily follow this report as gene drive research advances. Given those two goals, the committee used the following three criteria to select case studies:
- Plausibility: Selection of organisms suitable for the development of a gene drive.
- Likelihood: Selection of areas for gene drive research or applications that are expected to be pursued in the near term.
- Diversity: Selections are intended to reflect a range of plausible target organisms, applications, mechanisms of action, and locations (in terms of where gene drive research is carried out and where organisms could potentially be released).
BASIC CRITERIA FOR THE DEVELOPMENT OF GENE-DRIVE MODIFIED ORGANISMS
It is particularly important to understand what is meant by plausibility . Many organisms and traits are not suitable for gene drive research. The two most basic requirements for a target organism of gene drive work are that it reproduces sexually and that it reproduces rapidly (see Box 3-1 ). For this reason, many insects and rodents are good candidates for gene drive research. Organisms such as viruses, many plants, and most bacteria, which use other means to reproduce, are not good targets for gene drive research (see Box 3-2 for additional considerations for plants). Humans, elephants, and trees are also not good targets for gene drive research because they have long generation times; any modification introduced into such a population could require decades or centuries to become established. However, a gene drive could work in an organism that has alternating sexual and asexual phases of reproduction, as in Plasmodium falciparum , the parasite that causes malaria ( de Koning-Ward et al., 2015 ), even though its population structure may render spread of the gene drive difficult.
In addition, some traits may simply be too complex to alter because they are governed by many genes, their expression is shaped by the external environment, or they are modified by internal or development cues (e.g., epigenetics) that are not yet fully elucidated. For example, flowering time in maize is determined by the cumulative effects of many genes ( Buckler et al., 2009 ).
In some cases, many applications of gene drive research may not be necessary, because efficient non-gene drive approaches are able to generate the desired outcome.
Given these and other technical and regulatory challenges (discussed in detail in the other chapters), predictions about how gene drives might be used need to be treated critically. The committee developed case studies to illustrate the issues highlighted in Table 3-1 .
CASE STUDY 1: USING AEDES AEGYPTI AND AEDES ALBOPICTUS MOSQUITOES TO MANAGE DENGUE
Establish gene drives in Aedes aegypti and Aedes albopictus mosquitos to control the spread of dengue throughout the world.
Dengue, a debilitating viral infection, is one of the leading causes of sickness and death in subtropical and tropical countries around the world. Adults and children who contract dengue often experience a flu-like illness. Severe dengue, also called Dengue Hemorrhagic Fever, causes bleeding, persistent vomiting, breathing difficulties, and other complications that may lead to death. Severe dengue disproportionately affects children.
Dengue is caused by infection with any of five serotypes of dengue virus (which is a flavivirus). The virus is transmitted to humans via the bite of female 1 Aedes aegypti , the primary vector (carrier) in urban areas, or Aedes albopictus , the primary vector in rural areas. In April 2016, the World Health Organization endorsed the use of the first-ever dengue vaccine, Dengvaxia (CYD-TDF) by Sanofi Pasteur, in countries where dengue is endemic. 2 Research is ongoing for other vaccine candidates. Patient recovery for those who are unvaccinated depends heavily on an early diagnosis and careful management of fever symptoms.
___________________
1 Only female mosquitoes bite and drink blood. Female mosquitoes need the protein in blood to make their eggs.
2 See http://www.who.int/immunization/research/development/dengue_vaccines/en [accessed May 2, 2016].
TABLE 3-1 Selected Case Studies on Gene Drive Research and Related Applications
Use the mosquitoes and to manage dengue | Credit: James Gathany | |
Using mosquitoes to combat malaria | Credit: James Gathany | |
Using the mosquitoes to combat avian malaria | Credit: Susanne Bard | |
Controlling populations of non-indigenous mice to protect native biodiversity on islands throughout the world | ||
Controlling non-indigenous knapweeds to protect biodiversity in rangelands and forests in the United States | Credit: Matt Levin | |
Controlling (Palmer amaranth, also known as pigweed) to increase agriculture productivity in the Southern United States | Credit:Michael J. Plagens | |
Developing a vertebrate model for gene drive research using | Credit: Monte Westerfield |
Current Mitigation Efforts
Prevention of dengue relies entirely on vector control, mostly through ultra-low volume spraying of insecticides. Insecticide resistance is challenging the efficacy of such dengue vector control methods using currently available chemicals. Another vector control intervention is the
management of mosquito vector breeding sites, which are typically man-made containers. However, because dengue disease exhibits spatiotemporal heterogeneity epidemic activity (alternating as high and low incidences between years and seasons), and because of the potential serotype interaction and co-circulations, predicting possible epidemics is extremely complex as is effective prevention. These strategies are laborious and typically reactive rather than proactive ( Achee et al., 2015 ). Additional control strategies are listed in Appendix C .
Biological controls also exist, such as the use of cyclopoid copepods ( Marten et al., 1994 ), population reduction via community participation ( Scholte et al., 2006 ; Majambere et al., 2007 ) and the use of larvivorous fish, but the maintenance of the distributed containers is a limiting factor to effective control. Another type of biological control is through the release of Wolbachia -infected mosquitoes. The bacterial symbionts in the genus Wolbachia are widely distributed in insects ( Werren et al., 1995 ; Werren and O’Neil, 1997 ; Bourtzis and Braig, 1999 ; Stouthamer et al., 1999 ). Wolbachia infection reduces the lifespan of the insect hosts ( Sinkins et al., 1997 ; Dobson et al., 2002 ; Ahantarig et al., 2011 ; Bull and Turelli, 2013 ). In addition, Wolbachia infection of Aedes aegypti confers resistance to infection with dengue and chikungunya viruses ( McMeniman et al., 2009 ; Moreira et al., 2009 ; Bian et al., 2010 ). In light of these results, small-scale trials to reduce dengue transmission using Wolbachia started in 2011 in Australia and further expanded to Vietnam, Indonesia, and Brazil. 3 Although on-going large field trials suggest a reduction of dengue incidence, there remain important considerations concerning the unanticipated evolution of the dengue virus (or other viruses infecting the same mosquito vector) that need to be addressed.
In summary, despite many available methods of mosquito control, existing methods are not yet fully effective at reducing dengue transmission.
Plausibility of a Gene Drive Solution
It may be possible to create two types of gene drives in Aedes species: one that prevents the transmission of the dengue virus and another that causes sterility. Research with Wolbachia demonstrates, in principle, the potential for those two approaches. In 2010, researchers showed that Wolbachia can be used to induce resistance in Aedes aegypti to the dengue virus. Wolbachia also can be used to shorten the life-span of Aedes aegypti ( McMeniman et al., 2009 ). Similarly, the U.K.-based company Oxitec has developed a technology to suppress Aedes aegypti populations in which male Aedes aegypti mosquitoes are genetically engineered to be sterile. 4 The first proofs-of-concept experiments demonstrating the creation of a gene drive in the fruit fly, a model organism for invertebrate research, and in other mosquito species (discussed below) also provide evidence that a gene drive could be developed in Aedes aegypti ( Gantz and Bier, 2015 ; Gantz et al., 2015 ; Hammond et al., 2016 ). These applications would require initial release of a number of the gene-drive modified mosquitoes within an urban setting where dengue is endemic or where dengue outbreaks are known.
CASE STUDY 2: USING ANOPHELES GAMBIAE MOSQUITOES TO COMBAT HUMAN MALARIA
Create gene drives in Anopheles gambiae mosquitoes to reduce the spread of human malaria in sub-Saharan Africa.
3 For details, see http://www.eliminatedengue.com/progress .
4 See http://www.oxitec.com/health/our-solution .
Malaria is a serious and sometimes fatal parasitic infection that occurs in nearly 100 countries worldwide. Adults and children who contract malaria often experience high fever and anemia. If the infection is severe, coma and death can occur. Malaria disproportionately affects people, particularly children, in low and middle income countries in sub-Saharan Africa, South Asia, and South America.
Human malaria is caused by any of the five protozoan parasites of the Plasmodium genus. The mosquito Anopheles gambiae is the primary vector (carrier) of Plasmodium in sub-Saharan Africa.
Current methods for malaria control focus on two themes, drug therapy and vector control. The ability to treat infection requires detection of the parasite and access of infected persons to healthcare, which can be extremely challenging in many, if not most, malaria-endemic settings. Malaria vaccines are under development and have shown promise, but will take many more years before they can be fully recommended for wide application. Prevention of transmission targeting the Anopheline mosquito vector is based on interventions recommended by the World Health Organization. These include measures to eliminate breeding sites, spraying insecticides with residual properties onto the walls of houses, and using insecticide-treated bed nets in areas where malaria is endemic. Additional control strategies are listed in Appendix C . However, all of these measures require organized campaigns and sustained resource availability. In addition, efforts to control malaria are in jeopardy due to the spread of insecticide resistance in Anopheles gambiae populations ( Edi et al., 2012 ; Namountougou et al., 2012 ; Cisse et al., 2015 ).
A gene drive that alters the female mosquito’s ability to become infected with the malaria parasite, or one that prevents parasite development within the mosquito, could block malarial transmission without affecting mosquito populations. In November 2015, researchers demonstrated that CRISPR/Cas9 can be used to create a gene drive that could spread anti- Plasmodium genes in populations of a malaria-carrying Anopheline mosquito, Anopheles stephensi ( Gantz et al., 2015 ). However, the system transmits the drive construct at Mendelian frequencies in some crosses, suggesting that this valuable proof-of-principle needs further modification and research before field release ( Gantz et al., 2015 ). Alternatively, a gene drive that alters the fitness of the female mosquito could result in reducing vector populations over time. In December 2015, researchers demonstrated that CRISPR/Cas9 can be used to create a gene drive that causes sterility in female Anopheles gambiae mosquitoes ( Hammond et al., 2016 ). Although one of the research team’s constructs is predicted to spread through a population, it has not yet been shown to spread to high frequency in a population containing heterogeneous genetic backgrounds. Nonetheless, the anti- Plasmodium and the female sterility gene drive approaches theoretically have the potential to eliminate malaria in sub-Saharan African villages where malaria is endemic.
CASE STUDY 3: USING CULEX QUINQUEFASCIATUS MOSQUITOES TO COMBAT AVIAN MALARIA IN HAWAII
Create gene drives in southern house mosquitoes, Culex quinquefasciatus , to reduce the spread of avian malaria to threatened and endangered honeycreeper birds in the Hawaiian Islands.
Avian malaria is a disease caused by protozoan parasites that infect birds. Birds become infected when they are “bitten” by female mosquitoes carrying the parasite. Birds without immune resistance to the parasite become anemic, grow progressively weaker, and ultimately die. Avian malaria is common in most continents, but absent from many isolated islands where mosquitoes (and hence Plasmodium ) do not naturally occur ( Atkinskon, 2005 ). 5 Thus, native birds in Hawaii, the Galapagos, and other archipelagoes, which evolved without natural exposure to Plasmodium parasites, are highly susceptible to avian malaria. The southern house mosquito, Culex quinquefasciatus , is the primary mosquito vector of Plasmodium relictum in Hawaii. The displacement and extinction of native birds has greatly impacted ecological systems and biodiversity in Hawaii, and climate change threatens to expand mosquito ranges into higher elevations, thereby presenting greater harm to bird populations at these elevations.
Prevention of avian malaria transmission has historically been through interventions that target mosquito vector populations using insecticide spraying and larval source management. Similar to resistance of parasites to drugs, many mosquito species are resistant to currently available chemicals, making control difficult. In Hawaii, attempts to control the mosquitoes through such methods have not eliminated the threat. See Appendix C for a comprehensive list of mosquito control strategies.
The use of gene drives could be used as a new strategy to target the mosquito vector to control avian malaria. As described in the first two case studies, there is strong potential to develop gene drives that alter the female mosquito’s ability to become infected with the malaria parasite, or that prevent mosquitoes from reproducing. The first proofs-of-concepts in which gene drives were created in the fruit fly and in other mosquito species provide evidence that a gene drive could also be developed in Culex quinquefasciatus ( Gantz and Bier, 2015 ; Gantz et al., 2015 ; Hammond et al., 2016 ).
CASE STUDY 4: CONTROLLING POPULATIONS OF NON-INDIGENOUS MUS MUSCULUS MICE TO PROTECT BIODIVERSITY ON ISLANDS
Reduce or eliminate populations of the non-indigenous mouse, Mus musculus , to protect native biodiversity on islands around the world.
Invasive species are a leading cause of extinction of native wildlife and plants on islands. Nearly half of all species included on the International Union for the Conservation of Nature’s list of species that are threatened with extinction live on islands. In addition, roughly 70%, 90%, and 95% of all extinctions of mammals, reptiles, and birds occur on islands, respectively ( Campbell et al., 2015 ; Godwin, 2015 ). The activities of the house mouse, Mus musculus , and other
5 See https://pubs.usgs.gov/fs/2005/3151/report.pdf .
introduced rodents reduce the ability of native species to reproduce, alter or destroy habitats so that they no longer support the needs of native species, and in other ways negatively affect island ecosystem dynamics. Approximately 80% of the world’s islands now have invasive rodents ( Campbell et al., 2015 ; Godwin, 2015 ).
Efforts to eradicate rodents from islands include the use of traps, poisons, and biological controls, such as the introduction of predators or diseases. Application of rodenticides can be cost-prohibitive due to expenses associated with regulation compliance, dispersal method, size of the treated area, and cost of the toxicant itself ( Meerburg et al., 2008 ; Williams, 2013 ). Mechanical traps are often considered more humane than rodenticides because they do not involve the use of chemicals that could adversely affect human, animal, and overall ecosystem health ( Lorvelec and Pascal, 2005 ; Witmer et al., 2011 ). However, placing traps and collecting the caught animals is labor intensive, traps do not discriminate between target and non-target organisms ( Lorvelec and Pascal, 2005 ), and traps are insufficient to fully eradicate a rodent population without the use of other methods. Other research aims to use genetic engineering approaches to control rodent populations including RNA interference and developing transgenes that cause female progeny to develop as males or prevent all progeny from developing ( Gemmell et al., 2013 ; He et al., 2015 ). It remains to be seen if such genetic engineering approaches will be effective, scalable and affordable ( Jacob et al., 2008 ; Campbell et al., 2015 ). Additional discussion of these methodologies and a more comprehensive list of other approaches used to control rodent populations are presented in Appendix D .
Scientists are studying a sex-determining gene drive that causes house mice to produce more male offspring than females ( Cocquet et al., 2012 ). If this occurs over multiple generations, it should lead to a reduction in population size over time. The molecular mechanism takes advantage of an endogenous region of high meiotic drive (meaning it is more likely to be inherited) in the mouse genome found on chromosome 17 (an autosome) called the t-complex. In this scenario, male mice are genetically engineered to possess the Sry gene, which promotes male characteristics ( Goodfellow and Lovell-Badge, 1993 ), on chromosome 17 instead of its usual location on the Y chromosome. An XY Sry male is fertile, and upon mating to a wild-type XX female, both the XY and XX offspring (both male and females) possess Sry and physically develop into male mice, with XX male mice being sterile and the XY mice still able to reproduce and transmit Sry. Over time, the population of mice would tend to become all male, leading to a decrease in reproduction and eventual population decline and suppression due to the loss of female mice ( Campbell et al., 2015 ). Male mice are promiscuous, and so have nearly an unlimited amount of reproductive potential, as long as fertile female mice are present. Female mice must go through a gestation period after mating, limiting their ability to contribute their genetic information to future generations. Hence, female mice are the limiting factor in the change of population densities over time. A description of the technique, and elements that helped in the development of a case study in this report can be found on a website dedicated to island conservation created by students from North Carolina State University. 6
Other potential gene drive mechanisms based upon Medea or underdominance strategies could also be used to achieve the same purpose and would involve inducing targeted translocations into the mouse genome.
6 See https://research.ncsu.edu/islandmice/what-can-genetic-engineering-offer/how-is-this-strain-created .
CASE STUDY 5: CONTROLLING NON-INDIGENOUS CENTAUREA MACULOSA KNAPWEEDS TO PROTECT BIODIVERSITY IN RANGELANDS AND FORESTS
Create gene drives in the non-indigenous knapweed species, Centaurea maculosa , to protect biodiversity of native plant species in rangelands and forests in the United States.
The spotted knapweed ( Centaurea maculosa ) is native to Eastern Europe but was introduced to the United States in the late 1800s. By the year 2000, spotted knapweed could be found in 45 of the 50 states and covered nearly 7 million acres of rangeland and pine forest ( Zouhar, 2001 ). Spotted knapweed first invades disturbed habitats; once established, it spreads to native ecosystems, causing soil erosion in the process.
Several attempts have been made to slow the spread of spotted knapweed by using biological controls; these reduce seed production but have not had large effects on the density of Centaurea maculosa plants ( Sheley et al., 1998 ). In addition to biological controls, management of knapweed populations has focused on physical removal, fire, and chemical treatment for infestations ( Sheley et al., 1998 ; Zouhar, 2001 ).
Spotted knapweed is obligately outcrossing ( Harrod and Taylor, 1995 ), meaning that there is little or no self-fertilization and that gene drives would be able to spread throughout knapweed populations. Another factor that makes it potentially suitable for a gene drive is that the basis for its ability to outcompete native plants is thought to come from the production of a compound called catechin ( Thelen et al., 2005 ), which it exudes from its the roots. Catechin inhibits the germination and growth of native plant species, thereby conferring a competitive advantage to spotted knapweed ( Bais et al., 2003 ).
There are two possible gene drive approaches to help limit the spread of spotted knapweed, which could potentially be employed together. The first option is to engineer a suppression gene drive by targeting sex-specific genes, thereby biasing gender ratios and facilitating a population crash. The second is to modify the population by targeting the catechin biosynthetic pathway, which in theory would negatively affect the knapweed’s ability to compete against endemic plants, although this effect is still debated (Perry et al., 2005). In either case, the rate of spread of either of these gene drives is expected to be slow, because spotted knapweed is a perennial plant that lives for approximately 9 years ( Zouhar, 2001 ). In addition, the success of a suppression drive is likely to depend critically on the fertility advantages of sex-modified plants compared to hermaphrodites and also on features such as pollen availability and spatial structure ( Hodgins et al., 2008 ).
Retraction : In March 2016, the Journal of Ecology and authors Laura G. Perry, Ragan Callaway, and Jorge Vivanco retracted a research publication on the ability of knapweed to outcompete native plants through the production of catechin. Given the retraction, a gene drive that inhibits catechin production in knapweed, as discussed in Case Study 5 on page 56 of this report, is no longer considered plausible. The retraction does not affect discussions of Case Study 5 in other chapters, or the report’s conclusions and recommendations. Details about the retraction are accessible online at: http://onlinelibrary.wiley.com/doi/10.1111/1365-2745.12560/full .
CASE STUDY 6: CONTROLLING PALMER AMARANTH TO INCREASE AGRICULTURE PRODUCTIVITY
Create gene drives in Palmer amaranth ( Amaranthus palmeri also called pigweed), to reduce or eliminate the weed on agricultural fields in the Southern United States.
Palmer amaranth infests agricultural fields throughout the American South. It has evolved resistance to the herbicide glyphosate, the world’s most-used herbicide ( Powles, 2008 ), and this resistance has become geographically widespread.
Whether a plant is considered a weed is context-dependent. In one region, a plant is desirable, whereas in another place, the same plant may be a weed. A plant is typically viewed as a “weed” when it has little recognized value in the locale where it is growing and when it grows rapidly and competes with a crop or pastureland for space, light, water, and nutrients. Weed management is a continual and major challenge. In addition to competition for resources and interfering with the management of desirable plants, poisonous weeds can negatively impact human health, crops and livestock ( Bridges et al., 1994 ). Management strategies fall into four major categories: physical and mechanical methods, cultural methods, chemical methods, and biological methods. Examples of mechanical practices include manual removal of weeds, which is labor intensive, or tilling, which can increase soil erosion. Examples of cultural practices include crop rotations using plants that choke out weeds (often there are limited choices available) and using drip irrigation to limit water to planting rows, which only works well in dry regions that extensively irrigate. Examples of biological methods include animal grazing and the use of natural enemies (microbes, insects, and other animals such as nematodes, fish, and birds); these strategies are primarily used in low-intensity management of rangelands, forests, preserved natural areas, and waterways.
In much of production agriculture, the primary approach to control weeds is to use herbicides. Glyphosate, the most commonly used herbicide, is a systemic herbicide that, when applied, moves throughout the plant thus destroying more tissues as compared to contact herbicides. The generation of herbicide-resistant crops has revolutionized weed control. Glyphosate-resistant crops have been rapidly adopted in multiple crops because of economic advantages, strong weed control, and the observation that the glyphosate-resistant crop system confers a lower environmental impact than the approaches it replaced ( Duke and Powles, 2009 ). Unfortunately, after decades of glycophosate use weeds are now adapting, and herbicide resistance is increasing among weed population, reducing the efficacy of glyphosate for weed control ( Powles and Yu, 2010 ). The current strategy to deal with herbicide-resistant weeds is to adopt diverse tactics, combining multiple weed control approaches ( Duke and Powles, 2009 ; Norsworthy et al., 2012 ). The particular combinations of strategies chosen depend on the crop, the region, and the major weeds impacting the particular agricultural system. Details on specific practices can be found on agricultural extension websites at land grant institutions throughout the United States and at equivalent international institutions’ websites.
Palmer amaranth is a likely candidate for gene drive technology, for five reasons. First, it is an annual plant, so it has yearly sexual reproduction and a rapid generation time. Second, Palmer amaranth and some other members of the genus are dioecious (male and female flowers occur on separate plants) ( Steckel, 2007 ), which ensures the outcrossing necessary to spread gene drives.
Third, it does not have an extensive seed bank; studies suggest that most seeds do not persist in the soil, so that there is unlikely to be a seed repository that is immune to the gene drive. Fourth, an Amaranthus species has been transformed genetically ( Pal et al., 2013 ), suggesting that it will be technologically feasible to insert gene drives into Palmer amaranth. Finally, Palmer amaranth is wind-pollinated, implying that the eradication of species will, at the very least, not harm insect pollinators.
In theory, Palmer amaranth could be subjected to two types of gene drive. In the first, a modification drive would target the genes that confer resistance to glyphosate and reestablish the population’s susceptibility to glycophosate herbicides. The potential targets of this gene drive are known, because the glyphosate herbicide acts by interrupting the function of 5-enolpyruvylshikimate-3-phosphate synthase. In Palmer amaranth, this synthase gene has been duplicated extensively, leading to enzyme overexpression and glyphosate resistance ( Gaines et al., 2010 ). Thus, a candidate gene drive would need to target multiple 5-enolpyruvylshikimate-3-phosphate synthase copies that are scattered throughout the genome. If the gene drive succeeded and susceptibility became fixed, glyphosate could then be used again as a tool to limit Palmer amaranth populations.
A second approach would be to build a suppression drive. Although the target and content of such a drive is not yet clear, the fact that there are separate male and female plants implies that there are sex-specific genes that are suitable targets for biasing the sex ratio. Under this approach, the goal would be skew sex ratios until the entire population (or species) collapses.
CASE STUDY 7: DEVELOPING A VERTEBRATE MODEL FOR GENE DRIVE RESEARCH USING ZEBRAFISH 7
Create gene drives in the zebrafish, Danio rerio , to study gene drive mechanisms in a vertebrate animal.
As of April 2016, researchers have not developed a gene-drive modified vertebrate for use in fundamental research in the laboratory but proofs-of-concept for gene drives have been demonstrated in yeast, the fruit fly, and mosquitoes, with the expectation that this technique will be translated to a vertebrate animal at a future date ( DiCarlo et al., 2015 ; Gantz and Bier, 2015 ; Gantz et al., 2015 ; Hammond et al., 2016 ). These current animal models, and the behavior of gene drives in them, will not necessarily recapitulate the behavior of gene drives in vertebrate species. Given the fundamental differences between vertebrates and invertebrates, a vertebrate species for gene drive research will be needed to address a variety of fundamental research topics before using gene drives in other vertebrate animals, particularly those intended for release into the environment; and also potentially to make comparisons with gene drive mechanisms in invertebrates.
7 A mouse could also potentially be a candidate vertebrate model for gene drive research. Research on the naturally occurring t-complex in mice offers insight into how regions of high meiotic drive function and affect characteristics associated with vertebrate development and behavior (see Case Study 4 ). However, these studies may not be broadly applicable to other vertebrates. Also, the gestation period, and thus the generation time, is longer in mice than in zebrafish, which could make it more difficult for research to keep pace with rapid advances in invertebrates. However, existing approaches for gene editing through transient introduction of CRISPR/Cas9 (or other mechanisms) have been successful; thus, the committee considers development of a gene-drive modified mouse for laboratory research plausible, a close second to the case study on zebrafish presented in this report.
Containment of zebrafish is straightforward due to the requirement for appropriate aquatic facilities, while other potential vertebrate models for gene drives, such as the mouse, could more easily escape from, and survive outside, the laboratory. In addition, it may be possible to develop
a self-limiting gene drive in zebrafish by making the drive active only in the presence of tetracycline, which could be required to activate the promoter needed to express the gene drive construct ( Hammond et al., 2016 ).
A gene-drive modified zebrafish could be developed specifically for laboratory studies with no intention for environmental release. The zebrafish provides an outstanding model to address basic research questions about gene drives in a vertebrate species for many reasons ( Shah and Moens, 2016 ). The zebrafish genome has been fully sequenced, and zebrafish have well-characterized traits associated with reproduction and other behaviors ( Howe et al., 2013 ). Zebrafish are also low cost and easy to maintain, have a short generation time, and produce large numbers of offspring ( Lawrence et al., 2012 ; Harris et al., 2014 ). They are also preferred from a regulatory standpoint (e.g., from the standpoint of Institutional Animal Care and Use Committee) with regards to using animal models for research. Moreover, gene editing has already been used successfully in this organism ( Ma and Liu, 2015 ; D’Agostino et al., 2016 ; Lin et al., 2016 ; Prykhozhij et al., 2016 ).
A gene-drive modified zebrafish could be created by inserting a gene drive construct into the fish consisting of Cas9, a gRNA targeting a non-essential locus (e.g., a gene expressed in the eye) and a green fluorescent protein marker to identify the gene-drive modified organism. The latter characteristic would give rise to a visible phenotype upon insertion of the donor template on the construct.
Achee, N.L., F. Gould, T.A. Perkins, R.C. Reiner, Jr., A.C. Morrison, S.A. Ritchie, D.J. Gubler, R. Teyssou, and T.W. Scott. 2015. A critical assessment of vector control for dengue prevention. PLoS Negl. Trop. Dis. 9(5):e0003655.
Ahantarig, A., N. Chauvatcharin, T. Ruang-areerate, V. Baimai, and P. Kittayapong. 2011. Infection incidence and relative density of the bacteriophage WO-B in Aedes albopictus mosquitoes from fields in Thailand. Curr. Microbiol. 62(3):816-820.
Atkinson, CT. 2005. Ecology and Diagnosis of Introduced Avian Malaria in Hawaiian Forest Birds. USGS FS 2005-3151. Pacific Island Ecosystems Research Center, U.S. Geological Survey, 2005 .
Bais, H.P., R. Vepachedu, S. Gilroy, R.M. Callaway, and J.M Vivanco. 2003. Allelopathy and exotic plant invasion: From molecules and genes to species interactions. Science 301(5638):1377-1380.
Bian, G.W., Y. Xu, P. Lu, Y. Xie, and Z.Y. Xi. 2010. The endosymbiotic bacterium Wol-bachia induces resistance to dengue virus in Aedes aegypti . Plos Pathog. 6(4):e1000833.
Bourtzis, K., and H.R. Braig. 1999. The many faces of Wolbachia. Pp. 199-219 in Rickettsiae and Rickettsia Diseases at the Turn of the Third Millennium, D. Raoult, and P. Brouqui, eds. Amsterdam: Elsevier.
Bridges, D.C., C.K. Kvien, J.E. Hook, and C.R. Stark Jr. 1994. Weeds and herbicides of the Virginia-Carolina peanut market area. Appendix 3.1. In D.C. Bridges, ed, An Analysis of the Use and Benefits of Pesticides in U.S.-Grown Peanut: III Virginia-Carolina Production Region. Tifton, GA: National Environmentally Sound Production Agriculture Laboratory. pp. 1-39.
Buckler, E.S., Holland J.B, Bradbury P.J, Acharya C.B., Brown P.J., Browne C., Ersoz E., Flint-Garcia S., Garcia A., Glaubitz J.C., Goodman, M.M., Harjes C., Guill K., Kroon D.E., Larsson S., Lepak N.K., Li H., Mitchell S.E., Peiffer J.A., Rosas M.O., Rocheford T.R., Romay M.C., Romero S., Salvo S., Sanchez Villeda H., Sofia da Silva H., Sun Q., Tian F., Upadyayula N., Ware D., Yates H., Yu J., Zhang Z., Kresovich S., and M.D. McMullen. 2009. The genetic architecture of maize flowering time. Science 325:714-718.
Bull, J.J., and M. Turelli. 2013. Wolbachia versus dengue: Evolutionary forecasts. Evol. Med. Public Health (1):197-207.
Campbell, K.J., J. Beek, C.T. Eason, A.S. Glen, J. Godwin, F. Gould, N.D. Holmes, G.R. Howald, F.M. Madden, J.B. Ponder, D.W. Threadgill, S.A. Wegmann, and G.S. Baxter. 2015. The next generation of rodent eradications: Innovative technologies and tools to improve species specificity and increase their feasibility on islands. Biol. Conserv. 185:47-58.
Cisse, M.B., C. Keita, A. Dicko, D. Dengela, J. Coleman, B. Lucas, J. Mihigo, A. Sadou, A. Belemvire, K. George, C. Fornadel, and R. Beach. 2015. Characterizing the insecticide resistance of Anopheles gambiae in Mali. Malar. J. 14:327.
Cocquet, J., P.J. Ellis, S.K. Mahadevaiah, N.A. Affara, D. Vaiman, and P.S. Burgoyne. 2012. A genetic basis for a postmeiotic X versus Y chromosome intragenomic conflict in the mouse. PLoS Genet. 8(9):e1002900.
D’Agostino, Y., A. Locascio, F. Ristoratore, P. Sordino, A. Spagnuolo, M. Borra, and S. D’Aniello. 2016. A rapid and cheap methodology for CRISPR/Cas9 zebrafish mutant screening. Mol. Biotechnol. 58(1):73-78.
de Koning-Ward, T., P.R. Gilson, and B.S. Crabb. 2015. Advances in molecular genetic systems in malaria. Nat. Rev. Microbiol. 13(6):373-387.
DiCarlo, J.E., A. Chavez, S.L. Dietz, K.M. Esvelt, and G.M. Church. 2015. Safeguarding CRISPR-Cas9 gene drives in yeast. Nat. Biotech. 33(12):1250-1257.
Dobson, S.L., C.W. Fox, and F.M. Jiggins. 2002. The effect of Wolbachia -induced cytoplasmic incompatibility on host population size in natural and manipulated systems. Proc. Biol. Sci. 269(1490):437-445.
Duke, S.O., and S.B. Powles. 2009. Glyphosate resistant crops and weeds: Now and in the future. AgBio-Forum 12(3-4):346-357.
Edi, C.V., B.G. Koudou, C.M. Jones, D. Weetman, and H. Ranson. 2012. Multiple-insecticide resistance in Anopheles gambiae mosquitoes, Southern Côte d’Ivoire. Emerg. Infect. Dis. 18(9):1508-1511.
Gaines, T.A., W. Zhang, D. Wang, B. Bukun, S.T. Chisholm, D.L. Shaner, S.J. Nissen, W.L Patzoldt, P.J. Tranel, A.S Culpepper, T.L. Grey, T.M. Webster, W.K. Vencill, R.D Sammons, J. Jiang, C. Preston, J.E. Leach, and P. Westra. 2010. Gene amplification confers glyphosate resistance in Amaranthus palmeri . Proc. Natl. Acad. Sci. U.S.A. 107(3):1029-1034.
Gantz, V.M., and E. Bier. 2015. Genome editing. The mutagenic chain reaction: A method for converting heterozygous to homozygous mutations. Science 348(6233):442-444.
Gantz, V.M., N. Jasinskiene, O. Tatarenkova, A. Fazekas, V.M. Macias, E. Bier, and A.A. James. 2015. Highly efficient Cas9-mediated gene drive for population modification of the malaria vector mosquito Anopheles stephensi . Proc. Natl. Acad. Sci. U.S.A. 112:E6736-E6743.
Gemmell, N.J., Jalilzadeh, A., Didham, R.K., Soboleva, T., Tompkins, D.M., 2013. TheTrojan female technique: a novel, effective and humane approach for pest population control. Proc. Roy Soc. B: Biol. Sci. 280, 20132549.
Godwin, J. 2015. Gene Drives in Rodents for Invasive Species Control [Webinar]. Available at: http://nas-sites.org/gene-drives/2015/10/02/webinar-gene-drive-research-in-different-organisms/ [accessed April, 2016].
Goodfellow, P. N., Lovell-Badge, R. 1993. SRY and sex determination in mammals. Annu. Rev. Genet. 27: 71-92.
Hammond, A., R. Galizi, K. Kyrou, A. Simoni, C. Siniscalchi, D. Katsanos, M. Gribble, D. Baker, E. Marois, S. Russell, A. Burt, N. Windbichler, A. Crisanti, and T. Nolan. 2016. A CRISPR-Cas9 gene drive system targeting female reproduction in the malaria mosquito vector Anopheles gambiae . Nat. Biotechnol. 34(1):78-83.
Harris, M.P., K. Henke, M.B. Hawkins, and P.E. Witten. 2014. Fish is fish: The use of experimental model species to reveal causes of skeletal diversity in evolution and disease. J. Appl. Ichthyol. 30(4):616-629.
Harrod, R.J., and R.J. Taylor. 1995. Reproduction and pollination biology of Centaurea and Acroptilon species, with emphasis on C. diffusa . Northwest Sci. 69(2):97-105.
He, C., Yin, L., Tang, C., Yin, C., 2013. Multifunctional polymeric nanoparticles for oral delivery of TNF-a siRNA to macrophages. Biomaterials 34, 2843-2854.
Hodgins, K.A., S.C.H. Barrett. 2008. Geographic variation in floral morphology and style-morph ratios in a sexually polymorphic daffodil. American Journal of Botany 95: 185-195.
Howe, K., M.D. Clark, C.F. Torroja, J. Torrance, C. Berthelot, M. Muffato, J.E. Collins, S. Humphray, et al. 2013. The zebrafish reference genome sequence and its relationship to the human genome. Nature 496(7446):498-503.
Jacob, J., G.R. Singleton, and L.A. Hinds. 2008. Fertility control of rodent pests. Wildlife Res. 35(6):487-493.
Lawrence, C., I. Adatto, J. Best, A. James, and K. Maloney. 2012. Generation time of zebrafish ( Danio rerio ) and medakas ( Oryzias latipes ) housed in the same aquaculture facility. Lab. Anim. 41(6):158-165.
Lin, C.Y., C.Y. Chiang, and H.J. Tsai. 2016. Zebrafish and medaka: New model organisms for modern biomedical research. J. Biomed. Sci. 23(1):19.
Lorvelec, O., and M. Pascal. 2005. French attempts to eradicate nonindigenous mammals and their consequences for native biota. Biol. Invasions 7(1):135-140.
Ma, D., and F. Liu. 2015. Genome editing and Its applications in model organisms. Genomics Proteomics Bioinformatics 13(6):336-344.
Majambere, S., S.W. Lindsay, C. Green, B. Kandeh, and U. Fillinger. 2007. Microbial larvicides for malaria control in The Gambia. Malar. J. 6:76.
Marten, G.G., E.S.Bordes, M. Nguyen. 1994. Use of cyclopoid copepods for mosquito control. Hydrobiologia 292(293):491-496.
McMeniman, C.J., R.V. Lane, B.N. Cass, A.W. Fong, M. Sidhu, Y.F. Wang, and S.L. O’Neill. 2009. Stable introduction of a life-shortening Wolbachia infection into the mosquito Aedes aegypti . Science 323(5910):141-144.
Meerburg, B.G., F.W.A. Brom, and A. Kijlstra. 2008. The ethics of rodent control. Pest Manag. Sci. 64(12):1205-1211.
Moreira, L.A., I. Iturbe-Ormaetxe, J.A. Jeffery, G.J. Lu, A.T. Pyke, L.M. Hedges, B.C. Rocha, S. Hall-Mendelin, A. Day, M. Riegler, L.E. Hugo, K.N. Johnson, B.H. Kay, E.A. McGraw, A.F. van den Hurk, P.A. Ryan, and S.L. O’Neill. 2009. A Wolbachia seymbiont in Aedes aegypti limits infection with dengue, Chikungunya, and Plasmodium . Cell 139(7):1268-1278.
Namountougou, M., F. Simard, T. Baldet, A. Diabaté, J.B. Ouédraogo, T. Martin, and R.K. Dabiré. 2012. Multiple insecticide resistance in Anopheles gambiae s.l. populations from Burkina Faso, West Africa. PLoS ONE 7(11):e48412.
Norsworthy, J.K., S.M. Ward, D.R. Shaw, R.S. Llewellyn, R.L. Nichols, T.M. Webster, W. Bradley, G. Frisvold, S.B. Powles, N.R. Burgos, W.W. Witt, and M. Barrett. 2012. Reducing the risks of herbicide resistance: Best management practices and recommendations. Weed Sci. 60 (Suppl. 1):31-62.
Pal, A., S.S. Swain, A.B. Das, A.K. Mukherjee, and P.K. Chand. 2013. Stable germ line transformation of a leafy vegetable crop amaranth (Amaranthus tricolor L.) mediated by Agrobacterium tumefaciens . In Vitro Cell. Dev. Biol. Plant 49(2):114-128.
Powles, S.B., and Q. Yu. 2010. Evolution in action: Plants resistant to herbicides. Annu. Rev. Plant Biol. 61:317-347.
Prykhozhij, S.V., V. Rajan, and J.N. Berman. 2016. A guide to computational tools and design strategies for genome editing experiments in zebrafish using CRISPR/Cas9. Zebrafish 13(1):70-73.
Scholte, E.J., B.G. Knols, and W. Takken. 2006. Infection of the malaria mosquito Anopheles gambiae with the entomopathogenic fungus Metarhizium anisopliae reduces blood feeding and fecundity. J. Invertebr. Pathol. 91(1):43-49.
Shah, A.N., and C.B. Moens. 2016. Approaching perfection: New development in zebrafish genome engineering. Dev. Cell 36(6):595-596.
Sheley, R.L., J.S. Jacobs, and M.F Carpinelli. 1998. Distribution, biology, and management of diffuse knapweed ( Centaurea diffusa ) and spotted knapweed ( Centaurea maculosa ). Weed Technol. 12(2):353-362.
Sinkins, S.P., C.F. Curtis, and S.L. O’Neill. 1997. The potential application of inherited symbiont systems to pest control. Pp. 155-175 in Influential Passengers, S.L. O’ Neill, A. Hoffman, and J. Werren, eds. Oxford: Oxford University Press.
Steckel, L.E. “The dioecious Amaranthus spp.: here to stay.” Weed Technology 21:567-570. 2007.
Stouthamer, R., J.A. Breeuwer, and G.D. Hurst. 1999. Wolbachia pipientis : Microbial manipulator of arthropod reproduction. Annu. Rev. Microbiol. 53:71-102.
Thelen, G.C., J.M. Vivanco, B. Newingham, W. Good, H.P. Bais, P. Landres, A. Caesar, and R.M. Callaway. 2005. Insect herbivory stimulates allelopathic exudation by an invasive plant and the suppression of natives. Ecol. Lett. 8(2):209-217.
Werren, J.H., and S. O’Neil. 1997. The evolution of heritable symbionts. Pp. 1-41 in Influential Passengers: Inherited Microorganisms and Arthropod Reproduction, S. O’Neil, A.A. Hoffmann, and J.H. Werren, eds. New York: Oxford University Press.
Werren, J.H., W. Zhang, and L.R. Guo. 1995. Evolution and phylogeny of Wolbachia : Reproductive parasites of arthropods. Proc. Biol. Sci. 261(1360):55-63.
Williams, T. 2013. Poisons used to kill rodents have safer alternatives. Audubon Magazine, January-February 2013. Available at: http://www.audubonmagazine.org/articles/conservation/poisons-usedkill-rodents-have-safer-alternatives?page=3 [accessed March 17, 2016].
Witmer, G., J. Pierce, and W.C. Pitt. 2011. Eradication of invasive rodents on islands of the United States. Pp. 135-138 in Island Invasives: Eradication and Management. C.R. Vietch, M.N. Clout, and D.R. Towns, eds. Occasional Paper of the IUCN Species Survival Commission No. 42. Available at: https://portals.iucn.org/library/efiles/documents/ssc-op-042.pdf [accessed April 21, 2016].
Zouhar, K.L. 2001. Centaurea maculosa . In Fire Effects Information System. U.S. Department of Agriculture, Forest Service, Rocky Mountain Research Station, Fire Sciences Laboratory [online]. Available at: http://www.fs.fed.us/database/feis [accessed April 21, 2016].
Research on gene drive systems is rapidly advancing. Many proposed applications of gene drive research aim to solve environmental and public health challenges, including the reduction of poverty and the burden of vector-borne diseases, such as malaria and dengue, which disproportionately impact low and middle income countries. However, due to their intrinsic qualities of rapid spread and irreversibility, gene drive systems raise many questions with respect to their safety relative to public and environmental health. Because gene drive systems are designed to alter the environments we share in ways that will be hard to anticipate and impossible to completely roll back, questions about the ethics surrounding use of this research are complex and will require very careful exploration.
Gene Drives on the Horizon outlines the state of knowledge relative to the science, ethics, public engagement, and risk assessment as they pertain to research directions of gene drive systems and governance of the research process. This report offers principles for responsible practices of gene drive research and related applications for use by investigators, their institutions, the research funders, and regulators.
READ FREE ONLINE
Welcome to OpenBook!
You're looking at OpenBook, NAP.edu's online reading room since 1999. Based on feedback from you, our users, we've made some improvements that make it easier than ever to read thousands of publications on our website.
Do you want to take a quick tour of the OpenBook's features?
Show this book's table of contents , where you can jump to any chapter by name.
...or use these buttons to go back to the previous chapter or skip to the next one.
Jump up to the previous page or down to the next one. Also, you can type in a page number and press Enter to go directly to that page in the book.
Switch between the Original Pages , where you can read the report as it appeared in print, and Text Pages for the web version, where you can highlight and search the text.
To search the entire text of this book, type in your search term here and press Enter .
Share a link to this book page on your preferred social network or via email.
View our suggested citation for this chapter.
Ready to take your reading offline? Click here to buy this book in print or download it as a free PDF, if available.
Get Email Updates
Do you enjoy reading reports from the Academies online for free ? Sign up for email notifications and we'll let you know about new publications in your areas of interest when they're released.
We're sorry but you will need to enable Javascript to access all of the features of this site.
Stanford Online
Genetic engineering and biotechnology.
Stanford School of Medicine , Stanford Center for Health Education
The COVID-19 vaccine works by using mRNA genetic sequencing to help your body recognize and defend itself against the virus. This is just one example of how genetic engineering, or the use of technologies to manipulate DNA, is revolutionizing our world today. From genetically modified vegetables designed to stay fresher longer and grow larger to the development of a synthetic human growth hormone, genetic engineering and biotechnology has and holds the potential to change the way we live. In this course, you’ll further your knowledge of genome research and its benefits and risks and explore the latest technologies in the field to form your own opinions on controversial topics.
- Produce different genetically modified organisms (GMOs)
- Apply genetic engineering in biotechnology
- Characterize and classify drug development
- Understand technology licensing and patents in life sciences
Course Outline
Demystify GMOs (genetically modified organisms) through definitions and illustrative examples. You’ll explore methods of genetic modification to better understand its relevance. | Delve into genetic manipulation, such as with bacteria and yeast, and understand the bridge between algae, biology, and genetics. |
Explore the process of developing genetically engineered crops, from gene identification to crop transformation, plant testing, and regulatory approval. | Discover the application of genetic engineering to animals, including xenotransplantation. |
Core Competencies
- Genetically Engineered Organisms
- Genetic Modification Methods
- Genetic Engineering in Plants
- Biotechnology
- Applications of Genetic Engineering
- Pharmaceuticals
- Drug Development
All-Access Plan
One Year Subscription
Enroll in all the courses in the Genetics Advanced Topics program. View and complete course materials, video lectures, assignments and exams, at your own pace. Revisit course materials or jump ahead – all content remains at your fingertips year-round. You also get 365 days of email access to your Stanford teaching assistant.
Small Groups and Team Programs
Special Pricing
Enroll as a group or team and learn together. We can advise you on the best group options to meet your organization’s training and development goals and provide you with the support needed to streamline the process. Participating together, your group will develop a shared knowledge, language, and mindset to tackle the challenges ahead.
What Our Learners Are Saying
The genetic engineering and biotechnology course covers everything from genetically modified vegetables to xenotransplantation. This comprehensive course allowed me to gain practical and theoretical knowledge about the latest sciences in the field.
Shivang P., CEO
Teaching Team

Ximena Ares
Licensing Associate
Stanford University
Ximena Ares is a Licensing Associate at the Stanford Office of Technology Licensing (OTL). Dr. Ares received her Ph.D training in Molecular Biology in Buenos Aires, Argentina and completed her postdoctoral training at the University of California, San Francisco in Human Genetics. Later, she was a scientist at Geron Corporation and a Research Fellow at the Molecular Sciences Institute in Berkeley, California. She joined Stanford OTL in 2004, where she manages a portfolio of about 250 life sciences inventions, makes decisions about their intellectual property protection and negotiates license agreements and other contracts.

Barbara Dunn
Final Foods Inc.
Barbara Dunn is a Senior Biocuration Research Scientist in the Department of Genetics at Stanford University, currently working with the Saccharomyces Genome Database in the laboratory of Dr. J. Michael Cherry. She received her A.B. in Botany at Berkeley, and her Ph.D. in Biological Chemistry at Harvard University, where she studied yeast telomeres in the laboratory of Dr. Jack Szostak. Her recent research has focused on using whole-genome DNA and RNA sequencing, ChIP-Seq, array-CGH, and other “omics” methods to broadly explore evolution in yeast, and particularly the genome structures and genome evolution of industrial yeasts (lager, ale, wine, ethanol, bread).

Arthur Grossman
Professor (by courtesy), Biology
- School of Medicine
Arthur Grossman has been a Staff Scientist at The Carnegie Institution for Science, Department of Plant Biology since 1982, and holds a courtesy appointment as Professor in the Department of Biology at Stanford University. He has performed research across fields ranging from plant biology, microbiology, marine biology, ecology, genomics, engineering and photosynthesis and initiated large scale algal genomics by leading the Chlamydomonas genome project (sequencing of the genome coupled to transcriptomics). During his tenure at Carnegie, he mentored more than fifteen PhD students and approximately 40 post-doctoral fellows (many of whom have become very successful independent scientists at both major universities and in industry). In 2002 he received the Darbaker Prize (Botanical Society of America) for work on microalgae and in 2009 received the Gilbert Morgan Smith Medal (National Academy of Sciences) for the quality of his publications on marine and freshwater algae. In 2015 he was Vice Chair of the Gordon Research Conference on Photosynthesis and in 2017 was Chair of that same conference (Photosynthetic plasticity: From the environment to synthetic systems). He also gave the Arnon endowed lecture on photosynthesis in Berkeley in March of 2017, has given numerous plenary lectures and received a number of fellowships throughout his career, including the Visiting Scientist Fellowship - Department of Life and Environmental Sciences (DiSVA), Università Politecnica delle Marche (UNIVPM) (Italy, 2014), the Lady Davis Fellowship (Israel, 2011) and most recently the Chaire Edmond de Rothschild (to work IBPC in Paris in 2017-2018). He has been Co-Editor in Chief of Journal of Phycology and has served on the editorial boards of many well-respected biological journals including the Annual Review of Genetics, Plant Physiology, Eukaryotic Cell, Journal of Biological Chemistry, Molecular Plant, and Current Genetics. He has also reviewed innumerable papers and grants, served on many scientific panels that has evaluated various programs for granting agencies [NSF, CNRS, Marden program (New Zealand)] and private companies. He has also served on scientific advisory boards for both nonprofit and for profit companies including Phoenix Bioinformatics, Excelixis, Martex, Solazyme/TerraVia, Checkerspot and Phycoil.

Vice President of Program Management
Jose loves talking about science, especially to non-scientists. He has been involved in science outreach and education since he first learned of the simplicity and beauty of the structure of DNA. Naturally, Jose went on to graduate school at Stanford where he received a M.A. in Education and a Ph.D. in Developmental Biology. His doctoral work focused on understanding how epigenetic regulators control the biology of adult stem cells. For example, when some of these regulators misbehave, stem cells are lost to the detriment of the tissue they normally maintain. Why? How? Well, Jose still doesn’t know, but he hopes his work helped add one more piece to the never-ending puzzle of scientific research. After finishing his Ph.D., Jose moved to St. Louis, MO and joined Monsanto as part of a rotational leadership program, where he’s been doing a number of fun things both close and far from his science background. His year-long rotations have spanned biotechnology regulation and policy, global technology strategy, and development of molecular detection technologies. All of these rotations have complemented each other and contributed to his passion for sustainably and safely increasing food productivity and agricultural efficiency. Jose’s favorite activity is backpacking and talking about how light his backpack is over an open fire under the Milky Way-splattered sky of the Sierra Nevada. When he’s not outdoors, which is more frequent than he’d like, Jose enjoys good beer (peanut butter chocolate milk stout is real and delicious), good music (Tool), and thoughtful discussions involving science, education and politics.

Michael Snyder
Stanford W. Ascherman Professor of Genetics
Michael Snyder is the Stanford Ascherman Professor and Chair of Genetics and the Director of the Center of Genomics and Personalized Medicine. Dr. Snyder received his Ph.D. training at the California Institute of Technology and carried out postdoctoral training at Stanford University.
He is a leader in the field of functional genomics and proteomics, and one of the major participants of the ENCODE project. His laboratory study was the first to perform a large-scale functional genomics project in any organism, and has launched many technologies in genomics and proteomics. These including the development of proteome chips, high resolution tiling arrays for the entire human genome, methods for global mapping of transcription factor binding sites (ChIP-chip now replaced by ChIP-seq), paired end sequencing for mapping of structural variation in eukaryotes, de novo genome sequencing of genomes using high throughput technologies and RNA-Seq. These technologies have been used for characterizing genomes, proteomes and regulatory networks. Seminal findings from the Snyder laboratory include the discovery that much more of the human genome is transcribed and contains regulatory information than was previously appreciated, and a high diversity of transcription factor binding occurs both between and within species.
He has also combined different state-of–the-art “omics” technologies to perform the first longitudinal detailed integrative personal omics profile (iPOP) of person and used this to assess disease risk and monitor disease states for personalized medicine. He is a cofounder of several biotechnology companies, including Protometrix (now part of Life Tehcnologies), Affomix (now part of Illumina), Excelix, and Personalis, and he presently serves on the board of a number of companies
You May Also Like

Nutrition Science
SOM-XCHE0001
Stanford School of Medicine, Stanford Center for Health Education

Digital Health Product Development
SOM-XCHE0025

Biology and Applications of the CRISPR/Cas System
- Engineering
- Artificial Intelligence
- Computer Science & Security
- Business & Management
- Energy & Sustainability
- Data Science
- Medicine & Health
- Explore All
- Technical Support
- Master’s Application FAQs
- Master’s Student FAQs
- Master's Tuition & Fees
- Grades & Policies
- HCP History
- Graduate Application FAQs
- Graduate Student FAQs
- Graduate Tuition & Fees
- Community Standards Review Process
- Academic Calendar
- Exams & Homework FAQs
- Enrollment FAQs
- Tuition, Fees, & Payments
- Custom & Executive Programs
- Free Online Courses
- Free Content Library
- School of Engineering
- Graduate School of Education
- Stanford Doerr School of Sustainability
- School of Humanities & Sciences
- Stanford Human Centered Artificial Intelligence (HAI)
- Graduate School of Business
- Stanford Law School
- Learning Collaborations
- Stanford Credentials
- What is a digital credential?
- Grades and Units Information
- Our Community
- Get Course Updates
Thank you for visiting nature.com. You are using a browser version with limited support for CSS. To obtain the best experience, we recommend you use a more up to date browser (or turn off compatibility mode in Internet Explorer). In the meantime, to ensure continued support, we are displaying the site without styles and JavaScript.
- View all journals
- Explore content
- About the journal
- Publish with us
- Review Article
- Open access
- Published: 17 July 2021
Transgenic and genome-edited fruits: background, constraints, benefits, and commercial opportunities
- Maria Lobato-Gómez ORCID: orcid.org/0000-0002-2589-6216 1 ,
- Seanna Hewitt 2 ,
- Teresa Capell 1 ,
- Paul Christou 1 , 3 ,
- Amit Dhingra ORCID: orcid.org/0000-0002-4464-2502 2 &
- Patricia Sarai Girón-Calva ORCID: orcid.org/0000-0003-0166-8478 1
Horticulture Research volume 8 , Article number: 166 ( 2021 ) Cite this article
42k Accesses
48 Citations
23 Altmetric
Metrics details
- Metabolic engineering
- Molecular engineering in plants
Breeding has been used successfully for many years in the fruit industry, giving rise to most of today’s commercial fruit cultivars. More recently, new molecular breeding techniques have addressed some of the constraints of conventional breeding. However, the development and commercial introduction of such novel fruits has been slow and limited with only five genetically engineered fruits currently produced as commercial varieties—virus-resistant papaya and squash were commercialized 25 years ago, whereas insect-resistant eggplant, non-browning apple, and pink-fleshed pineapple have been approved for commercialization within the last 6 years and production continues to increase every year. Advances in molecular genetics, particularly the new wave of genome editing technologies, provide opportunities to develop new fruit cultivars more rapidly. Our review, emphasizes the socioeconomic impact of current commercial fruit cultivars developed by genetic engineering and the potential impact of genome editing on the development of improved cultivars at an accelerated rate.
Similar content being viewed by others
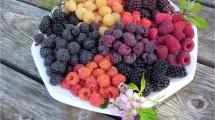
Genetic and genomic resources for Rubus breeding: a roadmap for the future
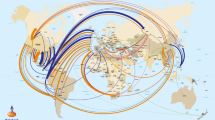
Can gene editing reduce postharvest waste and loss of fruit, vegetables, and ornamentals?
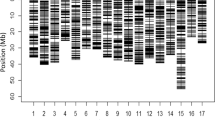
Turbocharging introgression breeding of perennial fruit crops: a case study on apple
Introduction.
The conventional breeding of fruit crops can take more than two decades due to the long juvenile period of woody species 1 . Genetic engineering allows improved varieties to be developed more quickly, and the vegetative propagation of fruit trees allows the engineered cultivars to achieve coverage of larger areas than crops that depend on sexual reproduction 2 . All genetically engineered fruit crops have been produced either by Agrobacterium -mediated transformation or direct DNA transfer. In each case, the efficiency of transformation is highly dependent on the species and even cultivar, requiring the development of bespoke optimized methods consisting of efficient gene delivery, selection, and regeneration from transformed explants 2 . Most fruit tree species are highly heterozygous, and to maintain the characteristics of the original variety the transgenic events should be derived from mature tissue (such as leaves) rather than embryogenic explants 3 .
The first genetically engineered fruit product (Flavr Savr™ tomato) was deregulated in 1992 and introduced into the market in 1994 4 . A gene that triggers pectin solubilization was downregulated in the transgenic fruits, resulting in delayed fruit softening and an extended shelf-life 5 . Several additional fruit crops with traits improved by genetic engineering have received regulatory approval for commercialization in different parts of the world, and are intended for cultivation either as human food or animal feed. These are tomato ( Solanum lycopersicum ) 6 , 7 , 8 , 9 , papaya ( Carica papaya L.) 10 , 11 , pepper ( Capsicum annuum ) 12 , plum ( Prunus domestica ) 13 , eggplant ( Solanum melongena L.) 14 , apple ( Malus domestica Borkh.) 15 , melon ( Cucumis melo L.) 16 , and pineapple ( Ananas comosus L. Merr.) 17 . Most of the transgenic fruits were developed to improve agronomic productivity by conferring pest or disease resistance, or delayed ripening. However, more recent products have addressed quality traits by eliminating fruit browning or adding new visual traits such as flesh color. Some engineered fruit crops have been withdrawn from the market because they were not commercially viable (Flavr Savr™ tomato 4 , 18 ) or were never commercialized (Melon A and B 16 , 19 ).
Advances in genetic engineering, particularly the development of genome editing technologies have provided new tools for the generation of improved fruit varieties. Many proof-of-concept examples involving fruit crops have been reported and the further development and marketing of such varieties could have a major socioeconomic impact. Here we discuss the history and current status of genetically engineered fruit crops and the promise offered by genome editing. In recent years, several countries have amended their current regulations or have developed new guidelines to regulate genome-edited plants and its products 20 . This may make it possible that genome-edited fruits, similarly to all other genome-edited crops, reach the market faster in countries with a genome editing friendly policy 20 , 21 . Here, we first discuss fruit varieties that have already been approved for commercialization, focusing on those that are on the market. We then consider fruit varieties developed more recently using genetic engineering or genome editing, and their potential socioeconomic impact.
Genetically engineered fruits approved for commercialization
Trait description and drivers.
Genetically engineered fruits have been developed with unique agronomic characteristics that are often difficult to achieve by conventional breeding, and are designed to meet the specific needs of growers and/or customers. Fruits that have been developed by genetic engineering are shown in Fig. 1 . Some varieties were approved but not ultimately commercialized, or were launched but subsequently removed from the market, and these are not considered in detail.
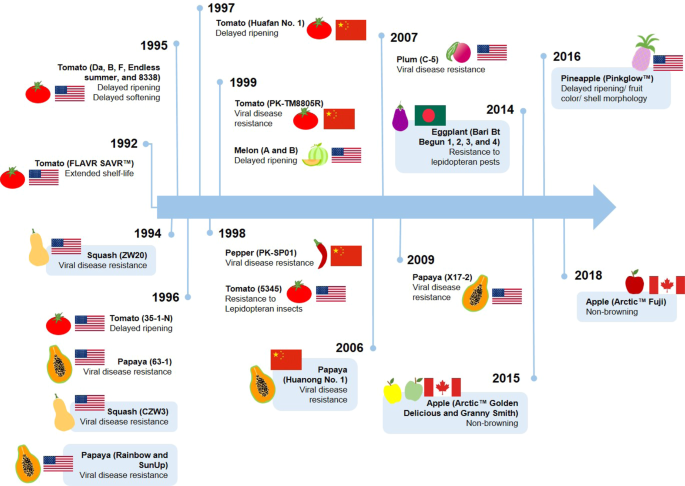
Year indicates the year of first approval. Currently on the market indicated as light blue boxes
Papaya resistant to papaya ringspot virus
In 1992, papaya ringspot virus (PRSV) was detected in Puna, the major papaya-producing district in Hawaii. PRSV resistance was not found in papaya germplasm or in wild Carica species suitable as candidates for interspecific hybridization. Furthermore, insecticides failed to control the aphid vectors responsible for virus transmission 22 , and many orchards were therefore abandoned due to PRSV infestation 10 . The widely cultivated ‘Sunset’ papaya was transformed with a gene derived from a Hawaiian strain to produce the transgenic papaya ‘SunUp’, which is completely resistant to PRSV in Hawaii 10 . ‘SunUp’ papaya was crossed with ‘Kapoho’, a non-engineered cultivar, to obtain the yellow-flesh ‘Rainbow’ papaya, which is also resistant to PRSV 23 .
In China, PRSV has threatened the papaya industry for 50 years 24 . Similarly to the ‘SunUp’ variety, transgenic Huanong No. 1 papaya is resistant to the four predominant PRSV strains found in South China (Hainan, Guangdong, Guangxi, and Yunnan provinces), namely Ys, Vb, Sm and Lc 24 . Additionally, Huanong No. 1 produces bigger fruits with thicker flesh than the original cultivar 24 . In 2012, some Huanong No. 1 papayas grown in Hainan exhibited PRSV-like symptoms, suggesting that resistance is beginning to break. Phylogenetic analysis revealed the presence of a new virus lineage in Hainan and Guangdong papaya plantations, which may pose a threat to Huanong No. 1 papaya cultivation 25 .
Tomato and sweet pepper resistant to cucumber mosaic virus
In 1990, tomato crops in Fujian province (China) were affected by a virulent strain of cucumber mosaic virus (CMV) causing severe necrosis 26 . CMV is a major threat to tomato and sweet pepper and thus the tomato line PK-TM8805R and the sweet pepper line PK-SP01 were developed 24 . Both fruits express a CMV protein gene, conferring resistance to CMV, but data concerning the performance of these cultivars have not been published 26 .
Squash resistant to potyviruses
Like CMV, zucchini yellow mosaic virus (ZYMV) and watermelon mosaic virus 2 (WMV 2) are potyviruses transmitted by aphids. Together, these viruses can reduce the yields of squash by up to 80% 27 . Resistance to these viruses is not found in squash germplasm, and cannot be introduced by interspecific hybridization due to hybrid incompatibility and the concomitant transfer of undesirable traits 28 . In 1995, several transgenic inbred squash lines were developed by transformation with single or multiple viral protein genes from ZYMV, WMV2, and CMV. Transgenic lines ZW-20 and CZW-3 showed complete resistance to ZYMV and WMV2, line CZW-3 showed additional resistance to CMV 28 .
Eggplant resistant to eggplant fruit and shoot borer
In Bangladesh, eggplant is the second most important fruit crop and a major source of income for small, resource-poor farmers 29 . Eggplant fruits are unmarketable when infested with eggplant fruit and shoot borer (EFSB) larvae ( Leucinodes orbonalis ) but effective prevention requires the application of more than 100 sprays of insecticide each season. In addition to the detrimental impact on the environment, this accounts for more than a quarter of production costs, and there are still losses due to the prevalence of EFSB 30 . Resistant cultivars have not been developed by conventional breeding 31 , but a transgenic variety producing Bacillus thuringiensis (Bt) toxins is resistant to EFSB has been commercialized 30 . Infestations of the Bt variety occur at a frequency of 0.04–0.88% compared to 48–57% for the equivalent non-transgenic cultivar. In 2019, the average yield of Bt eggplant in Bangladesh was 19.8 t/ha, compared to 16.6 t/ha for the non-transgenic cultivar 29 .
Non-browning apple
Fruit quality is affected by the activity of polyphenoloxidases (PPOs), which oxidize phenolic compounds and cause gradual browning in fleshy fruits such as apple. PPOs are activated by exposure to oxygen, resulting in browning when fruits are damaged, peeled, or cut. Enzymatic browning can be prevented by storage in an air-free environment, the inactivation of PPOs by irradiation, or through the use of chemical inhibitors and natural antioxidants 32 . The Arctic ® apple concept was developed by silencing of PPOs 33 , 34 . Currently, there are three commercial varieties of Arctic ® apple: Arctic ® Golden Delicious, Arctic ® Granny Smith, and Arctic ® Fuji. Commercial harvest of Arctic ® Golden Delicious and Arctic ® Granny Smith started in 2016, and Arctic ® Fuji will be on the market in 2021 35 .
Pink-fleshed pineapple
Fruits with different skin and flesh colors have been developed by conventional breeding 36 and in proof-of-concept engineering experiments 37 . In 2005, the Pinkglow™ transgenic pineapple was developed, in which the pink flesh accumulates lycopene due to the modification of the carotenoid pathway 17 . The skin of the Pinkglow™ pineapple also has a combination of green, yellow, orange, and red colors, whereas conventional pineapple is green and yellow. In addition to the modulation of carotenoid accumulation, an endogenous ethylene biosynthesis gene was suppressed to control flowering, but this trait has yet to be evaluated 17 .
Development of commercial transgenic fruits (currently on the market)
In 1986, the coat protein of a Hawaiian PRSV isolate was cloned at Cornell University in collaboration with the Asgrow Seed Company. The USDA Section 406 grant program supported the development of transgenic PRSV-resistant papaya with the aim to control PRSV in Hawaii. In 1992, the first PRSV-resistant papayas were developed through a collaboration involving Cornell University, University of Hawaii and the Asgrow company 10 . The University of Hawaii established the protocol for papaya transformation by particle bombardment using zygotic embryos as the starting material 10 , 38 , whereas Huanong No. 1 papaya was generated using an Agrobacterium -mediated procedure established by an independent laboratory 11 . Transgenic papaya resistant to PRSV were developed using a pathogen-derived resistance approach, in which the resistance is mediated via RNA post-transcriptional gene silencing. The underpinning mechanism involves the expression of a partial or full pathogen gene sequence in the host to disrupt the pathogen’s replication 39 . ‘SunUp’ and ‘Rainbow’ papaya contain the coat protein gene from the mild PRSV HA 5-1 isolate 10 . The coat protein is required for virus survival outside the cell and for aphid transmission 40 . The required RNA specificity explains why PRSV-resistant transgenic papaya shows a narrow spectrum of resistance to particular PRSV isolates 41 . Huanong No.1 contains the replicase protein domain (NIb) from the PRSV Ys isolate, the most prevalent strain in China in 1994 24 . The N1b and N1a proteins are needed for virus replication 40 .
Seminis Vegetable Seeds and Monsanto Company developed transgenic virus-resistant squashes in 1995 27 . ZW-20 and CZW-2 virus-resistant squashes were generated using an Agrobacterium -mediated transformation protocol 28 PTGS has been also used to produce ZW-20 and CZW-3 squash. Specifically, these lines contain the coat protein gene from FL isolates of ZYMV and WMV2, and line CZW-3 contains in addition the coat protein gene from CMV strain C 28 .
In 2000, the Maharashtra Hybrid Seeds Company (Mahyco) started to develop Bt eggplant with the collaboration of Monsanto, in India. In 2003, the Agricultural Biotechnology Support Project II (ABSPII) funded a partnership between Mahyco, Cornell University, the US Agency for International Development (USAID), and public-sector partners in India, Bangladesh, and the Philippines to develop and commercialize Bt eggplant. Under the ABSPII agreement, the EE-1 eggplant event, resistant to EFSB, was donated to the public Bangladesh Agricultural Research Institute (BARI) by Mahyco via a public–private partnership 30 . EFSB resistance was incorporated into nine local eggplant lines by BARI. The ASBPII project ended in 2014 and the distribution of Bt eggplant to farmers in Bangladesh was funded by the South Asia Eggplant Improvement Partnership (SAEIP), which comprises BARI, Cornell University, USAID, the University of the Pihilippines Los Banos, and Allience for Science 14 , 30 . Mahyco also set up its own eggplant transformation pipeline. Cotyledons from eggplant seedlings were used as explants for Agrobacterium -mediated transformation with the Bt cry1Ac gene, producing the EE-1 transgenic variety 42 .
Okanagan Specialty Fruits developed Arctic ® Apple events GD743 (Golden Delicious), GS784 (Granny Smith) 33 and GS784 (Fuji) 35 using their patented method to limit quinone biosynthesis 43 . Quinones are produced from diphenols in a reaction catalyzed by PPO, and their condensation with amino acids and proteins generates lignin-like compounds that cause browning. Cell damage is needed for plastidial PPO to act on vacuolar substrates, which is why browning only occurs in cut or otherwise damaged fruit 43 . RNA interference (RNAi) technology was used to target four apple PPO genes by expressing a chimeric sense RNA containing partial coding sequences of PPO2 , GPO3 , APO5 and pSR7 , leading to the generation of dsRNA and the suppression of homologous genes by post-transcriptional silencing 32 .
Del Monte started to develop the Pinkglow™ pineapple by modulating the carotenoid pathway 44 . ‘MD2’, also known as the Del Monte Gold pineapple, is a commercial variety developed by the company and was used as starting material. Ten years later, this transgenic pineapple was patented in the US 17 . Del Monte also patented the transformation method, which involved the cultivation of organogenic pineapple cells with A. tumefaciens . Conventional pineapple on the market has yellow flesh, reflecting the β-carotene content. The Pinkglow™ pineapple expresses the tangerine ( Citrus reticulata ) PSY gene, which is a rate-limiting enzyme in carotenoid biosynthesis during fruit development 17 . In addition, the endogenous lycopene β and ε cyclase genes ( βLYC and εLYC ) were suppressed by RNAi 17 . Ethylene promotes flowering in pineapple, and 1-aminocyclopropane-1 carboxylic acid (ACC) is the immediate ethylene precursor in plants 45 . A meristem-specific ACC synthase (ACS) was suppressed by RNAi in the Pinkglow™ pineapple to inhibit flowering 17 .
Regulatory approval and commercialization of improved fruit crops
The USA has issued the most approvals for transgenic fruit cultivation either for human consumption or as animal feed. Like other genetically engineered crops, three government agencies are responsible for the oversight of transgenic fruit cultivation and import: the US Department of Agriculture (USDA) Animal and Plant Health Inspection Service (APHIS), the US Environmental Protection Agency (EPA), and the US Food and Drug Administration (FDA), which is part of the Department of Health and Human Services. Depending on its characteristics, a genetically engineered fruit may fall under the jurisdiction of one or more of these agencies 46 . APHIS regulates the environmental release of genetically engineered organisms that may pose a risk to plant health, the EPA oversees pesticides, including genetically engineered plants expressing plant incorporated protectants (PIP), and the FDA ensures the safety of all human food and animal feed (also from plant origin).
In 2020, APHIS published a revision of its 1987 biotechnology regulations 47 . The new framework, known as the SECURE rule (Sustainable, Ecological, Consistent, Uniform, Responsible, and Efficient) differs from the previous regulatory framework by focusing on an organism’s properties and not on the production method 47 .
Flavr Savr™ tomato developed by Monsanto Company was the first genetically engineered fruit to gain non-regulated status from APHIS and approval by the FDA 5 , 18 . Flavr Savr™ was also approved for import into Mexico in 1995 by the Federal Commission for the Protection against Sanitary Risk (COFEPRIS), a decentralized organ of the Mexican Secretariat of Health that oversees the safe release and import of genetically engineered plants 48 . COFREPIS also permitted the import of the engineered tomato varieties Da, B, F, and Endless summer. Similarly, in 1995 Health Canada and Agriculture and Agri-food Canada determined that the Flavr Savr™ tomato was safe for human consumption and did not pose risks as a plant pest 49 . In Canada, the Flavr Savr™ tomato was marketed under the brand name MacGregor, allowing consumers to make an informed choice 49 . Flavr Savr™ was removed from the market in 1997 because the fruits were less firm than expected and the costs of production were uncompetitive 18 .
APHIS deregulated additional engineered tomato lines in the 1990s, namely Da, B, F developed by Zeneca and Petoseed Company; 35-1-N developed by Agritope, Inc; and 5345 and 8338 “Endless summer” developed by the Monsanto Company 6 , 7 , 8 , 9 , 50 . These lines were also approved as food and feed. The Da, B, and F lines were intended for processing 4 . Between 1996 and 1999, more than 1.8 million cans derived from hybrids of the F line were sold in the UK 18 , but from 1998 onwards were no longer used as food ingredients 18 . In 2000 Health Canada also approved line 5345, which was resistant to insect pests, but it has not been released onto the market 51 .
In 1999, Agritope was granted FDA approval of the Melon A and B lines for use as food 16 . The company also requested the deregulation of these lines, but withdrew the APHIS petition the same year 19 , and neither line has been commercialized.
The Pinkglow™ pineapple received FDA approval in 2016 and was marketed for the first time in October 2020 by Fresh del Monte 52 , 53 . This cultivar is grown on a single farm in Costa Rica. The C5 plum (HoneySweet) developed by the US Department of Agriculture, which is resistant to plum pox virus (PPV), has also been deregulated by APHIS, approved by the FDA and registered by the EPA 54 . It was patented in the US in 2004, but no trees have been planted thus far and it is therefore not on the market. On request, the Agricultural Research Service (the research branch of the USDA) can freely provide a limited number of heat-treated bud wood samples to be used as a genetic resource for the breeding of PPV-resistant varieties 55 .
Genetically engineered squash has been on the US market for 25 years. CZW3 squash is also approved for import as food by Health Canada 56 . The cultivation of genetically engineered papaya in the US began in 1996, and the current predominant variety is ‘Rainbow’ because it has yellow fruit flesh favored by consumers 4 . Canada and Japan are the major importers of genetically engineered papaya produced in the US, although it is also approved for cultivation in Japan 57 . Two additional papaya lines resistant to PRSV were approved for cultivation by APHIS: 63-1 developed by Cornell University and the University of Hawaii 58 , and X17-2 developed by the University of Florida, respectively 59 . Neither lines have been commercialized 4 .
Arctic ® apples were developed by Okanagan Specialty Fruits Company in Canada, and the Golden Delicious, Granny Smith, and Fuji varieties have received approval for cultivation, human consumption and use as animal feed in both Canada and the US 15 , 60 , 61 , 62 . However, Arctic ® apples are only grown in the US, and it is unclear if Artic varieties are among the 206,259 tons of apples (including dried apples) imported to Canada, most of which are grown in the US 63 , 64 .
In China, the commercialization of all genetically engineered crops is regulated by the Ministry of Agriculture (MOA) 65 , with safety advice provided mainly by the Biosafety Management Division of the Center for Science and Technology Development (CSTD) and the National Biosafety Committee (NBC). The NBC can recommend safety certification based on product testing and field trials, but only the MOA can formally provide regulatory clearance 25 . After registration, genetically engineered crops can be cultivated and commercialized but approval for commercialization is only granted at the province/region level and not nationwide.
Huafan No. 1 tomato developed by Huazhong Agricultural University was the first genetically engineered fruit to be approved for cultivation, human consumption and use as animal feed in China, followed by Da Dong No. 9 (Institute of Microbiology, CAS) and PK-TM8805R (Beijing University) tomatos 26 . Huafan No. 1 and Da Dong No. 9 are no longer cultivated in China, and the status of PK-TM8805R is unclear 26 . Similarly, the genetically engineered sweet pepper PK-SP01 developed by Beijing University was approved for cultivation and for human consumption, but the extent of its cultivation is unclear 26 . PRSV-resistant papaya Huanong No. 1 was approved for cultivation in 2006 and is commercially available in China.
In Bangladesh, the National Committee on Biosafety (NCB) grants regulatory approvals for all genetically engineered crops, assisted by a Biosafety Core Committee (BCC) 66 . The eggplant varieties Bari Bt Begun 1, 2, 3, and 4 were approved for cultivation and food use in Bangladesh, and in 2020 they are the only genetically engineered fruit commercialized in this country 29 , 30 .
Socioeconomic impact of commercialized fruits with improved traits
The socioeconomic impact of genetically engineered fruits is growing with the scale of cultivation, although less than 0.01% of the 185.43 million ha cultivated with genetically engineered crops in 2018 was represented by fruits 67 . Production and adoption rate details are provided in Table 1 . PRSV-resistant papaya is the most widely cultivated genetically engineered fruit, followed by Bt eggplant, virus-resistant squash, Arctic ® apples, and Pinkglow™ pineapple.
Virus-resistant fruits
China grew 9600 ha of PRSV-resistant papaya in 2018. Initial plantings took place in the southern Guangdong Province in 2006, but Hainan Island became the leading location for PRSV-resistant papaya production in 2017 (46%), followed by Guangdong (36%) and Guangxi (18%) provinces 57 . CMV-resistant sweet pepper and tomato have been cultivated in China since 1998 and 1999, respectively, in Beijing municipality and in Fujian and Yunnan provinces, but the scale of cultivation is unclear 26 . Data on the profitability of PRSV-resistant papaya have not been published by the Chinese authorities, so the socioeconomic impact is difficult to judge 68 .
In the US, PRSV-resistant papaya has been commercially grown in Hawaii since 1999 and it has prevented the collapse of the Hawaiian papaya industry due to the prevalence of PRSV in orchards of conventional varieties 23 . In 1992, when PRSV was first detected on Hawaii, the Puna district produced 95% of all Hawaiian papaya grown (~24,000 tons) but yields had fallen to ~12,000 tons in 1998. Two years after the introduction of the resistant variety, yields recovered to ~18,000 tons 23 . Although lower than 1992 levels, the lack of production was not caused by the virus but by the falling demand from Japan, resulting in the papaya cultivation area in Hawaii declining from more than 500 ha in 2015 to only 250 ha in 2018 4 , 67 . The shrinking Japanese market partly reflected the reluctance of retailers to handle genetically engineered products and partly the increased competition from Philippine papaya growers 4 . Nevertheless, the yield of genetically engineered papaya in 2018 was 17% higher than conventional papaya, with a net farm income gain of $2623/ha. Overall, the accumulated farm income benefit between 1999 and 2018 was $38.4 million 67 . Cultivation of PRSV-resistant papaya in Hawaii has also reduced the threat of PRSV in the Puna district, allowing papaya growers to cultivate non-transgenic varieties alongside the genetically engineered crop 23 .
Virus-resistant squash has been commercially grown in the US since 2004, mainly in Florida and Georgia. In 2018, virus-resistant squash was planted on 1000 ha, representing 6% of total squash production in the US 67 . The genetically engineered varieties achieve higher yields than conventional squash, resulting in a net gain to farmers of $10.1 million. Overall, the cumulative farm income benefit between 2004 and 2018 was $310.9 million 67 .
Insect-resistant fruit crops
Bt eggplant was first grown commercially in Bangladesh in 2014, and was cultivated on 2975 ha in 2018 67 . Eggplant is mostly grown by resource-poor farmers, who can obtain seed at no or minimal cost from three organizations: BARI, the Department of Agricultural Extension, and the Bangladesh Agricultural Corporation. Accordingly, the cost of this technology to the farmers is near zero 29 . The Bt eggplant was initially provided to 20 farmers, but by 2018, the variety had been adopted by 20,695 farmers 29 . Bt eggplant achieved 20% higher yields than conventional eggplant in 2018, and the enhanced quality resulted in a 10% increase in price. As a result, farm income has increased by $616–704/ha 29 , 67 .
As well as the direct income gains, Bt eggplant also helps to reduce pesticides. In 2016, farmers in 35 districts cultivating Bt eggplant spent 61% less on pesticides compared to farmers growing conventional varieties 69 . This difference solely represents the cost of pesticides to control EFSB because different chemicals are used to control other pests. However, the prevention of damage caused by EFSB also reduces infestations by secondary pests such as leaf-eating beetles, thrips, whitefly, mites, leaf wing bugs, and leaf roller, by 42–60% 70 .
Fruits with enhanced quality traits
Arctic ® apples were first planted in 2016 (70,000 trees planted over 80 ha). This had grown to 300,000 trees over 101 ha by 2018 and in 2019 the cultivated area exceeded 500 ha 71 . Although the profitability of growing this variety has not been made public, Okanagan Specialty Fruits states that Arctic ® apples are more suitable for mechanical harvesting and suffer less impact from finger bruising, bin rubs and other superficial damage, which results in higher packouts (an industry measure of fruit suitable for market) and therefore less waste, and similar benefits for retailers 72 . Furthermore, the Arctic ® Golden variety does not require warm packing, reducing the cost of production. Del Monte commercialized the Pinkglow™ pineapple in October 2020 so the socioeconomic impact of this variety will not be known until market data are available.
Technological advances in gene functional analysis and genetic modification of fruits
Genetic engineering can be used to investigate the functions of genes and to exploit these functions for the improvement of traits such as biotic and abiotic stress tolerance, flowering time, ripening, fruit flavor, and nutrient content. In this section, we discuss genetic engineering and genome editing technologies that have been used for the enhancement of target traits in fruit crops, which may facilitate commercialization in the future (Table 2 ). Use of CRISPR and associated genome editing technologies for the development or enhancement of fruit crops may open the door to new commercial opportunities, potentially circumventing restrictions on GM crops in many parts of the world 20 . While marketability will vary by country, additional, transgene-free cultivars may be accessible to consumers in the near future 20 , 73 , 74 .
Pathogen and pest resistance
Pathogens and pests are severe constraints affecting the growth and development of fruit trees, the development and ripening of fruits, and the quality of fruit products. In 2017 up to 30% of the fruit and vegetables losses worldwide were pre-harvest, mainly caused by pests and pathogens 75 . In many cases, conventional breeding for resistance is not possible because strong resistance is not present in available germplasm and the introgression process would take too long 2 . One strategy to enhance disease resistance in fruit crops is the modification of receptors that directly interact with or perceive the presence of a specific pathogen. In apple, overexpression of the HcrVf2 gene encoding such a receptor resulted in near-complete resistance to fungal scab ( Venturia inaequalis ) 76 . Recently, CRISPR/Cas9-mediated inactivation of the susceptibility-associated gene DspA/E-interacting protein ( DIPM4 ), also encoding a receptor, significantly reduced bacterial fire blight ( Erwinia amylovora ) symptoms by 50% in apple 77 .
Another strategy for the mitigation of pathogen symptoms is the targeting of response pathways (innate immunity) in the host. For example, the nonexpressor of pathogenesis-related 1 ( NPR1 ) gene encodes a transcriptional regulator of pathogenesis-related (PR) protein genes as part of the salicylic acid-dependent systemically acquired resistance (SAR) pathway. Sweet orange trees ( Citrus sinensus ) overexpressing NPR1 under the control of the phloem-specific SUC2 promoter exhibited enhanced resistance to huánglóngbìng (citrus greening disease), and up to 46% of the engineered plants remained disease-free for 2 years 78 . These findings highlight the importance of promoter selection in overexpression studies and indicate that NPR1 possesses a conserved role among tree fruit species in the response to pathogens.
Other PR-associated proteins have been targeted for modification in banana, chili pepper, and citrus in order to mitigate the effect of bacterial and fungal pathogens. In banana, the induction of a hypersensitive response (HR) by the overexpression of genes encoding an HR-assisting protein and a plant ferredoxin-like protein conferred resistance to banana Xanthomonas wilt, with 50–60% of the transgenic plants displaying no disease symptoms following inoculation 79 . Overexpression of the pepper carboxylesterase gene in chili pepper reduced infections by anthracnose fungus from 70% in wild-type plants to 20% 80 . Similarly, expressing the J1-1 gene encoding an antifungal defensin reduced the frequency of anthracnose lesions by up to 90% 80 , 81 . CRISPR/Cas9 was used to inactivate the grapefruit lateral organ boundary domain family protein 1 and orange WRKY22 genes, which regulate immunity responses, improving resistance to canker caused by Xanthomonas citri subsp. citri ( Xcc ) in Duncan grapefruit ( Citrus ✕ paradisi ) and Wanjincheng orange ( Citrus sinensis (L.) Osbeck) 82 , 83 , 84 , 85 . The CRISPR-induced mutation rate in grapefruit was 23–89%, and Xcc resistance was correlated with the mutation rate, as shown by the corresponding range of canker symptoms 85 . Similar findings were reported for orange plants with mutations in the WRKY22 gene 83 .
In addition to the knockout of host genes to improve pathogen and pest resistance, pathogen-derived transgenes (or other heterologous genes) serve as additional routes for the improvement of fruit traits. In pear, the expression of a bovine lactoferrin gene, which encodes a bactericidal glycoprotein, reduced fire blight symptoms by 78% compared to controls 86 . In sweet orange, expression of the E. amylovora hairpin protein triggered HR in the host plants and reduced susceptibility to citrus canker by up to 79% 87 . The expression of a synthetic insect antimicrobial peptide (cecropin B) in blood orange improved long-term resistance to huánglóngbìng by 85–100% 88 .
An important strategy in the fight against viral diseases is the expression of non-translatable pathogen genes to elicit a PR response or to silence viral components essential for replication, packaging, or systemic spreading. RNAi-mediated silencing of viral components has been achieved in banana, resulting in the complete absence of bunchy top virus disease symptoms in transgenic plants 6 months after challenge 89 . Similarly, transgenic melon and watermelon ( Citrullus lanatus ) lines displayed up to 100% resistance when challenged with several cucurbit viruses 90 , 91 , and grafted transgenic plum lines remained resistant to PPV for more than 9 years 92 . In cucumber, the CRISPR/Cas9 system was used to mutate the eukaryotic translation initiation factor 4E gene, which is associated with CMV susceptibility, resulting in 100% virus-free fruits in the T3 generation 93 . Bt cry genes have been expressed in kiwifruit ( Actinidia chinensis ) and walnut ( Juglans regia ) to protect them against insect pests, resulting in 75–100% insect pest mortality 94 .
Abiotic stress tolerance
Abiotic factors, such as drought, are also among the main factors causing pre-harvest losses of fruit and vegetables 75 . The engineering of abiotic stress tolerance in fruit trees allows them to be grown in environments where temperatures are sub-optimal, water is scarce, or high concentrations of salt and/or heavy metals in the soil are toxic and prevent the uptake of water and nutrients. Overexpression of the Na + /H + cation antiporter gene NHX1 in apple and kiwifruit prolonged survival in saline conditions by allowing the accumulation of higher concentrations of antioxidant flavonoids (60% more than normal) as well as sodium and potassium (2x more than normal) thus delaying the stress response 95 , 96 . In chili pepper, the expression of a tobacco osmotin gene increased yields by 31% accompanied by higher levels of proline, chlorophyll and reactive oxygen species (ROS) scavengers, as well as a higher relative water content 97 . Transgenic citrumelo ( Citrus paradise × Poncirus trifoliata ) plants overexpressing the enzyme Δ1-pyrroline-5-carboxylate synthase, required for proline synthesis, showed a 2.5-fold increase in drought tolerance, as determined by turgor pressure maintenance, stomatal conductance, photosynthetic rate, and transpiration rate 98 .
Fruit crops are often threatened by cold temperatures, which affect plant growth as well as the quality of maturing and ripening fruits. Cold tolerance is therefore an important target in commercial fruit development programs. In apple, overexpression of the transcription factor MYB4, which regulates cold-induced dormancy and stress pathways, allowed the transgenic plants to tolerate cold temperatures for long periods while maintaining normal water content, reflecting the accumulation of glucose, fructose, and sucrose to levels 30–38% higher than normal 99 . Overexpression of the Arabidopsis dehydration response element-binding 1b protein in grapevine reduced cold-induced wilting by 73% 100 . Similarly, the expression of a Poncirus trifoliata basic helix-loop-helix protein in pumello ( Citrus grandis ) enhanced cold tolerance, reduced electrolyte leakage by 13% and increased proline levels by up to 67% compared to wild-type plants 101 .
Flowering time and dormancy release
Flowering time is a very important trait targeted for improvement in fruit crops because of its close association with the timing of fruit development. This trait is under strict genetic regulation and is dependent on environmental conditions, particularly temperature and day length, which limits the geographical regions in which crops can be cultivated 102 . Genetic engineering has been used to express floral activators or repressors, allowing the specification of floral transition and dormancy requirements in major fruit tree species. In transgenic apple, plum, and citrus trees, the overexpression of FT family floral activators needed to trigger bud breaking promoted early flowering (by up to 45 weeks in apple and 12 weeks in orange), and reduced dormancy requirements, eliminating them completely in plum 103 , 104 , 105 . Recently, CRISPR/Cas9 was used to inactivate the self-pruning 5G gene in tomato, which abolished sensitivity to day length and reduced the time to harvest by 2 weeks, translating to a greatly accelerated flowering stage and early fruit yield 102 . In kiwifruit, CRISPR/Cas9-mediated repression of the CEN-like genes also led to rapid and early terminal flowering 106 . These experiments provide insights into the genetic and environmental control of flowering time in different fruits and form the basis for additional engineering strategies to develop early or late-flowering cultivars adapted to specific growing regions.
Fruit ripening and sensory attributes
The modulation of fruit ripening is one of the major strategies by which flavor, aroma, and nutrient profiles can be adjusted, and by which the shelf-life can be extended to improve marketability and reduce waste. In climacteric fruits such as apple, banana, and tomato, the key targets are genes associated with ethylene biosynthesis and degradation. In apple, the silencing of ACS and ACC oxidase ( ACO ) by expressing antisense RNA generated fruit that produced 60% less ethylene, increasing firmness by 20% and allowing cold storage for up to 3 years 107 . Although the synthesis of volatile esters was suppressed, sugar and organic acid accumulation were unaffected. Co-suppression and knockdown of ethylene-biosynthetic genes achieved similar results in pear, kiwifruit, and papaya 108 , 109 , 110 .
Sugar and organic acid content can be modified to enhance fruit flavor. In strawberry, the suppression of ADP-glucose pyrophosphorylase by expressing antisense RNA under the control of a fruit-specific promoter inhibited the conversion of sugar to starch and reduced the starch content of transgenic fruits by up to 47% while increasing the soluble sugar content by up to 37% 111 . Plant pigments such as anthocyanins and carotenoids are also major targets for metabolic engineering in fruits because they provide health benefits and allow the production of fruits with unique colors. The overexpression of MYB family transcription factors in apple, grapevine, and strawberry enhanced the production and storage of anthocyanins, with transgenic fruits accumulating up to 50% more than normal 36 , 112 , 113 . The accumulation of carotenoids has been achieved by the RNAi-mediated silencing of β-carotene hydroxylase in sweet orange, preventing conversion of β-carotene to xanthophylls and thus increasing the β-carotene content in the fruit pulp by 26-fold. Caenorhabditis elegans adults fed with diets supplemented with β-carotene-enriched orange pulp were 20% more resistant to hydrogen peroxide-induced oxidative stress than those fed with control diet 114 . These studies demonstrate how genetic engineering and genome editing can be used to produce fruits with enhanced flavor, texture, and nutrient levels.
Trans-grafting
Grafting is widely used during the propagation of fruit trees to allow the selection of rootstock and scions with different favorable characteristics that may be difficult or laborious to combine in one cultivar (such as high fruit yields paired with resistance to root pests). The rootstock and scion still influence each other by exchanging soluble signals, but the two components maintain their genetic integrity 115 . Trans-grafting refers to grafting of a non-transgenic scion onto a transgenic rootstock. Some desirable characteristics of the rootstock, such as dwarfing or disease resistance, are conferred upon the scion by the vascular transport of RNA, hormones or signaling proteins, but the shoot, leaves, and fruits remain transgene-free 116 , 117 . Although the specific regulations vary by country, trans-grafting can be used to circumvent restrictions on the marketing of GM products in certain jurisdictions 118 . This technology has been used in apple, by grafting non-transgenic scions onto rootstock expressing the Agrobacterium rhizogenes rolB gene, which confers dwarfing characteristics on the scion 119 . In grapevine, non-transgenic scions were grafted onto rootstocks engineered to produce an antimicrobial peptide and a protein that inhibits cell wall degradation. These proteins were transported to the scion through the xylem, resulting in the enhanced mobilization of water and nutrients and a 30–95% reduction in pathogen-induced mortality 120 . Transgenic rootstocks can therefore improve the production of commercially important fruit trees but the fruits and seeds do not carry any exogenous DNA 79 .
Moving beyond transgenesis—genome editing technologies
Genome editing is perhaps the most important recent development in crop breeding, and protocols based on the versatile CRISPR/Cas9 system have been optimized for several fruit species to increase the editing efficiency. In apple, CRISPR/Cas9 produced transgene-free edits 121 . In cucumber, wild strawberry, and watermelon, CRISPR/Cas9 constructs were integrated as part of the T-DNA but segregation was then achieved through back-crossing 122 , 123 , 124 , 125 . A major challenge to the commercial development of edited varieties is the successful transmission of targeted mutations through the germline 126 . This is particularly difficult in woody species, including fruit trees, because they are propagated vegetatively. Back-crossing could take decades (depending on the species) and could result in the unintentional outcrossing of the edited gene. It is also difficult to achieve homozygosity at the edited locus within the desired genetic background because most fruit trees are self-incompatible and thus require obligate outcrossing. Such characteristics hinder the introduction of genome edits that are stable and heritable 127 , 128 , 129 . Several new derivatives of the original CRISPR/Cas9 editing platform have been proposed, including CRISPR/Cas9 ribonucleoprotein (RNP) technology, CRISPR cytidine and adenosine base editors (CBEs/ABEs), CRISPR flippase, and new CRISPR-associated nucleases such as Cas12a/Cpf1, which may help to address these challenges and accelerate the development and commercialization of genome-edited crops 77 , 126 , 129 , 130 , 131 , 132 .
CRISPR RNP technology
Transgene-free genome editing improves the commercialization potential of modified crops (including fruits) because the CRISPR/Cas9 cassette is not inserted into the genome and, in many jurisdictions, the resulting variety is regulated in the same manner as a conventional crop, with certain caveats 21 . CRISPR/Cas9 RNP technology avoids transgene integration by delivering purified RNPs containing the Cas9 protein and gRNA into plant protoplasts and the subsequent regeneration of plants 133 , 134 . This approach has already been used in apple and grapevine to introduce mutations that confer resistance to fire blight and powdery mildew, respectively 129 . In addition to Cas9 RNPs, CRISPR/Cpf1-RNPs have also been employed successfully for gene editing in protoplasts of soybean and tobacco, paving the way for future use in other crops, including fruits and vegetables 134 . Subsequent optimization experiments permitted plant regeneration from protoplasts and improved the transformation protocol for grape protoplasts, reducing the amount of time needed for RNP delivery and genome editing to less than 3 weeks 131 . It is likely that species- and even cultivar-specific protocol optimization will be necessary to achieve satisfactory editing efficiencies because the major hurdle is not the delivery of RNPs across the protoplast membrane, but the subsequent recovery and regeneration of fertile plants.
CRISPR base editing
Whereas conventional CRISPR/Cas9 editing tends to introduce short insertions or deletions at the target locus, cytidine and adenosine base editing facilitates the targeted introduction of single nucleotide replacements by direct C-to-T or A-to-G base conversion, respectively. Base editors have been used to introduce herbicide resistance traits in fruit crops in proof-of-concept experiments. For example, CBE in the watermelon ALS gene resulted in a single amino acid substitution that was sufficient to confer broad-spectrum and heritable resistance to commercial sulfonylurea herbicides 122 .
CRISPR flippase
Flp/ FRT is a yeast site-specific recombinase system in which the recombinase Flp (flippase) catalyzes recombination between two copies of the 34-bp FRT site, resulting in the excision or inversion of the intervening DNA, depending on the relative orientation of the FRT sites. The Flp/ FRT system has been used to remove selectable markers in T1 apple, apricot, citrus, and grapevine plants, leaving a single FRT site behind as a footprint 2 . These studies laid the foundations for more recent work in which the FLP/FRT system was placed under the control of a heat-shock promoter and incorporated into the CRISPR/Cas9 plasmid, allowing the editing of a disease susceptibility gene in apple and subsequent removal of the CRISPR/Cas9 components 77 . This technology has yet to be applied in other fruit crops, but it shows great promise given the efficiency of editing and T-DNA excision.
New CRISPR nucleases
Most CRISPR studies thus far have used the endonuclease Cas9 from Streptococcus pyrogenes (SpCas9). In its native form, SpCas9 requires a trans-activating CRISPR RNA (tracrRNA) and a CRISPR-RNA (crRNA) to induce blunt double-strand breaks in target DNA. These functions were combined into a single gRNA for the development of CRISPR/Cas9 as an engineering tool. But SpCas9 is only one of a large family of CRISPR-associated nucleases with diverse properties, some of which may be advantageous for genome editing in fruit crops by improving efficiency, specificity, or versatility, or by reducing costs 135 . For example, Cas9 from Staphylococcus aureus (SaCas9) differs from SpCas9 in terms of protospacer adjacent motif (PAM) specificity but has a similar editing efficiency. It has been used in several model plant species and also recently in citrus, and provides greater versatility by extending the range of potential genomic targets 126 .
Cas12a/Cpf1 from Prevotella and Francisella spp. recognizes a T-rich PAM and generates compatible cohesive ends with overhangs of 4–5 nt, differing from the blunt ends introduced by Cas9, and increasing the efficiency of DNA integration (knock-in) 136 . Cas12a/Cpf1 is also a smaller protein than Cas9, which improves the efficiency of multiplex editing. CsmI is also smaller than Cas9 136 , and recognizes AT-rich PAM sites thus improving the accuracy of genome editing in AT-rich regions 135 . This approach has been employed to edit the PDS gene in citrus, establishing the feasibility of Cpf1-mediated, DNA-free editing in fruit crops 137 .
Conclusions
Genetic engineering facilitates the development of fruits with useful agronomic or quality traits that are difficult or laborious to achieve by conventional breeding, either due to the lack of suitable germplasm or the long breeding cycles and need for multiple rounds of back-crossing. The same traits can be introduced by genetic engineering in one generation, often directly into elite varieties. Some genetically engineered fruits have been on the market for more than 25 years, and have achieved a remarkable positive socioeconomic impact by reducing pests and diseases and increasing the quality of the end product, both of which help to increase income for farmers. Further benefits to farmers, consumers, and the environment reflect the reduced use of pesticides. The development of new molecular breeding technologies such as trans-grafting and genome editing not only offer the promise of further commercial fruit varieties with resistance to biotic and abiotic stresses, improved flavor and nutrient content, and modified flowering and ripening times, but also help to address some of the regulatory constraints that limit the cultivation of first-generation transgenic crops. In particular, the development of transgene-free genome editing methods based on CRISPR/Cas9 and other nucleases offers a way to introduce precise changes at preselected genomic sites with no genetic footprints and no off-targets. In many jurisdictions, some varieties generated through genome editing are exempt from GMO regulations. These tools and techniques are available for the accelerated development of fruit crops with properties that satisfy the needs of producers, retailers, and consumers, in a sustainable and environmentally friendly manner.
Janick, J. in Plant Breeding Reviews Ch. 8, Vol. 25 (ed Janick, J.) (Springer, 2005).
Song, G., Prieto, H. & Orbovic, V. Agrobacterium-mediated transformation of tree fruit crops: methods, progress, and challenges. Front. Plant Sci. 10 , 226 (2019).
Article PubMed PubMed Central Google Scholar
Limera, C., Sabbadini, S., Sweet, J. B., Mezzetti, B. & Kühn-institut, J. New biotechnological tools for the genetic improvement of major woody fruit species. Front. Microbiol. 8 , 1–16 (2017).
Google Scholar
Baranski, R., Klimek-Chodacka, M. & Lukasiewicz, A. Approved genetically modified (GM) horticultural plants: a 25-year perspective. Folia Hortic. 31 , 3–49 (2019).
Article Google Scholar
Kramer, M. G. & Redenbaugh, K. Commercialization of a tomato with an antisense polygalacturonase gene: The FLAVR SAVR™ tomato story. Euphytica 79 , 293–297 (1994).
APHIS. Interpretative ruling on DNA Plant Technology Corporation petition for determination of non-regulated status of delayed-ripening tomato line 1345-4. Docket No. 94-092-2 (1994).
APHIS. Interpretative ruling on Agritope, Inc. petition for determination of regulatory status of cherry tomatoes with a S - adenosylmethionine hydrolase gene. Docket No. 95-097-1 (1995).
APHIS. Interpretative ruling on Zeneca Plant Science and Petoseed Company Inc. petition for release from regulation for modified lines B, Da- and F derived from T7 varieties of processing tomatoes. Docket No. 95–016–1 (1995).
APHIS. Interpretive ruling on Monsanto petition of nonregulated status for insect resistant tomato line 5345. Docket number: 97-114-1 (1998).
Fitch, M., Manshardt, R., Gonsalves, D., Slightom, J. & Sanford, J. Virus resistant papaya plants derived from tissues bombarded with the coat protein gene of papaya ringspot virus. Nat. Biotechnol. 10 , 1466–1472 (1992).
Article CAS Google Scholar
Chen, G., Ye, C. M., Huang, J. C., Yu, M. & Li, B. J. Cloning of the papaya ringspot virus (PRSV) replicase gene and generation of PRSV-resistant papayas through the introduction of the PRSV replicase gene. Plant Cell Rep. 20 , 272–277 (2001).
Chen, Z. L. et al. Safety assessment for genetically modified sweet pepper and tomato. Toxicology 188 , 297–307 (2003).
Article PubMed CAS Google Scholar
Scorza, R. et al. Transgenic plums ( Prunus domestica L.) express the plum pox virus coat protein gene. Plant Cell Rep. 14 , 18–22 (1994).
Shelton, A. M. et al. Bt Eggplant: a genetically engineered ‘minor’ crop comes of age in Bangladesh and the Philippines. ISB News Rep . (VTechWorks, 2017).
USDA. USDA announces deregulation of non-browning apples. (2015). Available at: https://www.aphis.usda.gov/stakeholders/downloads/2015/SA_arctic_apples.pdf [Accessed June 2020].
Biosafety Clearing-House. Melon A and B. Country’s decision or any other communication (1999). Available at: http://bch.cbd.int/database/record.shtml?documentid=6351 [Accessed Sept 2020]
Firoozbady, E. & Young, T. R. Pineapple plant named “Rose”. US Patent PP25,763 P3 (2015).
Bruening, G. & Lyons, M. J. The case of the FLAVR SAVR tomato. Calif. Agric . 54 , 6–7 (2000).
APHIS. Petition for determination of nonregulated status—Melon. Petition number 98-350-01p (1999).
Menz, J., Modrzejewski, D., Hartung, F., Wilhelm, R. & Sprink, T. Genome edited crops touch the market: a view on the global development and regulatory environment. Front. Plant Sci. 11 , 586027 (2020).
Álvarez, D. et al. Fruit crops in the era of genome editing–closing the regulatory gap. Plant Cell Rep. 40 , 915–930 (2021).
Conover, R. A. Distortion ringspot, a severe virus disease of papaya in Florida. Proc. Fl. State Hortic. Soc. 77 , 440–444 (1964).
Gonsalves, D., Basin, P., Ferreira, S., Sciences, E. P. & Resources, H. Transgenic papaya: a case for managing risks of papaya ringspot virus in Hawaii plant health progress. Plant Manag. Netw. 1 , 1–5 (2003).
Ye, C. & Li, H. 20 years of transgenic research in china for resistance to papaya ringspot virus. Transgenic Plant J. 4 , 58–63 (2010).
Wu, Z., Mo, C., Zhang, S. & Li, H. Characterization of papaya ringspot virus isolates infecting transgenic papaya ‘Huanong No.1’ in South China. Sci. Rep . 8 , 8206 (2018).
ChinaAg. Market reports - The splice must grow: The bright and shady sides of GM agriculture in China. (2016). Available at: https://www.chinaag.org/markets/gm-agriculture-in-china/ [Accessed May 2020].
APHIS. Interpretive ruling on Asgrow Seed Company petition for determination of nonregulated status: Squash containing the coat protein genes from cucumber mosaic virus (CMV), watermelon mosaic virus 2 (WMV 2) and zucchini yellow mosaic virus (ZYMV). Docket No. 96-002-1 (1995).
Tricoli, D. M. et al. Field evaluation of transgenic squash containing single or multiple virus coat protein gene constructs for resistance to cucumber mosaic virus, watermelon mosaic virus 2, and zucchini yellow mosaic virus. Nat. Biotechnol. 13 , 1458–1465 (1995).
Shelton, A. M., Sarwer, S. H., Hossain, M. J., Brookes, G. & Paranjape, V. Impact of Bt Brinjal cultivation in the market value chain in five districts of Bangladesh. Front. Bioeng. Biotechnol. 8 , 1–12 (2020).
Shelton, A. M. et al. Bt eggplant project in Bangladesh: History, present status, and future direction. Front. Bioeng. Biotechnol. 6 , 1–6 (2018).
Alam, S. N. et al. Development of an integrated pest management strategy for eggplant fruit and shoot borer in South Asia. AVRDC 3 , (2003).
Moon, K. M., Kwon, E. Bin, Lee, B. & Kim, C. Y. Recent trends in controlling the enzymatic browning of fruit and vegetable products. Molecules 25 , 2754 (2020).
APHIS. Interpretative rule on Okanagan Speciality Fruits petition for determination of nonregulated status: Arctic™ Apple ( Malus × domestica) . Events GD743 and GS784. Docket No. APHIS-2012-0025 (2012).
Stowe, E. & Dhingra, A. Development of the Arctic ® Apple. Plant Breed. Rev. 44 , 273–296 (2021).
Okanagan Specialty Fruits. Arctic apples: our apples. (2020). Available at: https://arcticapples.com/our-apples/ [Accessed May 2020].
Zhang, J. et al. Decreased protein abundance of lycopene á-cyclase contributesto red flesh in domesticated watermelon. Plant Physiol. 183 , 1171–1183 (2020).
Article PubMed PubMed Central CAS Google Scholar
Espley, R. V. et al. Red colouration in apple fruit is due to the activity of theMYB transcription factor, MdMYB10. Plant J 49 , 414–427 (2007).
Fitch, M. M. M., Manshardt, R. M., Gonsalves, D., Slightom, J. L. & Sanford, J. C. Stable transformation of papaya via microprojectile bombardment. Plant Cell Rep. 9 , 189–194 (1990).
Tennant, P. et al. Papaya ringspot virus resistance of transgenic Rainbow and SunUp is affected by gene dosage, plant development, and coat protein homology. Eur . Eur. J. Plant Pathol. 107 , 645–653 (2001).
Ivanov, K. I., Eskelin, K., Lõhmus, A. & Mäkinen, K. Molecular and cellular mechanisms underlying potyvirus infection. J. Gen. Virol. 95 , 1415–1429 (2014).
Tennant, P. F. et al. Differential protection against papaya ringspot virus isolates in coat protein gene transgenic papaya and classically cross-protected papaya. Phytopathology 84 , 1359–1366 (1994).
Bhagirath, C. & Kadambini, G. The development and regulation of Bt Brinjal in India. ISAAA Briefs 38 , xii + 102 (2009).
Armstrong, J. & Lane, W. D. Genetically modified reduced-browning fruit-producing plant and produced fruit thereof, and method of obtaining such. US Patent 8,563,805 B2 (2013).
Farré, G. et al. Engineering complex metabolic pathways in plants. Annu. Rev. Plant Biol. 65 , 187–223 (2014).
Adams, D. O. & Yang, S. F. Ethylene biosynthesis: Identification of 1-aminocyclopropane-1-carboxylic acid as an intermediate in the conversion of methionine to ethylene. Proc. Natl Acad. Sci. 76 , 170–174 (1979).
USDA. Regulation of biotech plants. (2020). Available at: https://www.usda.gov/topics/biotechnology/how-federal-government-regulates-biotech-plants [Accessed Oct 2020].
APHIS. About the secure rule. (2020). Available at: https://www.aphis.usda.gov/aphis/ourfocus/biotechnology/biotech-rule-revision/secure-rule/secure-about/340_2017_perdue_biotechreg [Accessed Oct 2020].
COFEPRIS. Organismos Géneticamente Modificados. (2017). Available at: https://www.gob.mx/cofepris/acciones-y-programas/organismos-geneticamente-modificados [Accessed Aug 2020].
Health Canada. Safety Assessment of the Flavr Savr™ Tomato. (1997). Available at: https://www.canada.ca/en/health-canada/services/food-nutrition/genetically-modified-foods-other-novel-foods/approved-products/information-safety-assessment-flavr-savr-tomato.html [Accessed Feb, June 2020].
APHIS. Interpretative ruling on Monsanto Company petition for determination of regulatory status of tomatoes with delayed ripening gene. Docket No. 95-042-1 (1994).
ILSI. A review of the environmental safety of the Cry1Ac protein. Environ. Biosaf. Res. 10 , 27–49 (2011).
FDA. New plant variety consultations, Pineapple event EF2-114. BNF No . 149 (2016).
Fresh del Monte. Pinkglow pineapple. (2020). Available at: https://www.pinkglowpineapple.com/ [Accessed Dec 2020].
Scorza, R. et al. ‘HoneySweet’—a transgenic plum pox virus resistant plum—from laboratory and experimental field plots, to regulatory approval. Acta Hortic. 974 , 57–64 (2013).
Scorza, R. et al. Honeysweet (C5), the first genetically engineered plum pox virus-resistant plum ( Prunus domestica L.) cultivar. HortScience 51 , 601–603 (2016).
Health Canada. Novel food information—Virus resistant squash line CZW-3. (1999). Available at: https://www.canada.ca/en/health-canada/services/food-nutrition/genetically-modified-foods-other-novel-foods/approved-products/virus-resistant-squash-line-czw-3.html [Accessed May 2020].
ISAAA. Global status of commercialized biotech/GM Crops in 2017: Biotech crop adoption surges as economic benefits accumulate in 22 years. ISAAA Briefs 53 , 1–143 (2017).
APHIS. Interpretative ruling on Cornell University and the University of Hawaii petition for determination of nonregulated status for ‘Sunset’ papaya lines 55-1 and 63-1. Docket No. 96-024-1 (1996).
APHIS. Interpretative ruling on University of Florida petition for determination of nonregulated status for X17-2 papaya resistant to papaya ringspot virus. Docket No. APHIS-2008-0054 (2009).
Health Canada. Arctic ® Apple Events GD743 and GS784. (2015). Available at: https://www.canada.ca/en/health-canada/services/food-nutrition/genetically-modified-foods-other-novel-foods/approved-products/novel-food-information-arctic-apple-events-gd743-gs784.html [Accessed June 2020].
APHIS. Extended determination of nonregulated status for Okanagan Specialty Fruits non-browning Arctic ® apple (16-004-01p). Docket No. APHIS-2016-0043 (2016).
Health Canada. Novel food Information—Arctic Fuji Apple event NF872. (2018). Available at: https://www.canada.ca/en/health-canada/services/food-nutrition/genetically-modified-foods-other-novel-foods/approved-products/arctic-fuji-apple/information.html [Accessed June 2020].
Statistics Canada. Statistical Overview of the Canadian Fruit Industry 2019. Fruit export volume by commodity – metric tonnes. (2020). Available at: https://www.agr.gc.ca/eng/horticulture/horticulture-sector-reports/statistical-overview-of-the-canadian-fruit-industry-2019/?id=1564485377504 [Accessed Oct 2020].
USDA. List of bioengineered foods, BE Apple crop. (2020). Available at: https://www.ams.usda.gov/sites/default/files/media/BEAppleCropSummary.pdf [Accessed June 2020].
Huang, J. & Wang, Q. Agricultural biotechnology development and policy in China. AgBioForum 5 , 122–135 (2002).
Bangladesh Biosafety. 2017 user’s guide to biosafety regulatory process for genetically engineered plants in Bangladesh. (2017). Available at: https://bangladeshbiosafety.org/wp-content/uploads/2017/11/Users_Guide_to_Biosafety_Regulatory_Process_Bangladesh_2017.pdf [Accessed Aug 2020].
Brookes, G. & Barfoot, P. GM crops: global socio-economic and environmental impacts 1996-2018. PG Econ. Ltd , UK 1–213 (2020).
Brookes, G. & Barfoot, P. GM crop technology use 1996–2018: farm income and production impacts. GM Crop. Food 11 , 242–261 (2020).
Rashid, M., Hasan, M. & Matin, M. Socio-economic performance of Bt eggplant cultivation in Bangladesh. Bangladesh . J. Agric. Res. 43 , 187–203 (2018).
Ahmed, A., Hoddinott, J. F., Abedin, N. & Hossain, N. Z. Economic and health impacts of genetically modified eggplant: Results from a randomized controlled trial of Bt brinjal in Bangladesh. IFPRI Discussion paper 01866 (2019).
Fresh Plaza. Non-browning fruit grower eyes big growth. (2019). Available at: https://www.freshplaza.com/article/9153190/non-browning-fruit-grower-eyes-big-growth/ [Accessed Sept 2020].
Okanagan Specialty Fruits. Supply chain benefits of Arctic® apples. (2020). Available at: https://www.okspecialtyfruits.com/apple-supply-chain/ [Accessed Oct 2020].
Wang, T., Zhang, H. & Zhu, H. CRISPR technology is revolutionizing the improvement of tomato and other fruit crops. Hortic. Res. 6 , 77 (2019).
Erpen-Dalla Corte, L. et al. Development of Improved Fruit, Vegetable, and Ornamental Crops Using the CRISPR/Cas9 Genome Editing Technique. Plants 8 , 601 (2019).
Article PubMed Central CAS Google Scholar
FAO. Food loss and waste database (2019). Available at: http://www.fao.org/platform-food-loss-waste/flw-data/en/ [Accessed Jan 2021].
Szankowski, I. et al. Highly scab-resistant transgenic apple lines achieved by introgression of HcrVf2 controlled by different native promoter lengths. Tree Genet. Genomes 5 , 349–358 (2009).
Pompili, V., Dalla Costa, L., Piazza, S., Pindo, M. & Malnoy, M. Reduced fire blight susceptibility in apple cultivars using a high-efficiency CRISPR/Cas9-FLP/FRT-based gene editing system. Plant Biotechnol. J. 18 , 845–858 (2020).
Dutt, M., Barthe, G., Irey, M. & Grosser, J. Transgenic citrus expressing an Arabidopsis NPR1 gene exhibit enhanced resistance against Huanglongbing (HLB; Citrus greening). PLoS ONE 10 , 1–17 (2015).
Tripathi, L. et al. Field trial of Xanthomonas wilt disease-resistant bananas in East Africa. Nat. Biotechnol. 32 , 868–870 (2014).
Seo, H. H. et al. Overexpression of a defensin enhances resistance to a fruit-specific anthracnose fungus in pepper. PLoS One 9 , 1–11 (2014).
Ko, M. et al. Constitutive expression of a fungus-inducible carboxylesterase improves disease resistance in transgenic pepper plants. Planta 244 , 379–392 (2016).
Peng, A. et al. Engineering canker‐resistant plants through CRISPR/Cas9‐targeted editing of the susceptibility gene Cs LOB 1 promoter in citrus. Plant Biotechnol. J. 15 , 1509–1519 (2017).
Wang, L. et al. CRISPR/Cas9-mediated editing of CsWRKY22 reduces susceptibility to Xanthomonas citri subsp. citri in Wanjincheng orange (Citrus sinensis (L.) Osbeck. Plant Biotechnol. Rep. 13 , 501–510 (2019).
Jia, H., Orbovic, V., Jones, J. B. & Wang, N. Modification of the PthA4 effector binding elements in Type I CsLOB1 promoter using Cas9/sgRNA to produce transgenic Duncan grapefruit alleviating XccΔpthA4: DCsLOB1.3 infection. Plant Biotechnol. J. 14 , 1291–1301 (2016).
Jia, H. et al. Genome editing of the disease susceptibility gene CsLOB1 in citrus confers resistance to citrus canker. Plant Biotechnol. J. 15 , 817–823 (2017).
Malnoy, M., Venisse, J.-S., Brisset, M.-N. & Chevreau, E. Expression of bovine lactoferrin cDNA confers resistance to Erwinia amylovora in transgenic pear. Mol. Breed. 12 , 231–244 (2003).
Barbosa-Mendes, J. M. et al. Genetic transformation of Citrus sinensis cv. Hamlin with hrpN gene from Erwinia amylovora and evaluation of the transgenic lines for resistance to citrus canker. Sci. Hortic. (Amst.). 122 , 109–115 (2009).
Zou, X. et al. Transgenic citrus expressing synthesized cecropin B genes in the phloem exhibits decreased susceptibility to Huanglongbing. Plant Mol. Biol. 93 , 341–353 (2017).
Shekhawat, U. K. S., Ganapathi, T. R. & Hadapad, A. B. Transgenic banana plants expressing small interfering RNAs targeted against viral replication initiation gene display high-level resistance to banana bunchy top virus infection. J. Gen. Virol. 93 , 1804–1813 (2012).
Emran, A. M., Tabei, Y., Kobayashi, K., Yamaoka, N. & Nishiguchi, M. Molecular analysis of transgenic melon plants showing virus resistance conferred by direct repeat of movement gene of Cucumber green mottle mosaic virus. Plant Cell Rep. 31 , 1371–1377 (2012).
Lin, C.-Y. et al. Development of transgenic watermelon resistant to Cucumber mosaic virus and Watermelon mosaic virus by using a single chimeric transgene construct. Transgenic Res. 21 , 983–993 (2012).
Sidorova, T., Mikhailov, R., Pushin, A., Miroshnichenko, D. & Dolgov, S. Agrobacterium -mediated transformation of Russian commercial plum cv. “Startovaya” ( Prunus domestica L.) with virus-derived hairpin RNA construct confers durable resistance to PPV infection in mature plants. Front. Plant Sci. 10 , 1–15 (2019).
Chandrasekaran, J. et al. Development of broad virus resistance in non-transgenic cucumber using CRISPR/Cas9 technology. Mol. Plant Pathol. 17 , 1140–1153 (2016).
Dandekar, A. M. et al. High levels of expression of full-length cryIA(c) gene from Bacillus thuringiensis in transgenic somatic walnut embryos. Plant Sci. 131 , 181–193 (1998).
Li, Y. et al. Overexpression of a Malus vacuolar Na + /H + antiporter gene ( MdNHX1 ) in apple rootstock M.26 and its influence on salt tolerance. Plant Cell. Tissue Organ Cult. 102 , 337–345 (2010).
Tian, N., Wang, J. & Xu, Z. Q. Overexpression of Na + /H + antiporter gene AtNHX1 from Arabidopsis thaliana improves the salt tolerance of kiwifruit ( Actinidia deliciosa ). South Afr. J. Bot. 77 , 160–169 (2011).
Subramanyam, K., Sailaja, K. V., Subramanyam, K., Muralidhara Rao, D. & Lakshmidevi, K. Ectopic expression of an osmotin gene leads to enhanced salt tolerance in transgenic chilli pepper ( Capsicum annum L.). Plant Cell. Tissue Organ Cult. 105 , 181–192 (2011).
de Campos, M. K. F. et al. Drought tolerance and antioxidant enzymatic activity in transgenic ‘Swingle’citrumelo plants over-accumulating proline. Environ. Exp. Bot. 72 , 242–250 (2011).
Malnoy, M., Jin, Q., Borejsza-Wysocka, E. E., He, S. Y. & Aldwinckle, H. S. Overexpression of the apple MpNPR1 gene confers increased disease resistance in Malus × domestica . Mol. Plant-Microbe Interact. 20 , 1568–1580 (2007).
Jin, W. et al. Improved cold-resistant performance in transgenic grape ( Vitis vinifera L.) overexpressing cold-inducible transcription factors AtDREB1b . HortScience 44 , 35–39 (2009).
Geng, J., Wei, T., Wang, Y., Huang, X. & Liu, J. H. Overexpression of PtrbHLH , a basic helix-loop-helix transcription factor from Poncirus trifoliata , confers enhanced cold tolerance in pummelo ( Citrus grandis ) by modulation of H 2 O 2 level via regulating a CAT gene. Tree Physiol. 39 , 2045–2054 (2019).
PubMed CAS Google Scholar
Soyk, S. et al. Variation in the flowering gene SELF PRUNING 5G promotes day-neutrality and early yield in tomato. Nat. Genet. 49 , 162–168 (2017).
Tränkner, C. et al. Over-expression of an FT-homologous gene of apple induces early flowering in annual and perennial plants. Planta 232 , 1309–1324 (2010).
Endo, T. et al. Ectopic expression of an FT homolog from Citrus confers anearly flowering phenotype on trifoliate orange ( Poncirus trifoliata L. Raf.). Transgenic Res. 14 , 703–712 (2005).
Srinivasan, C., Dardick, C., Callahan, A. & Scorza, R. Plum ( Prunus domestica ) trees transformed with poplar FT1 result in altered architecture, dormancy requirement, and continuous flowering. PLoS ONE 7 , 1–11 (2012).
Varkonyi‐Gasic, E. et al. Mutagenesis of kiwifruit CENTRORADIALIS‐like genes transforms a climbing woody perennial with long juvenility and axillary flowering into a compact plant with rapid terminal flowering. Plant Biotechnol. J. 17 , 869–880 (2019).
Dandekar, A. M. et al. Effect of down-regulation of ethylene biosynthesis on fruit flavor complex in apple fruit. Transgenic Res. 13 , 373–384 (2004).
Atkinson, R. G. et al. Dissecting the role of climacteric ethylene in kiwifruit ( Actinidia chinensis ) ripening using a 1-aminocyclopropane-1-carboxylic acid oxidase knockdown line. J. Exp. Bot. 62 , 3821–3835 (2011).
López-Gómez, R. et al. Ripening in papaya fruit is altered by ACC oxidase cosuppression. Transgenic Res. 18 , 89–97 (2009).
Gao, M. et al. Gene expression and ethylene production in transgenic pear ( Pyrus communis cv.‘La France’) with sense or antisense cDNA encoding ACC oxidase. Plant Sci. 173 , 32–42 (2007).
Park, J.-I. et al. Modification of sugar composition in strawberry fruit by antisense suppression of an ADP-glucose pyrophosphorylase. Mol. Breed. 17 , 269–279 (2006).
Cutanda-Perez, M. C. et al. Ectopic expression of VlmybA1 in grapevine activates a narrow set of genes involved in anthocyanin synthesis and transport. Plant Mol. Biol. 69 , 633–648 (2009).
Lin-Wang, K. et al. An R2R3 MYB transcription factor associated with regulation of the anthocyanin biosynthetic pathway in Rosaceae. BMC Plant Biol. 10 , 50 (2010).
Pons, E. et al. Metabolic engineering of β-carotene in orange fruit increases its in vivo antioxidant properties. Plant Biotechnol. J. 12 , 17–27 (2014).
Rugini, E., Bashir, M. A., Cristofori, V., Ruggiero, B. & Silvestri, C. A review of genetic improvement of main fruit trees through modern biotechnological tools and considerations of the cultivation and research of the engineered plant restrictions. Pakistan J. Agric. Sci . 57 , 17–42 (2020).
Albacete, A. et al. Unravelling rootstock×scion interactions to improve food security. J. Exp. Bot. 66 , 2211–2226 (2015).
Orbović, V. Editorial: new developments in Agrobacterium-Mediated transformation of tree fruit crops. Front. Plant Sci . https://doi.org/10.3389/fpls.2019.01253 (2019).
Mitter, N. & Gleeson, M. Acceptance of disease-resistant GM rootstocks for non-GM fruit. In: XXIX International Horticultural Congress on Horticulture: Sustaining Lives, Livelihoods and Landscapes (IHC2014): III 1124 . pp 91–96 (2014).
Smolka, A., Li, X.-Y., Heikelt, C., Welander, M. & Zhu, L.-H. Effects of transgenicrootstocks on growth and development of non-transgenic scion cultivars inapple. Transgenic Res. 19 , 933–948 (2010).
Dandekar, A. M. et al. Trans-graft protection against Pierce’s disease mediated by transgenic grapevine rootstocks. Front. Plant Sci. 10 , 84 (2019).
Chen, L. et al. A method for the production and expedient screening of CRISPR/Cas9-mediated non-transgenic mutant plants. Hortic. Res. 5 , 1–12 (2018).
Tian, S. et al. Engineering herbicide-resistant watermelon variety through CRISPR/Cas9-mediated base-editing. Plant Cell Rep. 37 , 1353–1356 (2018).
Hu, B. et al. Engineering non-transgenic gynoecious cucumber using an improved transformation protocol and optimized CRISPR/Cas9 system. Mol. Plant 10 , 1575–1578 (2017).
Zhou, J., Wang, G. & Liu, Z. Efficient genome editing of wild strawberry genes, vector development and validation. Plant Biotechnol. J. 16 , 1868–1877 (2018).
Nekrasov, V. et al. Rapid generation of a transgene-free powdery mildew resistant tomato by genome deletion. Sci. Rep. 7 , 1–6 (2017).
Veillet, F. et al. Transgene-free genome editing in tomato and potato plants using Agrobacterium -mediated delivery of a CRISPR/Cas9 cytidine base editor. Int. J. Mol. Sci. 20 , 1–10 (2019).
Zhou, J. et al. Application and future perspective of CRISPR/Cas9 genome editing in fruit crops. J. Integr. Plant Biol. 62 , 269–286 (2020).
Sattar, M. N. et al. CRISPR/Cas9: a practical approach in date palm genome editing. Front. Plant Sci. 8 , 1469 (2017).
Nishitani, C. et al. Efficient genome editing in apple using a CRISPR/Cas9 system. Sci. Rep. 6 , 1–8 (2016).
Malnoy, M. et al. DNA-free genetically edited grapevine and apple protoplast using CRISPR/Cas9 ribonucleoproteins. Front. Plant Sci. 7 , 1–9 (2016).
Osakabe, Y. et al. CRISPR–Cas9-mediated genome editing in apple and grapevine. Nat. Protoc. 13 , 2844–2863 (2018).
Metje-Sprink, J., Menz, J., Modrzejewski, D. & Sprink, T. DNA-free genome editing: past, present and future. Front Plant Sci. 9 , 1957 (2019).
Woo, J. W. et al. DNA-free genome editing in plants with preassembled CRISPR-Cas9 ribonucleoproteins. Nat. Biotechnol. 33 , 1162–1164 (2015).
Kim, J., Chang, C. & Tucker, M. L. To grow old: regulatory role of ethylene and jasmonic acid in senescence. Front Plant Sci. 6 , 20 (2015).
Ghogare, R., Williamson-Benavides, B., Ramírez-Torres, F. & Dhingra, A. CRISPR-associated nucleases: the Dawn of a new age of efficient crop improvement. Transgenic Res. 29 , 1–35 (2020).
Begemann, M. B. et al. Precise insertion and guided editing of higher plant genomes using Cpf1 CRISPR nucleases. Sci. Rep. 7 , 1–6 (2017).
Jia, H., Zou, X., Orbovic, V. & Wang, N. in Plant Genome Editing with CRISPR Systems (ed Qi, Y.) 235–241 (Springer, 2019).
Yao, J. L. et al. Ectopic expression of the PISTILLATA homologous MdPI inhibits fruit tissue growth and changes fruit shape in apple. Plant Direct 2 (2018).
Jia, D. et al. Overexpression of a novel apple NAC transcription factor gene, MdNAC1, confers the dwarf phenotype in transgenic apple ( Malus domestica ). Genes (Basel) 9 , 229 (2018).
Pasquali, G., Biricolti, S., Locatelli, F., Baldoni, E. & Mattana, M. Osmyb4 expression improves adaptive responses to drought and cold stress in transgenic apples. Plant Cell Rep. 27 , 1677–1686 (2008).
Shao, X. et al. Using CRISPR/Cas9 genome editing system to create MaGA20ox2 gene-modified semi-dwarf banana. Plant Biotechnol. J. 18 , 17–19 (2020).
Tripathi, J. N. et al. CRISPR/Cas9 editing of endogenous banana streak virus in the B genome of Musa spp. overcomes a major challenge in banana breeding. Commun. Biol. 2 , 1–11 (2019).
Tripathi, L., Mwaka, H., Tripathi, J. N. & Tushemereirwe, W. K. Expression of sweet pepper Hrap gene in banana enhances resistance to Xanthomonas campestris pv. musacearum . Mol. Plant Pathol. 11 , 721–731 (2010).
PubMed PubMed Central CAS Google Scholar
Namukwaya, B. et al. Transgenic banana expressing Pflp gene confers enhanced resistance to Xanthomonas wilt disease. Transgenic Res. 21 , 855–865 (2012).
Kaur, N. et al. CRISPR/Cas9 directed editing of lycopene epsilon-cyclase modulates metabolic flux for β-carotene biosynthesis in banana fruit. Metab. Eng. 59 , 76–86 (2020).
Song, Gqing & Chen, Q. Overexpression of the MADS-box gene K-domain increases the yield potential of blueberry. Plant Sci. 276 , 22–31 (2018).
Qi, X., Liu, C., Song, L., Li, Y. & Li, M. PaCYP78A9 , a cytochrome P450, regulates fruit size in sweet cherry ( Prunus avium L.). Front. Plant Sci. 8 , 1–14 (2017).
Song, G. et al. Engineering cherry rootstocks with resistance to Prunus necrotic ring spot virus through RNA i‐mediated silencing. Plant Biotechnol. J. 11 , 702–708 (2013).
Fagoaga, C. et al. Engineering of gibberellin levels in citrus by sense and antisense overexpression of a GA 20-oxidase gene modifies plant architecture. J. Exp. Bot. 58 , 1407–1420 (2007).
Hao, G., Stover, E. & Gupta, G. Overexpression of a modified plant thionin enhances disease resistance to citrus canker and huanglongbing (HLB). Front. Plant Sci. 7 , 1–11 (2016).
Cervera, M. et al. Transgenic expression in citrus of single-chain antibody fragments specific to Citrus tristeza virus confers virus resistance. Transgenic Res. 19 , 1001–1015 (2010).
Vigne, E., Komar, V. & Fuchs, M. Field safety assessment of recombination in transgenic grapevines expressing the coat protein gene of Grapevine fanleaf virus. Transgenic Res. 13 , 165–179 (2004).
Zok, A. et al. Effect of Medicago sativa ferritin gene on stress tolerance in transgenic grapevine. Plant Cell. Tissue Organ Cult. 100 , 339–344 (2010).
Kim, M. et al. Transformation of carotenoid biosynthetic genes using a micro-cross section method in kiwifruit ( Actinidia deliciosa cv. Hayward). Plant Cell Rep. 29 , 1339–1349 (2010).
Wang, C. et al. Molecular cloning and heterologous expression analysis of JrVTE1 gene from walnut ( Juglans regia ). Mol. Breed. 35 , 1–12 (2015).
Rubio, J. et al. Silencing of one copy of the translation initiation factor eIFiso4G in Japanese plum ( Prunus salicina ) impacts susceptibility to Plum pox virus (PPV) and small RNA production. BMC Plant Biol. 19 , 1–12 (2019).
Martín-Pizarro, C., Triviño, J. C. & Posé, D. Functional analysis of the TM6 MADS-box gene in the octoploid strawberry by CRISPR/Cas9-directed mutagenesis. J. Exp. Bot. 70 , 885–895 (2019).
Lee, Y. K. & Kim, I. J. Modulation of fruit softening by antisense suppression of endo-β-1,4-glucanase in strawberry. Mol. Breed. 27 , 375–383 (2011).
Yu, Q. H. et al. CRISPR/Cas9-induced targeted mutagenesis and gene replacement to generate long-shelf life tomato lines. Sci. Rep. 7 , 1–9 (2017).
CAS Google Scholar
Klap, C. et al. Tomato facultative parthenocarpy results from Sl AGAMOUS-LIKE 6 loss of function. Plant Biotechnol. J. 15 , 634–647 (2017).
Li, X. et al. Lycopene is enriched in tomato fruit by CRISPR/Cas9-mediated multiplex genome editing. Front. Plant Sci. 9 , 1–12 (2018).
Tang, X. et al. Overexpression of Pto activates defense responses and confers broad resistance. Plant Cell 11 , 15–29 (1999).
Download references
Acknowledgements
M.L.-G., P.S.G.-C., P.C., and T.C. would like to acknowledge funding from MINECO, Spain (PGC2018-097655-B-I00 to P Christou), Generalitat de Catalunya Grant 2017 SGR 828 to the Agricultural Biotechnology and Bioeconomy Unit (ABBU). P.S.G.-C. was supported through an Agrotecnio postdoctoral fellowship. A.D. acknowledges the support from Washington State University Agriculture Center Research Hatch grant WNP00011.
Author information
Authors and affiliations.
Department of Crop and Forest Sciences, University of Lleida-Agrotecnio CERCA Center, Lleida, 25198, Spain
Maria Lobato-Gómez, Teresa Capell, Paul Christou & Patricia Sarai Girón-Calva
Department of Horticulture, Washington State University, PO Box, 646414, Pullman, WA, USA
Seanna Hewitt & Amit Dhingra
ICREA, Catalan Institute for Research and Advanced Studies, 08010, Barcelona, Spain
Paul Christou
You can also search for this author in PubMed Google Scholar

Contributions
P.C and A.D. conceived the idea. M.L.-G. and P.S.G.-C. planned the outline. M.L.-G., S.L.H., and P.S.G.-C. collected the literature and wrote the paper. M.L.-G. and P.S.G.-C. prepared the figure and tables. P.C., T.C., and A.D. critically reviewed and improved the paper. All authors approved the final version.
Corresponding author
Correspondence to Patricia Sarai Girón-Calva .
Ethics declarations
Conflict of interest.
The authors declare no competing interests.
Supplementary information
Rights and permissions.
Open Access This article is licensed under a Creative Commons Attribution 4.0 International License, which permits use, sharing, adaptation, distribution and reproduction in any medium or format, as long as you give appropriate credit to the original author(s) and the source, provide a link to the Creative Commons license, and indicate if changes were made. The images or other third party material in this article are included in the article’s Creative Commons license, unless indicated otherwise in a credit line to the material. If material is not included in the article’s Creative Commons license and your intended use is not permitted by statutory regulation or exceeds the permitted use, you will need to obtain permission directly from the copyright holder. To view a copy of this license, visit http://creativecommons.org/licenses/by/4.0/ .
Reprints and permissions
About this article
Cite this article.
Lobato-Gómez, M., Hewitt, S., Capell, T. et al. Transgenic and genome-edited fruits: background, constraints, benefits, and commercial opportunities. Hortic Res 8 , 166 (2021). https://doi.org/10.1038/s41438-021-00601-3
Download citation
Received : 25 January 2021
Revised : 14 April 2021
Accepted : 20 May 2021
Published : 17 July 2021
DOI : https://doi.org/10.1038/s41438-021-00601-3
Share this article
Anyone you share the following link with will be able to read this content:
Sorry, a shareable link is not currently available for this article.
Provided by the Springer Nature SharedIt content-sharing initiative
This article is cited by
Addressing the un sustainable development goals of woody plants through genetic engineering.
- Rafael Urrea-López
- Andrés Gatica-Arias
Plant Cell, Tissue and Organ Culture (PCTOC) (2024)
N-acylhomoserine lactonase-based hybrid nanoflowers: a novel and practical strategy to control plant bacterial diseases
Journal of Nanobiotechnology (2022)
Quick links
- Explore articles by subject
- Guide to authors
- Editorial policies

- Most Popular
- Discussion & Debate
- Saved Stories
The simple, ethical case for gene editing
Gene editing is a form of ‘ultimate cure’: it treats disease at its very root. This edited extract from the new book, Genes for Life, explores the ethical implications of this rapidly evolving technology
By Dr Christopher Gyngell and Professor Julian Savulescu , University of Melbourne

Published 17 January 2018
For over three decades, scientists have had the ability to alter the genomes of other species of animals. Using viruses to alter DNA sequences, scientists were able to create a range of transgenic animals — with altered physical, cognitive and social characteristics.
In 2007, scientists at Case Western Reserve University used viruses to alter a gene called PEPCK-A in mice. The resulting transgenic mice could run for six kilometres without a break — 30 times longer than a normal mouse’s limit of 200 metres.

But, early modes of genetic engineering were imprecise and inefficient. As a result, these technologies never had serious potential to be clinically useful modifiers of human DNA.
The last few years have seen the development of a number of efficient, more precise genetic engineering techniques. These techniques have been given the collective name ‘gene editing technologies’, to reflect their increased accuracy over previous methods. The most powerful gene editing technology is the CRISPR-cas9 system . CRISPR-cas9 consists of two parts, a DNA cutting enzyme (the cas9 part), and a guide sequence (the CRISPR part).
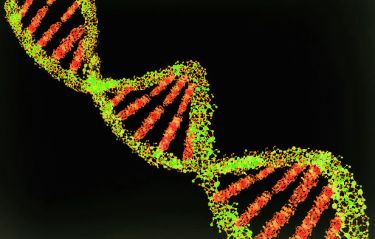
Health & Medicine
Genes, numbers and the holy grail of cancer prevention
When the guide DNA binds with a complementary DNA sequence in the cells it enters, it triggers the cas9 enzyme to cut, making a double-sided break to the DNA sequence. CRISPR-cas9 is used by bacteria as a defence against viruses, cutting viral DNA into small, non-functional fragments.
In 2012, a team at UC Berkeley showed that CRISPR-cas9 could be modified in the lab so that it could target virtually any DNA sequence. This allowed researchers to cut DNA virtually anywhere in the genome. Furthermore, they demonstrated that after the DNA was broken, DNA repair mechanisms could be recruited to add novel genetic material to the site of break. This gave researchers the ability to delete, add, or modify DNA sequences.
In April 2015, it was announced that CRISPR had been used for the first time to make edits in human embryos. This was the first instance of what we will call human germline gene editing or GGE.
The study , conducted in China, targeted the gene responsible for producing part of the haemoglobin molecule — with mixed success. In February 2016, the United Kingdom became the first country to officially approve gene-editing research in human embryos.
Some public interest groups, including the United Nations Educational Scientific and Cultural Organization (UNESCO) have called for an international ban on any gene-editing research in human embryos. The US-based National Institutes of Health, maintained that performing such research would cross “ a line that should not be crossed ”.

As a result of this rapid development of gene-editing technologies, governments around the world need to examine their regulatory frameworks for reproductive technologies.
Currently, GGE is highly restricted through legislation or through guidelines in many countries . However, there are strong moral reasons in favour of pursuing GGE.
Many diseases have a simple genetic mechanism, or are strongly influenced by genes. The development of gene editing could be a vital tool in our fight against these Mendelian diseases. In some cases, using GGE will be the only way that individuals can avoid fatal disease in their children.
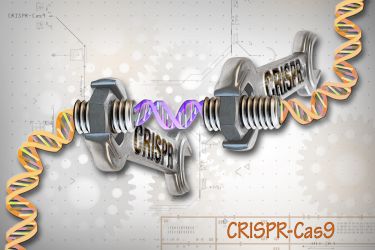
Sciences & Technology
Genetic snip and snap
Further in the future, GGE could provide a vital tool in the fight against polygenic diseases like cancer, diabetes and heart disease – which are among the leading causes of mortality worldwide .
Currently GGE is a very young technology. It should only be used for the above therapeutic purposes if it is shown to be safe through research. GGE is like all other medical technologies in this regard.
The central question currently facing governments around the world with regard to GGE is not whether GGE should be used for therapy, but whether it should be permitted in research.
For current legislative bans on GGE to be justified on moral grounds, there must be significant moral reasons against its use in research or reproduction.
Some believe we should not allow GGE because of concerns about the moral standing of early human embryos, believing that embryos have the same moral status as persons.
But this position is widely inconsistent with how other accepted technologies are regulated. Many jurisdictions permit embryo research that involves the destruction of the embryo for research aimed at improving fertility.
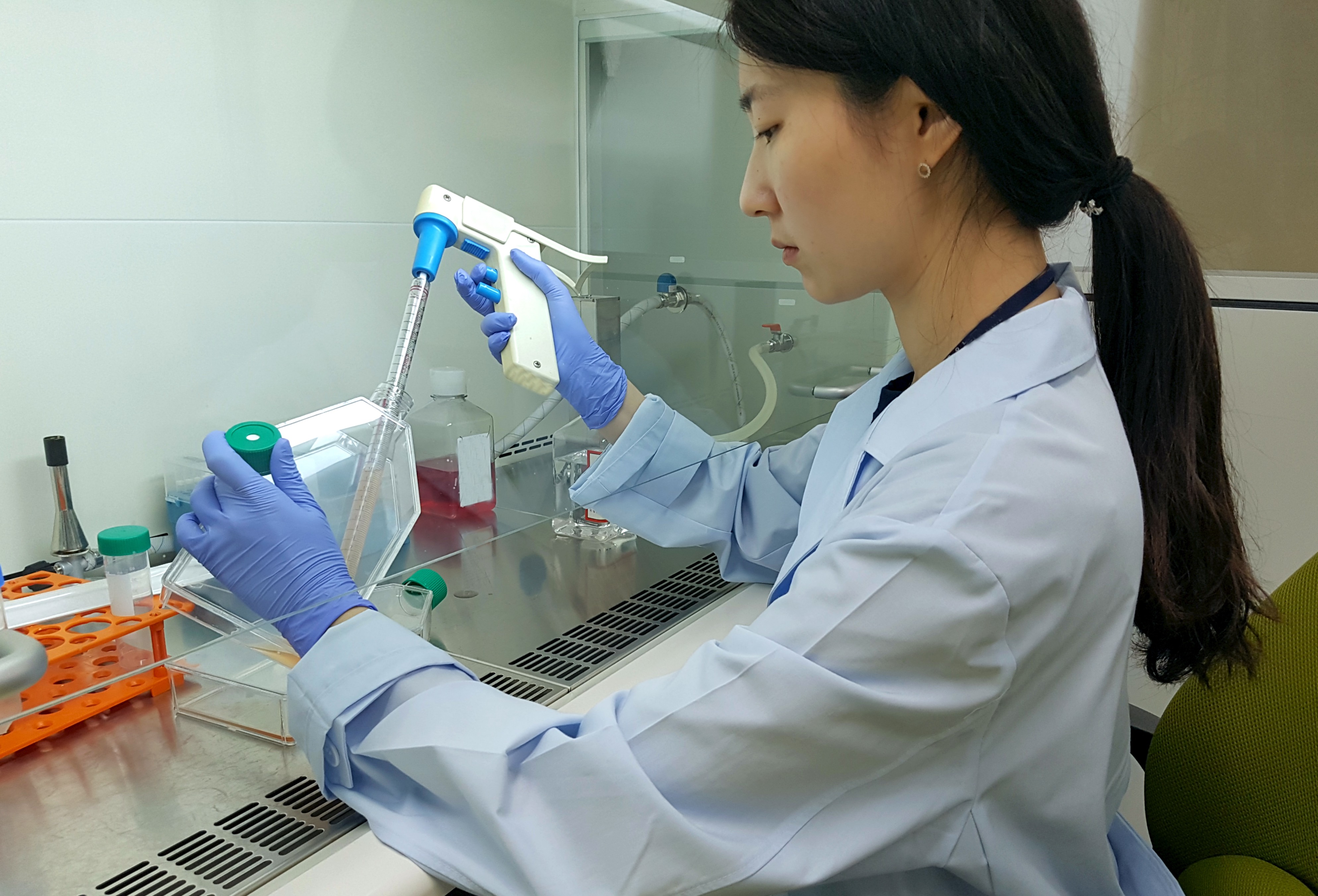
Even if we assume that early human embryos do have a high moral status, this does not count against all forms of GGE research. Some GGE research could be done entirely on gametes. For example, researchers could attempt to edit the gene that causes cystic fibrosis, CFTR, in spermatogonial stem cells and derive sperm carrying the corrected gene.
Some arguments against GGE focus not on its use in research, but its use in reproduction. Some think that any moral reasons against GGE in reproduction leads to reasons against its use in research, as they believe that GGE research will inevitably lead to reproductive applications.
Another common concern about GGE is that it is unjust. For example, American environmentalist, author, and journalist Bill McKibben states : “These would be mere consumer decisions — but that also means that they would benefit the rich far more than the poor. They would take the gap in power, wealth, and education that currently divides both our society and the world at large, and write that division into our very biology.”
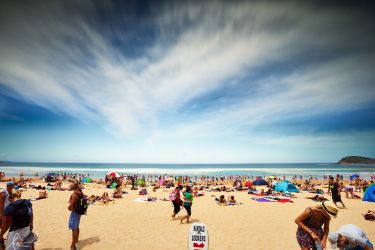
Tailored treatment turns up heat on melanomas
But, there are ways that GGE may help remedy existing injustices. The most obvious way in which GGEs will remedy injustice is by reducing rates of disease. Many diseases are caused only by quirks of our biology. Some people randomly develop diseases, while others do not. They can cause pain, reduce lifespan, and limit one’s ability to pursue one’s goals.
Hence, there is a simple moral case for allowing GGE — it has immediate utility in research and both short- and long-term potential in therapy.
Governments around the world need to radically shift their regulatory approach to GGE. At the very least, research into GGE ought to be permitted even if at this stage we ban GGE to produce babies because the science is in its infancy.
But if the science progresses, we would have the same moral obligation to employ GGE as we have to employ any other cure for disease.
Genes for Life , written by Australian and International academics and writers, provides information about the latest advances in genetics, how people can benefit and, conversely, be harmed by this ever evolving and exciting technology. The book is co-edited by Professor Martin Delatycki, Associate Professor Grant Blashki and Helen Sykes AM, Director of Future Leaders , and is available online here .
Banner: Getty Images
Featured individuals

Professor Julian Savulescu
Distinguished International Visiting Professor, Melbourne Law School, University of Melbourne; Visiting Professorial Fellow, Murdoch Children's Research Institute; Uehiro Chair in Practical Ethics Director, Oxford Uehiro Centre for Practical Ethics; Co-Director, Wellcome Centre for Ethics and Humanities, University of Oxford

Dr Christopher Gyngell
Research Fellow, Biomedical Ethics, Murdoch Children’s Research Institute; Co-Deputy Director of Health Law and Ethics Network, University of Melbourne
Find out more about research in this faculty
Content Card Slider
Subscribe for your weekly email digest.
By subscribing, you agree to our privacy policy.
Acknowledgement of country
We acknowledge Aboriginal and Torres Strait Islander people as the Traditional Owners of the unceded lands on which we work, learn and live. We pay respect to Elders past, present and future, and acknowledge the importance of Indigenous knowledge in the Academy.
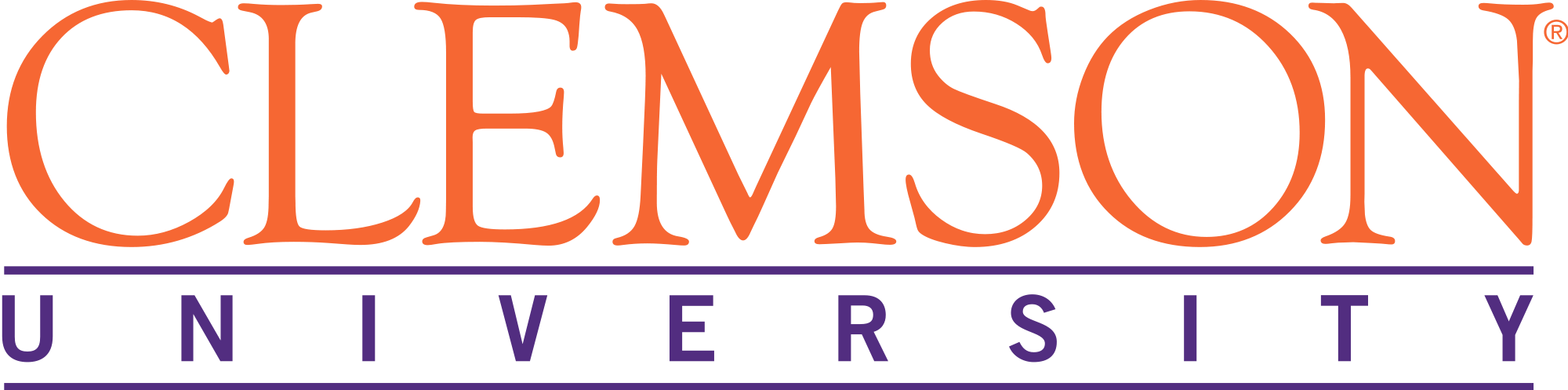
Want to create or adapt books like this? Learn more about how Pressbooks supports open publishing practices.
18 Human Genetic Engineering
Melissa Nolan
18.1 Introduction
- Gene – units of heredity transferred from a parent to offspring, contained in DNA
- Gene editing – deliberate manipulation of the genetic material to achieve desired results
- Human Genetic Engineering (HGE) – gene editing applied to human cells
- CRISPR/Cas9 – gene editing technology derived from bacterial immune system
- Germline gene editing – a process which alters the genome of an embryo, so that the entire organism has altered genes and can pass those genes down to offspring
- Somatic gene editing – a process which alters the genome of just a few cells in an organism, so that the altered genes are not passed down to offspring
- Recombinant DNA – genetic material from multiple sources
- Congenital disease – a disease present from birth, typically caused by some genetic factor
- Eugenics – the study of how to arrange reproduction within a human population to increase the occurrence of heritable characteristics regarded as desirable
- Technological determinism – technology determines the development of its social structure and cultural values or regulations
- Cultural determinism – the culture we are raised presents certain issues which necessitate the development of a specific technology
- Legacy thinking – using thinking strategies and actions which are outdated and no longer serve the purpose they once did
- Antibodies – proteins produced by an organism as a result of their immune response
Learning Objectives
By the end of this chapter, students should be able to:
- Understand the basic principles of gene editing and their applications.
- Understand the history of gene editing
- Identify potential ethical concerns regarding human genetic engineering
Those beautiful blue eyes you inherited from your mother are actually a result of a complex science known as Genetics. The scientific field of genetics studies genes in our DNA. Genes are units of heredity transferred from a parent to offspring and determine some characteristic of offspring. Your genes are responsible for coding all of your traits- including hair color, eye color, and so on. In recent years, scientists began exploring the concept of gene editing , which is the deliberate manipulation of genetic material to achieve desired results. Gene editing can potentially alter any given trait in an organism- from height to hair texture to susceptibility for certain diseases.
Gene editing applied to humans is referred to as Human Genetic Engineering , or HGE. There is extensive debate in and out of the scientific community regarding the ethics of HGE. Much of this debate stems from how this technology will affect society, and vice versa. Individuals may harbor concerns about the rise of “designer babies” or scientists “playing God” by determining the traits of an individual. On the contrary, HGE presents potential cures to diseases caused by genetic mutations. Human Genetic Engineering (HGE) is a novel technology which presents various ethical concerns and potential consequences. HGE should be approached cautiously and with extensive governmental regulation given it’s history, it’s current state, and the potential it has to change the world in the future.
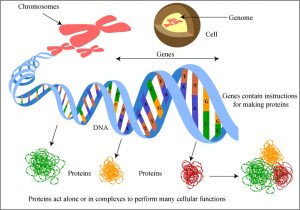
“ Genetic Encoding of Proteins ” by MIT OpenCourseWare is licensed under CC BY-NC-SA 2.0
18.2 What is HGE
Key Takeaway
HGE encompasses a variety of methods which all work to produce a deliberate change in the human genome. The most common and prevalent way to edit the human genome is via CRISPR/Cas9 . CRISPR stands for clustered regularly interspaced short palindromic repeats, and Cas9 is a protein that functions as scissors to cut DNA/genes. The CRISPR/Cas9 system originally developed as a part of a bacteria’s immune system, which can recognize repeats in DNA of invading viruses, then cut them out. Since then, scientists have harnessed the CRISPR/Cas9 system to cut DNA sequences of their choice and then insert new DNA sequences in their place.
The CRISPR/Cas9 system allows for designer genomes, and rapid engineering of any cell’s programming. With the use of CRISPR/Cas9, scientists can cut out certain traits from an individual’s cells and insert new traits into those same cells.
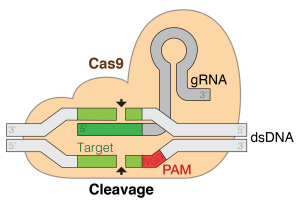
“ CRISPR Cas9 System ” by Marius Walter is licensed under CC-BY-SA-4.0
18.3 History of Gene Editing
Gene therapy concepts were initially introduced in the 1960s, utilizing outdated methods, such as recombinant DNA technology and viral vectors, to edit microorganisms’ genomes. Recombinant DNA consists of genetic material from multiple sources. The first experiments involved transferring a genome from one bacteria to another via a viral vector. Soon after was the first successful transformation of human cells with foreign DNA. The success of the experiment prompted public concern over the ethics of gene therapy, and led to political regulation. In the gene therapy report of the President’s Commission in the United States, germline genome editing was deemed problematic over somatic genome editing . Also, non-medical genome editing was deemed problematic over medical genome editing. Germline genome editing occurs when scientists alter the genome of an embryo, so that the entire organism has altered genes and the traits can be passed to offspring. Somatic genome editing involves editing only a few cells in the entire organism so that traits can not be passed down to offspring. In response to the report, the rDNA Advisory Committee of the National Institutes of Health was formed and proposed the first guidelines for the gene therapy clinical trials. This is an example of technological determinism , in which technology determines the development of its social structure and cultural values or regulations.
In the past few decades, gene editing has advanced exponentially, introducing state-of-the-art technologies such as the CRISPR/Cas9 system, which was developed to induce gene modifications at very specific target sites. Thus, gene editing became a major focus for medical research (Tamura, 2020). Gene editing has led to the potential for development of treatment strategies for a variety of diseases and cancers. So far, somatic genome editing has shown promise in treating leukemia, melanoma, and a variety of other diseases. In this way, HGE may be demonstrative of cultural determinism , in which the culture we are raised presents certain issues which necessitate the development of a specific technology.
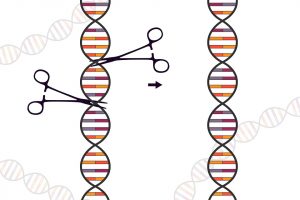
“ DNA CRISPR Scissors ” by Max Pixel is licensed under CC0 1.0
18.4. Impact of HGE on society
Somatic genome editing in HGE via the CRISPR/Cas9 system has proven to be effective at editing specific genome sites. Since 2015, genome editing technologies have been used in over 30 human clinical trials and have shown positive patient outcomes. The treatment of disease may be a positive benefit of HGE, but there are also various potential risks. Various forms of deliberative democracies formed in recent years to address scientific and ethical concerns in HGE. Deliberative democracies affirm the need to justify technological decisions made by citizens and their representatives with experts in the field via deliberation. Overall, the consensus remains that the pros and cons of HGE are not equivalent enough to justify widespread use of the technology.
18.4.1 Pros of HGE
Current human clinical trials show successful transformation of human immune cells to HIV-resistant cells. This implies that HGE may be the cure for HIV(Hu, 2019). Other successful somatic genome editing trials treated myeloma, leukemia, sickle cell disease, various forms of epithelial cancers, and hemophilia. Thus, gene editing has provided novel treatment options for congenital diseases and cancers (Tamaura, 2020). Congenital diseases are those present from birth, and typically have a genetic cause. For these reasons, scientific summits concluded HGE is ethical for research regarding somatic genome editing in congenital diseases and cancers.
18.4.2 Cons of HGE
There are many safety concerns regarding CRISPR applications, mainly in germline genome editing. As a result of technological determinism, a leading group of CRISPR/Cas9 scientists and ethicists met for the international Summit on Human Gene Editing. The summit determined that heritable genome research trials may be permitted only following extensive research on risks and benefits of HGE. However, the summit concluded that federal funding cannot be used to support research involving human embryos with germline editing techniques. These decisions were made to avoid potential risks such as the following.
The major concerns regarding germline genome editing in HGE include: serious injury or disability, a blurry line between therapeutic applications of HGE and medical applications, misapplications, potential for eugenics ( the study of how to arrange reproduction within a human population to increase the occurrence of heritable characteristics regarded as desirable), and inequitable access to the technology.
18.5. Future Outlook
The future of HGE is uncertain and requires immense forethought. The American Society of Human Genetics workgroup developed a position statement on human germline engineering. The statement argues that it is inappropriate to perform germline gene editing that culminates in human pregnancy; and that in vitro(outside of an organism) germline editing should be permitted with appropriate oversight. It also states future clinical human germline editing requires ethical justification, compelling medical rationale, and evidence that supports its clinical usage. Many of these decisions were made based on the potential concerts over the future possibilities of the technology.
At the societal level, there may be concerns related to eugenics, social justice, and accessibility to technology. Eugenics could potentially reinforce prejudice and enforce exclusivity in certain physical traits. Traits can be preselected for, thus labeling some as ‘good’ and others as ‘unfavorable’. This may perpetuate existing racist ideals, for example.
Moreover, germline genome editing may also increase the amount of inequality in a society. Human germline editing is likely to be very expensive and access may be limited to certain geographic regions, health systems, or socioeconomic statuses. Even if human genetic engineering is only used for medical purposes, genetic disease could become an artifact of class, location, or ethnic group. Therefore, preclinical trials are necessary to establish validity, safety, and efficacy before any wide scale studies are initiated.
Others argue that HGE may lessen genetic diversity in a human population, creating a biological monoculture that could lead to disease susceptibility and eventual extinction. Analyses have predicted that there will be negligible effect on diversity and will more likely ensure the health and longevity of humans (Russel, 2010). Legacy thinking may be responsible for the hesitations towards continuing forward with HGE, as there are also many potential pros for genetic engineering. Legacy thinking is using outdated thinking strategies and actions which may not be useful anymore.
In an alternative modernity, we can imagine HGE as an end-all for most congenital diseases and cancers. Moreover, it may be used in germline gene editing to prevent certain birth defects or heritable diseases. So, although HGE has a variety of potential risk factors, there is also great promise for novel medical therapies in the coming decades. The continued use of this technology should be approached cautiously and with extensive governmental regulation, allowing for research regarding its medical applications only.
In 2016, germline gene editing was proven feasible and effective in chickens by leading researchers in genetic engineering, Dimitrov and colleagues. In this study, scientists used CRISPR/Cas9 to target the gene for an antibody / immunoglobulin commonly produced in chickens. Antibodies are proteins produced in immune response. In the resulting population, the chickens grew normally and healthily with modified antibodies which conferred drug resistance. This study was the first to prove that germline editing is both feasible and effective.
Chapter Summary
HGE is a rapidly expanding field of research which presents novel possibilities for the coming decades. HGE utilizes CRISPR/Cas9 gene editing tools to cut out specific genes and replace them with a newly designed gene. As important as this technology is, it is also important to recognize how new it is. Gene therapy research began in the 1960’s, with somatic cell editing only commencing in the past two decades. This has presented many advantages for the potential treatment of congenital diseases, but also presents various risks. Those risks stem from germline gene editing and include eugenics and inequitable access to the technology creating large socio economic divides. In the future, more regulation should be placed on the advancement of HGE research before larger-scale studies take place.
Review Questions
1. What is the primary technology proposed for use in HGE?
A. Recombinant DNA technology
B. CRISPR/Cas9
C. Bacterial Transformation
D. Immunoglobulin
2. When was gene therapy concepts first introduced?
3. What is a major ethical concern regarding HGE addressed in this chapter?
A. Potential for ageism
B. Gene editing is only 50% effective
C. HGE can only be used in Caucasians
D. Potential for eugenics
Food for Thought
- Would you personally employ germline genome editing for your own children? In what scenarios? Why or why not?
- Should technologies such as this be federally regulated or should scientific freedom preside? Justify your reasoning.
Baltimore, D. et. al.(2015). A prudent path forward for genomic engineering and germline gene modification. Science. https://doi.org/10.1126/science.aab1028
Brokowski, C., & Adli, M. (2019). CRISPR Ethics: Moral Considerations for Applications of a Powerful Tool. Journal of Molecular Biology. https://doi.org/10.1016/j.jmb.2018.05.044
Cong, L., Ran, F., & Zhang, F. (2013). Multiplex Genome Engineering Using CRISPR/Cas9 Systems. Science. https://doi.org/10.1126/science.1231143
Dimitrov, L., et. al. (2016). Germline Gene Editing in Chickens by Efficient CRISPR-Mediated Homologous Recombination in Primordial Germ Cells. Plos One. https://doi.org/10.1371/journal.pone.0154303
Hu, C. (2019). Safety of Transplantation of CRISPR CCR5 Modified CD34+ Cells in HIV-Infected Subjects with Hematological Malignancies. U.S National Library of Medicine. https://clinicaltrials.gov/ct2/show/NCT03164135
Ormond, K., et. al.(2017). Human Germline Genome Editing. AJHG. https://doi.org/10.1016/j.ajhg.2017.06.012
Russell P.(2010) The Evolutionary Biological Implications of Human Genetic Engineering, The Journal of Medicine and Philosophy: A Forum for Bioethics and Philosophy of Medicine. https://doi.org/10.1093/jmp/jhq004
Tamura, R., & Toda, M. (2020). Historic Overview of Genetic Engineering Technologies for Human Gene Therapy. Neurologia medico-chirurgica. https://doi.org/10.2176/nmc.ra.2020-0049
Thomas, C. (2020). CRISPR-Edited Allogeneic Anti-CD19 CAR-T Cell Therapy for Relapsed/Refractory B Cell Non-Hodgkin Lymphoma. ClinicalTrials. https://clinicaltrials.gov/show/NCT04637763
Units of heredity transferred from a parent to offspring, contained in DNA.
Deliberate manipulation of the genetic material to achieve desired results.
Gene editing applied to human cells.
Gene editing technology derived from bacterial immune system.
genetic material from multiple sources
A process which alters the genome of an embryo, so that the entire organism has altered genes and can pass those genes down to offspring.
A process which alters the genome of just a few cells in an organism, so that the altered genes are not passed down to offspring.
The idea that a society's technology determines the development of its social and cultural values.
The culture we are raised presents certain issues which necessitate the development of a specific technology.
A disease present from birth, typically caused by some genetic factor.
The study of how to arrange reproduction within a human population to increase the occurrence of heritable characteristics regarded as desirable.
Using thinking strategies and actions which are outdated and no longer serve the purpose they once did.
Proteins produced by an organism as a result of their immune response,
Technology: Where it Started and Where it’s Going Copyright © by Melissa Nolan is licensed under a Creative Commons Attribution-NonCommercial-NoDerivatives 4.0 International License , except where otherwise noted.
Share This Book
Featured Topics
Featured series.
A series of random questions answered by Harvard experts.
Explore the Gazette
Read the latest.
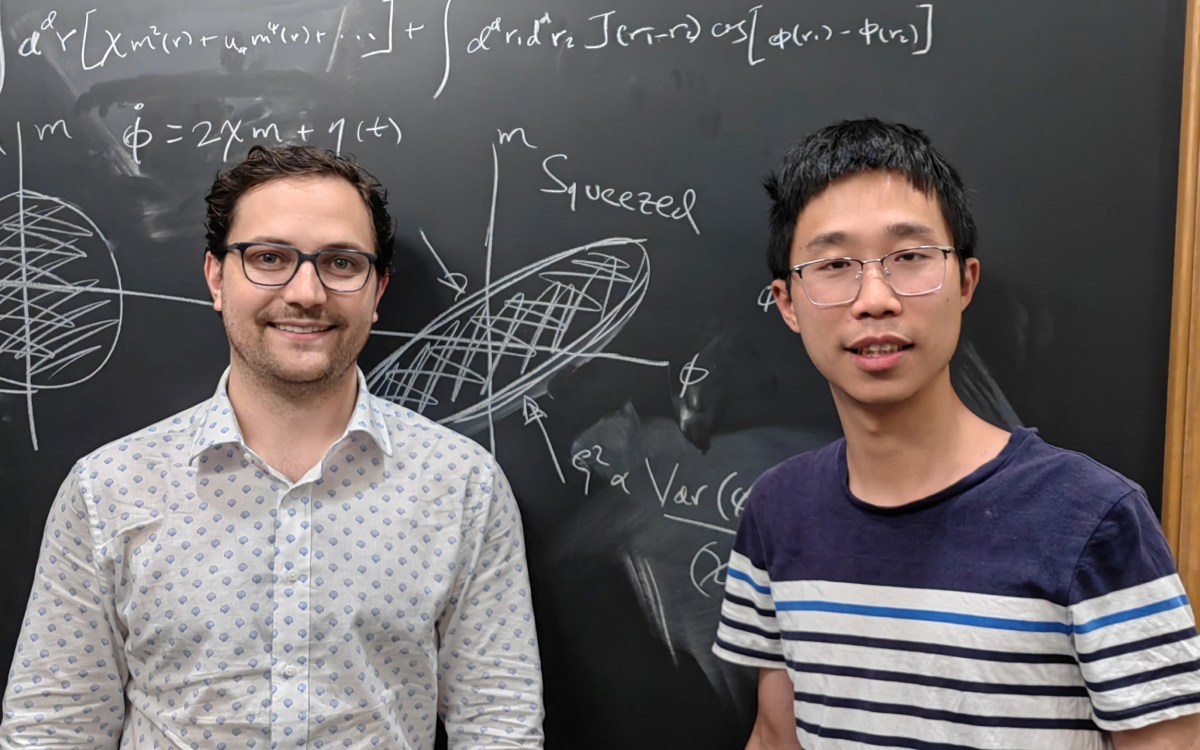
Spin squeezing for all
Should kids play wordle.
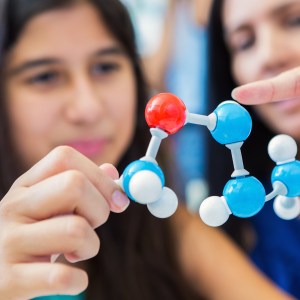
How moms may be affecting STEM gender gap
Perspectives on gene editing.
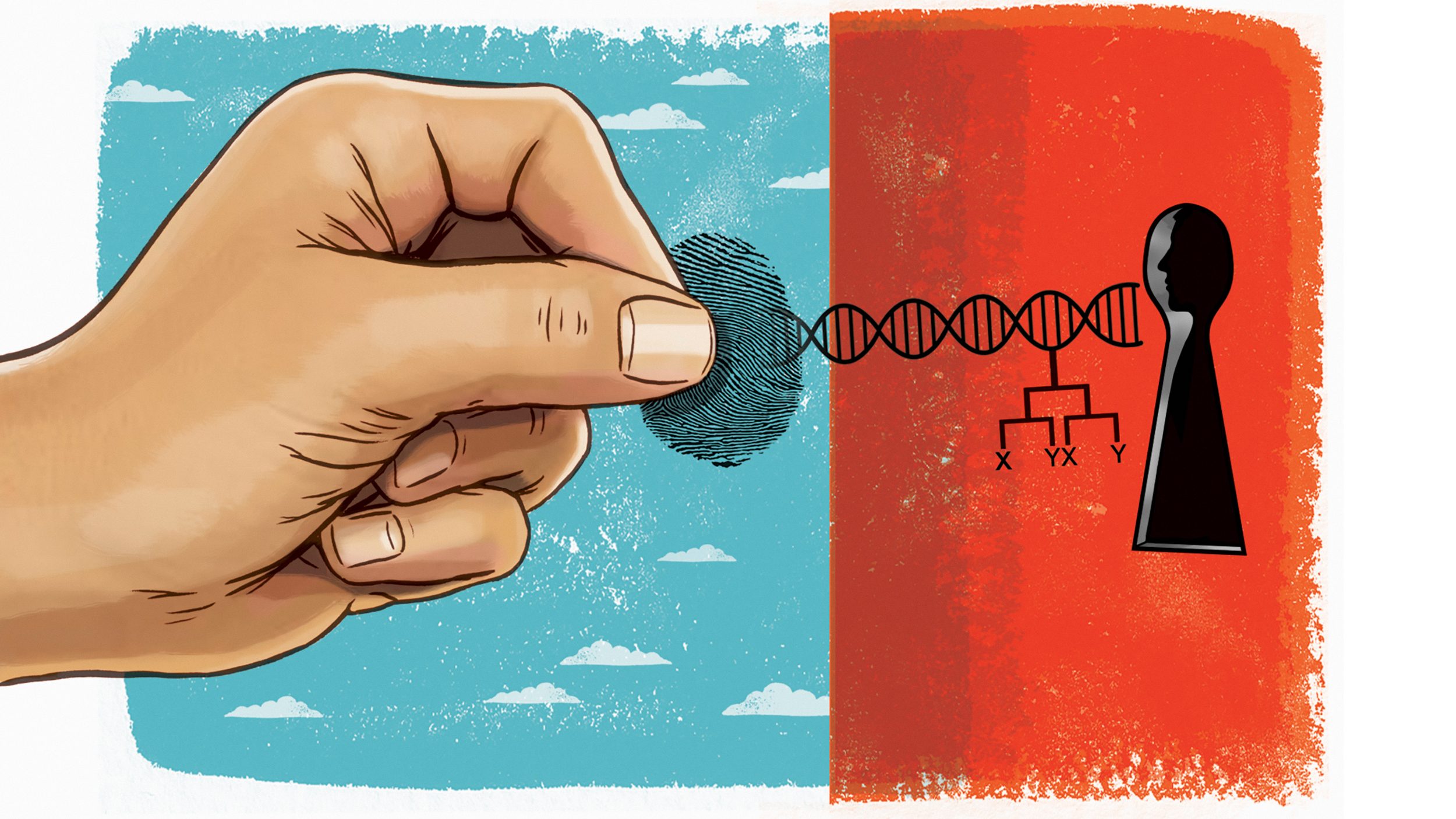
Illustration by Dan Mitchell
Mary Todd Bergman
Harvard Correspondent
Harvard researchers, others share their views on key issues in the field
Medicine is at a turning point, on the cusp of major change as disruptive technologies such as gene, RNA, and cell therapies enable scientists to approach diseases in new ways. The swiftness of this change is being driven by innovations such as CRISPR gene editing , which makes it possible to correct errors in DNA with relative ease.
Progress in this field has been so rapid that the dialogue around potential ethical, societal, and safety issues is scrambling to catch up.
This disconnect was brought into stark relief at the Second International Summit on Human Genome Editing , held in Hong Kong in November, when exciting updates about emerging therapies were eclipsed by a disturbing announcement. He Jiankui, a Chinese researcher, claimed that he had edited the genes of two human embryos, and that they had been brought to term.
There was immediate outcry from scientists across the world, and He was subjected to intense social pressure, including the removal of his affiliations, for having allegedly disregarded ethical norms and his patients’ safety.
Yet as I. Glenn Cohen, faculty director of the Petrie-Flom Center for Health Law Policy, Biotechnology, and Bioethics at Harvard Law School, has said, gene editing comes in many varieties, with many consequences. Any deep ethical discussion needs to take into account those distinctions.
Human genome editing: somatic vs. germline
The germline editing He claimed to have carried out is quite different from the somatic gene therapies that are currently changing the frontiers of medicine. While somatic gene editing affects only the patient being treated (and only some of his or her cells), germline editing affects all cells in an organism, including eggs and sperm, and so is passed on to future generations. The possible consequences of that are difficult to predict.
Somatic gene therapies involve modifying a patient’s DNA to treat or cure a disease caused by a genetic mutation. In one clinical trial, for example, scientists take blood stem cells from a patient, use CRISPR techniques to correct the genetic mutation causing them to produce defective blood cells, then infuse the “corrected” cells back into the patient, where they produce healthy hemoglobin. The treatment changes the patient’s blood cells, but not his or her sperm or eggs.
Germline human genome editing, on the other hand, alters the genome of a human embryo at its earliest stages. This may affect every cell, which means it has an impact not only on the person who may result, but possibly on his or her descendants. There are, therefore, substantial restrictions on its use.
Germline editing in a dish can help researchers figure out what the health benefits could be, and how to reduce risks. Those include targeting the wrong gene; off-target impacts, in which editing a gene might fix one problem but cause another; and mosaicism, in which only some copies of the gene are altered. For these and other reasons, the scientific community approaches germline editing with caution, and the U.S. and many other countries have substantial policy and regulatory restrictions on using germline human genome editing in people.
But many scientific leaders are asking: When the benefits are believed to outweigh the risks, and dangers can be avoided, should science consider moving forward with germline genome editing to improve human health? If the answer is yes, how can researchers do so responsibly?
CRISPR pioneer Feng Zhang of the Broad Institute of Harvard and MIT responded immediately to He’s November announcement by calling for a moratorium on implanting edited embryos in humans. Later, at a public event on “Altering the Human Genome” at the Belfer Center at Harvard Kennedy School (HKS), he explained why he felt it was important to wait:
“The moratorium is a pause. Society needs to figure out if we all want to do this, if this is good for society, and that takes time. If we do, we need to have guidelines first so that the people who do this work can proceed in a responsible way, with the right oversight and quality controls.”
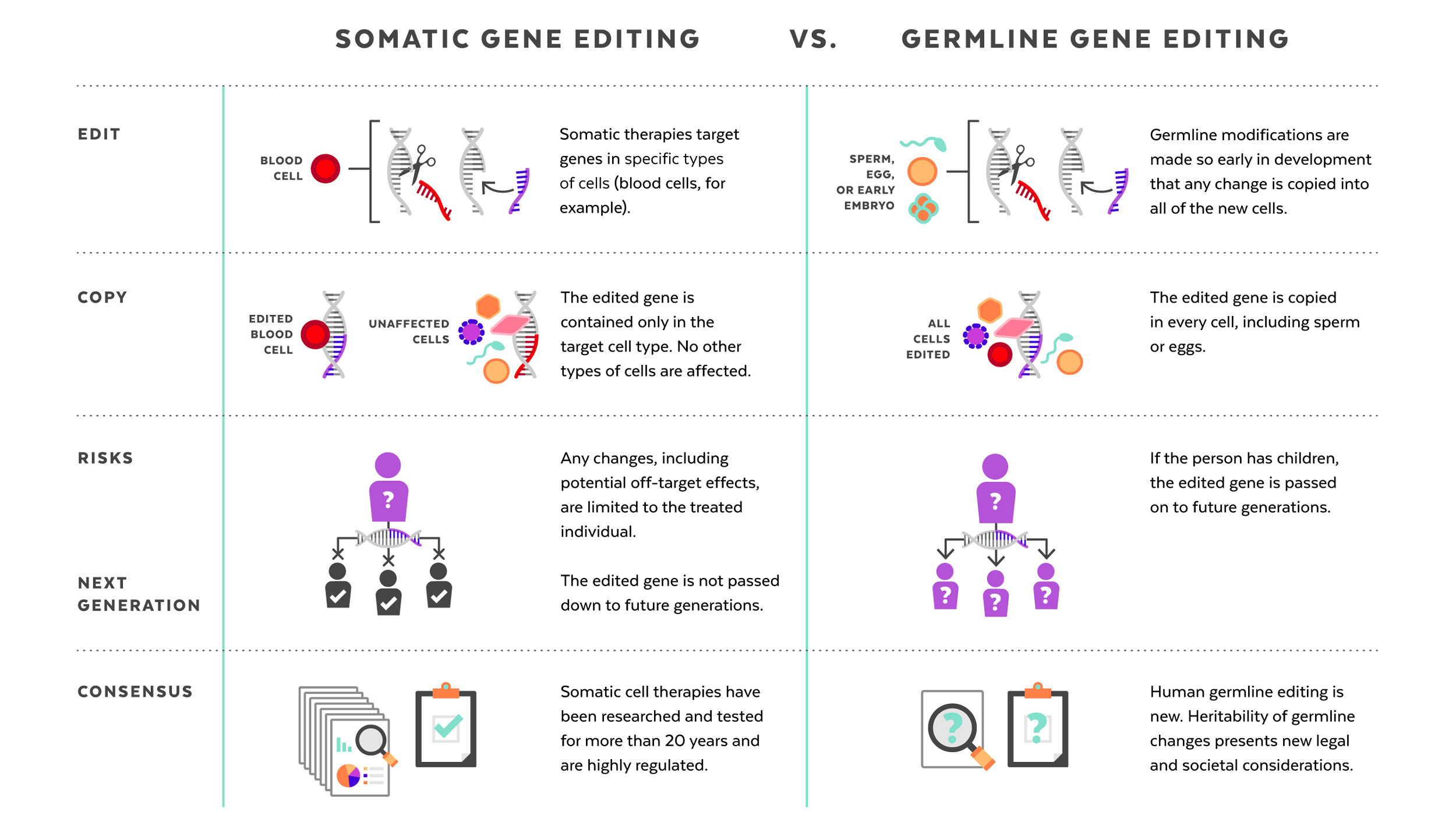
Professors at the University’s schools of medicine, law, business, and government saw He’s announcement as a turning point in the discussion about heritable gene therapies and shared their perspectives on the future of this technology with the Gazette.
Here are their thoughts, issue by issue:
Aside from the safety risks, human genome editing poses some hefty ethical questions. For families who have watched their children suffer from devastating genetic diseases, the technology offers the hope of editing cruel mutations out of the gene pool. For those living in poverty, it is yet another way for the privileged to vault ahead. One open question is where to draw the line between disease treatment and enhancement, and how to enforce it, considering differing attitudes toward conditions such as deafness.
Robert Truog , director of the Center for Bioethics at Harvard Medical School (HMS), provided context:
“This question is not as new as it seems. Evolution progresses by random mutations in the genome, which dwarf what can be done artificially with CRISPR. These random mutations often cause serious problems, and people are born with serious defects. In addition, we have been manipulating our environment in so many ways and exposing ourselves to a lot of chemicals that cause unknown changes to our genome. If we are concerned about making precise interventions to cure disease, we should also be interested in that.
“To me, the conversation around Dr. He is not about the fundamental merits of germline gene editing, which in the long run will almost certainly be highly beneficial. Instead, it’s about the oversight of science. The concern is that with technologies that are relatively easy to use, like CRISPR, how does the scientific community regulate itself? If there’s a silver lining to this cloud, I think it is that the scientific community did pull together to be critical of this work, and took the responsibility seriously to use the tools available to them to regulate themselves.”
When asked what the implications of He’s announcement are for the emerging field of precision medicine, Richard Hamermesh, faculty co-chair of the Harvard Business School/Kraft Precision Medicine Accelerator, said:
“Before we start working on embryos, we have a long way to go, and civilization has to think long and hard about it. There’s no question that gene editing technologies are potentially transformative and are the ultimate precision medicine. If you could precisely correct or delete genes that are causing problems — mutating or aberrant genes — that is the ultimate in precision. It would be so transformative for people with diseases caused by a single gene mutation, like sickle cell anemia and cystic fibrosis. Developing safe, effective ways to use gene editing to treat people with serious diseases with no known cures has so much potential to relieve suffering that it is hard to see how anyone could be against it.
“There is also commercial potential and that will drive it forward. A lot of companies are getting venture funding for interesting gene therapies, but they’re all going after tough medical conditions where there is an unmet need — [where] nothing is working — and they’re trying to find gene therapies to cure those diseases. Why should we stop trying to find cures?
“But anything where you’re going to be changing human embryos, it’s going to take a long time for us to figure out what is appropriate and what isn’t. That has to be done with great care in terms of ethics.”
George Q. Daley is dean of HMS, the Caroline Shields Walker Professor of Medicine, and a leader in stem cell science and cancer biology. As a spokesperson for the organizing committee of the Second International Summit on Human Genome Editing, he responded swiftly to He’s announcement in Hong Kong. Echoing those remarks, he said:
“It’s time to formulate what a clinical path to translation might look like so that we can talk about it. That does not mean that we’re ready to go into the clinic — we are not. We need to specify what the hurdles would be if one were to move forward responsibly and ethically. If you can’t surmount those hurdles, you don’t move forward.
“There are stark distinctions between editing genes in an embryo to prevent a baby from being born with sickle cell anemia and editing genes to alter the appearance or intelligence of future generations. There is a whole spectrum of considerations to be debated. The prospect includes an ultimate decision that we not go forward, that we decide that the benefits do not outweigh the costs.”
Asked how to prevent experiments like He’s while preserving academic freedom, Daley replied:
“For the past 15 years, I have been involved in efforts to establish international standards of professional conduct for stem cell research and its clinical translation, knowing full well that there could be — and has been — a growing number of independent practitioners directly marketing unproven interventions to vulnerable patients through the internet. We advocated so strongly for professional standards in an attempt to ward off the risks of an unregulated industry. Though imperfect, our efforts to encourage a common set of professional practices have been influential.
“You can’t control rogue scientists in any field. But with strongly defined guidelines for responsible professional conduct in place, such ethical violations like those of Dr. He should remain a backwater, because most practitioners will adhere to generally accepted norms. Scientists have a responsibility to come together to articulate professional standards and live by them. One has to raise the bar very high to define what the standards of safety and efficacy are, and what kind of oversight and independent judgment would be required for any approval.
“We have called for an ongoing international forum on human genome editing, and that could take many shapes. We’ve suggested that the national academies of more countries come together — the National Academy of Sciences in the U.S. and the Royal Society in the U.K. are very active here — because these are the groups most likely to have the expertise to convene these kinds of discussions and keep them going.”
Cohen , speaking to the legal consequences of germline human genome editing, said:
“I think we should slow down in our reaction to this case. It is not clear that the U.S. needs to react to Dr. He’s announcement with regulation. The FDA [Food and Drug Administration] already has a strong policy on germline gene editing in place. A rider in the Consolidated Appropriations Act of 2016 — since renewed — would have blocked the very same clinical application of human germline editing He announced, had it been attempted in the U.S.
“The scientific community has responded in the way I’d have liked it to. There is a difference between ‘governance’ and ‘self-governance.’ Where government uses law, the scientific community uses peer review, public censure, promotions, university affiliations, and funding to regulate themselves. In China, in Dr. He’s case, you have someone who’s (allegedly) broken national law and scientific conventions. That doesn’t mean you should halt research being done by everyone who’s law-abiding.
“Public policy or ethical discussion that’s divorced from how science is progressing is problematic. You need to bring everyone together to have robust discussions. I’m optimistic that this is happening, and has happened. It’s very hard to deal with a transnational problem with national legislation, but it would be great to reach international consensus on this subject. These efforts might not succeed, but ultimately they are worth pursuing.”
Professor Kevin Eggan of Harvard’s Department of Stem Cell and Regenerative Biology said, “The question we should focus on is: Will this be safe and help the health of a child? Can we demonstrate that we can fix a mutation that will cause a terrible health problem, accurately and without the risk of harming their potential child? If the answer is yes, then I believe germline human genome editing is likely to gain acceptance in time.
“There could be situations where it could help a couple, but the risks of something going wrong are real. But at this point, it would be impossible to make a risk-benefit calculation in a responsible manner for that couple. Before we could ever move toward the clinic, the scientific community must come to a consensus on how to measure success, and how to measure off-target effects in animal models.
“Even as recently as this past spring and fall, the results of animal studies using CRISPR — the same techniques Dr. He claimed to have used — generated a lot of confusion. There is disagreement about both the quality of the data and how to interpret it. Until we can come to agreement about what the results of animal experiments mean, how could we possibly move forward with people?
“As happened in England with mitochondrial replacement therapy, we should be able to come to both a scientific and a societal consensus of when and how this approach should be used. That’s missing.”
According to Catherine Racowsky, professor of obstetrics, gynecology and reproductive biology at Brigham and Women’s Hospital, constraints on the use of embryos in federally funded research pose barriers to studying the risks and benefits of germline editing in humans. She added:
“Until the work is done, carefully and with tight oversight, to understand any off-target effects of replacing or removing a particular gene, it is inappropriate to apply the technology in the clinical field. My understanding of Dr. He’s case is that there wasn’t a known condition in these embryos, and by editing the genes involved with HIV infection, he could also have increased the risks of susceptibility to influenza and West Nile viruses.
“We need a sound oversight framework, and it needs to be established globally. This is a technology that holds enormous promise, and it is likely to be applied to the embryo, but it should only be applied for clinical purposes after the right work has been done. That means we must have consensus on what applications are acceptable, that we have appropriate regulatory oversight, and, perhaps most importantly, that it is safe. The only way we’re going to be able to determine that these standards are met is to proceed cautiously, with reassessments of the societal and health benefits and the risks.”
Asked about public dialogue around germline human genome editing, George Church , Robert Winthrop Professor of Genetics at HMS, said:
“With in vitro fertilization (IVF), ‘test tube babies’ was an intentionally scary term. But after Louise Brown, the first IVF baby, was born healthy 40 years ago, attitudes changed radically. Ethics flipped 180 degrees, from it being a horrifying idea to being unacceptable to prevent parents from having children by this new method. If these edited twins are proven healthy, very different discussions will arise. For example, is a rate of 900,000 deaths from HIV infection per year a greater risk than West Nile virus, or influenza? How effective is each vaccine?”
Science, technology, and society
Sheila Jasanoff , founding director of the Science, Technology, and Society program at HKS, has been calling for a “global observatory” on gene editing, an international network of scholars and organizations dedicated to promoting exchange across disciplinary and cultural divides. She said:
“The notion that the only thing we should care about is the risk to individuals is very American. So far, the debate has been fixated on potential physical harm to individuals, and not anything else. This is not a formulation shared with other countries in the world, including practically all of Europe. Considerations of risk have equally to do with societal risk. That includes the notion of the family, and what it means to have a ‘designer baby.’
“These were not diseased babies Dr. He was trying to cure. The motivation for the intervention was that they live in a country with a high stigma attached to HIV/AIDS, and the father had it and agreed to the intervention because he wanted to keep his children from contracting AIDS. AIDS shaming is a fact of life in China, and now it won’t be applied to these children. So, are we going to decide that it’s OK to edit as-yet-to-be children to cater to this particular idea of a society?
“It’s been said that ‘the genie is out of the bottle’ with germline human genome editing. I just don’t think that’s true. After all, we have succeeded in keeping ‘nuclear’ inside the bottle. Humanity doesn’t lack the will, intelligence, or creativity to come up with ways for using technology for good and not ill.
“We don’t require students to learn the moral dimensions of science and technology, and that has to change. I think we face similar challenges in robotics, artificial intelligence, and all kinds of frontier fields that have the potential to change not just individuals but the entirety of what it means to be a human being.
“Science has this huge advantage over most professional thought in that it has a universal language. Scientists can hop from lab to lab internationally in a way that lawyers cannot because laws are written in many languages and don’t translate easily. It takes a very long time for people to understand each other across these boundaries. A foundational concept for human dignity? It would not be the same thing between cultures.
“I would like to see a ‘global observatory’ that goes beyond gene editing and addresses emerging technologies more broadly.”
To learn more:
Technology and Public Purpose project, Belfer Center for Science and International Affairs, Harvard Kennedy School of Government, https://www.belfercenter.org/tapp/person
Concluding statement from the Second International Summit on Human Genome Editing. http://www8.nationalacademies.org/onpinews/newsitem.aspx?RecordID=11282018b
A global observatory for gene editing: Sheila Jasanoff and J. Benjamin Hurlbut call for an international network of scholars and organizations to support a new kind of conversation. https://www.nature.com/articles/d41586-018-03270-w
Building Capacity for a Global Genome Editing Observatory: Institutional Design. http://europepmc.org/abstract/MED/29891181
Glenn Cohen’s blog: How Scott Gottlieb is Wrong on the Gene Edited Baby Debacle. http://blog.petrieflom.law.harvard.edu/2018/11/29/how-scott-gottlieb-is-wrong-on-the-gene-edited-baby-debacle/
Gene-Editing: Interpretation of Current Law and Legal Policy. https://www.ncbi.nlm.nih.gov/pmc/articles/PMC5651701/
Forum: Harvard T.H. Chan School of Public Health event on the promises and challenges of gene editing, May 2017: https://theforum.sph.harvard.edu/events/gene-editing/
Petrie-Flom Center Annual Conference: Consuming Genetics: Ethical and Legal Considerations of New Technologies: http://petrieflom.law.harvard.edu/events/details/2019-petrie-flom-center-annual-conference
Share this article
You might like.
Physicists ease path to entanglement for quantum sensing
Early childhood development expert has news for parents who think the popular online game will turn their children into super readers
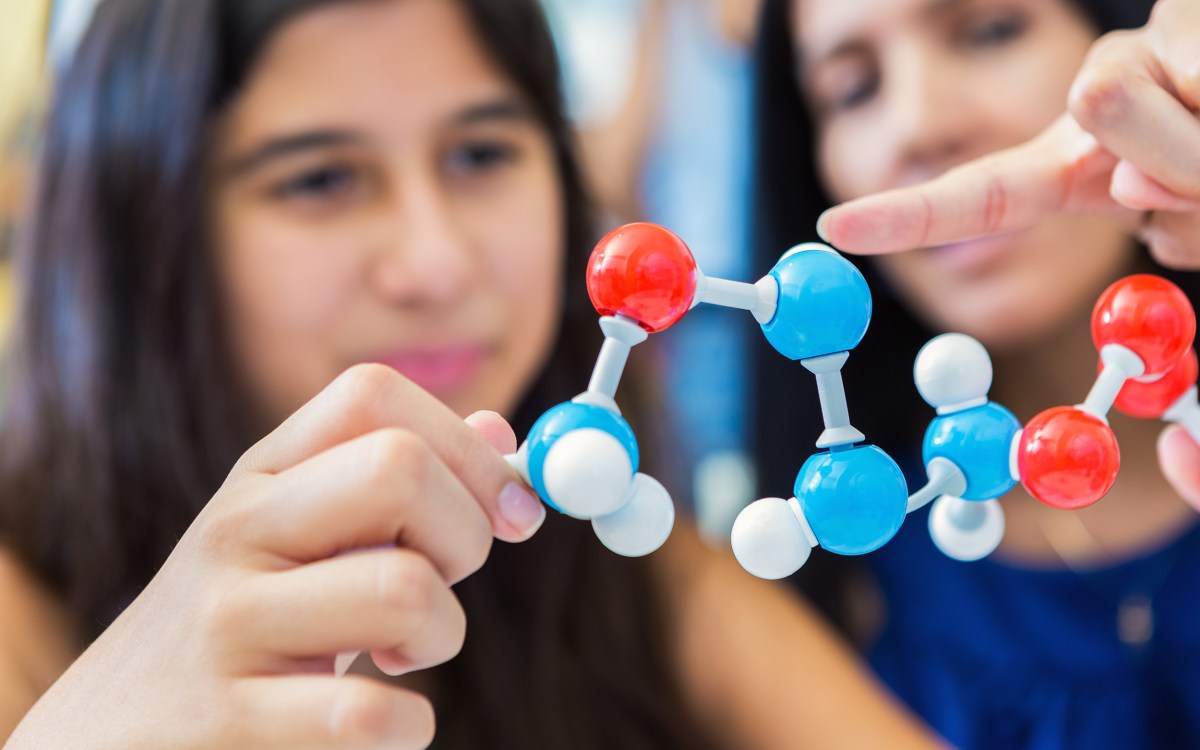
Research suggests encouragement toward humanities appears to be very influential for daughters
You want to be boss. You probably won’t be good at it.
Study pinpoints two measures that predict effective managers
Your kid can’t name three branches of government? He’s not alone.
Efforts launched to turn around plummeting student scores in U.S. history, civics, amid declining citizen engagement across nation
Good genes are nice, but joy is better
Harvard study, almost 80 years old, has proved that embracing community helps us live longer, and be happier
- Open access
- Published: 14 May 2020
Legal reflections on the case of genome-edited babies
- Shuang Liu 1
Global Health Research and Policy volume 5 , Article number: 24 ( 2020 ) Cite this article
54k Accesses
9 Citations
4 Altmetric
Metrics details
Human genome-editing is banned by guidelines, laws and regulations in most countries. However, the first criminal case on genome-edited babies was sentenced in China in 2019. In this commentary we discuss our legal reflections on this case. Genome-editing on healthy embryos of human may lead to irreversible mutations and serious consequences on the heredity of future generations, while its long-term safety is unpredictable. A full set of laws, regulations along with the guidelines should be formulated to penalize genome-editing behaviors and prevent similar negative events in the future. More effective and binding mechanisms should be constructed and implemented among different countries. A collaborative network should be strengthened for better global registry and surveillance of human genome-editing technologies and research.
Introduction
On December 30, 2019, a Chinese researcher, Jiankui He, was sentenced by Chinese local Court in Shenzhen City to 3 years of imprisonment with a fine of 3 million RMB Yuan for committing the crime of “Illegal Medical Practice”, and the other two defendants in the same case were also sentenced. One was sentenced to imprisonment of 2 years with a fine of 1 million RMB Yuan, another was sentenced to imprisonment of 1 year and 6 months (with probation of 2 years) with a fine of 0.5 million RMB Yuan [ 1 ]. The court concluded that each of the three defendants did not have a doctor’s practice license, and they applied the genome-editing technology (known as Clustered Regularly Interspaced Short Palindromic Repeats, CRISPR) to human assisted reproductive medicine, which caused the genetic changes of babies. In this case, the fathers are HIV positive and the mothers are HIV negative. Eggs were extracted from their body and the twin pregnancy gestated after the genome-edited embryos were transferred to their uterus. Genome-editing was undertaken to remove the CCR5 gene which allows the HIV to infect cells. The birth of babies represented a controversial leap in genome editing [ 2 ]. One day later when He announced the research of his team, more than 100 Chinese scientists and scholars signed a joint statement to denounce the trial. They reasserted that both the accuracy of the CRISPR and the potential off-target effects are controversial in the scientific community. Any attempt to directly transform human embryos and produce babies before rigorous tests poses tremendous risks. Such experiment is forbidden by the international biomedical community.
Genome-editing technology can bring great positive influence to the human being. It can be used to treat certain genome-related diseases [ 3 ]. However, it can also lead to some problems, such as how to (1) use it ethically and legally; (2) acquire the real consent from the experimental subjects; (3) how to penalize the genome-editing research for non-medical reasons, etc. Specific laws and regulations are important to resolve these problems because they are the last line of defense for social governance.
Current laws and regulations of genome-editing in different countries
Human genome-editing is largely forbidden by laws or guidelines even in countries permissive to human embryonic stem cell research [ 4 ]. Many countries have banned human genome-editing. Thirty nine countries were surveyed and categorized as “Ban based on legislation” (25 countries), “Ban based on guidelines” (4), “Ambiguous” (9) and “Restrictive” (1). China, India, Ireland, and Japan forbid genome-editing based on guidelines which are less enforceable than laws and are subject to amendment [ 3 ]. In the USA, Human genome-editing is not banned, but a moratorium is imposed under vigilance of the Food and Drug Administration (FDA) and the guidelines of the National Institutes of Health (NIH). Any clinical trial proposals for germline alterations will be rejected by the Recombinant DNA Advisory Committee (RAC) of the NIH. Clinical studies are regulated by FDA [ 5 ]. In the UK, the legislation of medical use of mitochondrial replacement is likely to lead to legal permission for the modification of germline nuclear genome that can be readily changed by genome-editing technology [ 6 ].
Although genome-editing is banned in many countries, necessary and practical laws, regulations and guidelines should be developed, and appropriate penalty should be applied in proportion to the crime. Preventive measures should also be stipulated in a specific law. Early embryo genome-editing for fertility purposes violates the ethical principles provided in the “Declaration of Helsinki-Ethical Principles for Medical Research Involving Human Subjects” (hereafter referred to as “Declaration of Helsinki”), which has been widely accepted by the international community. In He’s case, early human embryos were edited artificially. Consequently, the genome-editing babies not only face the risk of uncertainty, but also are deprived of the right to an open future. The Article 9 of “Declaration of Helsinki” states that the responsibility for the protection of research subjects must always rest with the physicians or other health care professionals and never with the research subjects, even though they have been given consent. He and his team violated the provisions of both Article 9 of Declaration of Helsinki and the Chinese criminal law, and their misconducts should be punished.
The sentence of genome-editing babies in China
Current Chinese laws are insufficient to deal with new challenges posed by new expertise and technologies. The regulations prohibit the development of genome-editing embryos beyond 14 days. The Chinese Guideline on Human Assisted Reproductive Technologies stipulates that the use of human egg plasma and nuclear transfer technology for the purpose of reproduction, and manipulation of the genomes in human gametes, zygotes or embryos for the purpose of reproduction are prohibited. In He’s case, it is unknown whether his team had acquired the true informed consent and they were convicted the crime of “Illegal Medical Practice”. The local court concluded that their behaviors deliberately violated the National Regulations on Scientific Research and Medical Management, crossed an ethical bottom line, and rashly applied genome-editing technology. Genome-editing on embryos with existing technologies and methods is not the only way to prevent mother-to-child transmission of AIDS [ 7 ]. Besides, the experimental procedure was unclear and nontransparent, but the consequence is full of risks. However, according to the Chinese Criminal Law, three-year imprisonment and below is regarded as misdemeanor. In contrast, the punishment of similar behavior could be 10 years of imprisonment in the UK and 20 years in France at maximum [ 8 ]. It is obvious that He and his team were eager for quick success, and their misconducts were irresponsible and dangerous. Moreover, their genome-editing behavior may cause irreversible damage to the entire human genome chain. Therefore, He’s case is very typical to warn other scientists not to commit similar misconducts. Fortunately, on May 28, 2019, the Chinese government promulgated the Regulation of the People’s Republic of China on the Administration of Human Genetic Resources, which aims to protect public health, national security, and public interest through effective protection and rational use of China’s human genetic resources.
Human genome-editing technology is a two-sided sword. The advantage of its benefit can be explored. However, further legislation is required to punish misconducts and avoid potential risks.
A specific crime and more severe penalty should be formulated in the Chinese Criminal Law. Civil responsibility should be assumed if a medical institution or a person in charge do not truthfully and fully informed patients of potential risks.
Better governance is needed. According to the Administrative Penalty Law, local government and other administrative agencies should assume responsibilities if they fail to carry out their duties in ethical review, supervision and management.
More effective and binding mechanisms to constrain the use of genome-editing technology should be developed. More specific guidelines and preventive measures should be formulated in consistent with the international regulations.
A collaborative network should be strengthened for better global registry and surveillance of human genome-editing technologies and research, led by the World Health Organization (WHO) Expert Advisory Committee on Developing Global Standards for Governance and Oversight of Human Genome Editing.
Availability of data and materials
Not applicable.
Abbreviations
Clustered Regularly Interspaced Short Palindromic Repeats.
Food and Drug Administration
National Institutes of Health
Recombinant DNA Advisory Committee
World Health Organization
Song D. Gene-editing scientist jailed in China. https://finance.sina.com.cn/china/gncj/2019-12-30/doc-iihnzhfz9255859.shtml?cre=tianyi&m od=pcpager_fin&loc=33&r=9&rfunc=100&tj=none&tr=9.Accessed 22 Jan 2020.
Cyranoski D, Ledford H. International outcry over genome-edited baby claim. Nature. 2018;563:–607.
Motoko A, Tetsuya I. International regulatory landscape and integration of corrective genome editing into in vitro fertilization. Reprod Biol Endocrinol. 2014;12:108.
Article Google Scholar
Tetsuya I. Potential impact of human mitochondrial replacement on global policy regarding germline gene modification. Reprod Biomed Online. 2014;29(2):150–5.
NIH. NIH Guidelines for Research Involving Recombinant or Synthetic Nucleic Acid Molecules. 2013.
Google Scholar
Wong CC, Johnson MH. Therapy for mitochondrial genetic disease: are we at the thin end of the wedge? Reprod Biomed Online. 2014;29(2):147–9.
World Health Organization. Global guidance on criteria and process for validation: elimination of mother-to-child transmission of HIV and syphilis. Geneva: World Health Organization; 2014.
Liu LJ. Three legal problems of gene-editing babies. http://www.jcrb.com/FYFZ/zxbd/201901/t20190114_1952628.html . Accessed 3 Mar 2020.
Download references
Acknowledgements
The author would like to thank the comments and advices of reviewers in improving the quality of the article.
This work is supported by a project Research on the Recent Expansion of Chinese Criminal Law and Its Reasonable Limits funded by National Social Science Fund Project of China (grant No.: 16BFX056).
Author information
Authors and affiliations.
European Law Research Center, Henan University, No.85, Minglun Road, Sunhe District, Kaifeng, 475001, China
You can also search for this author in PubMed Google Scholar
Contributions
Liu S designed and wrote the article. The author read and approved the final manuscript.
Corresponding author
Correspondence to Shuang Liu .
Ethics declarations
Ethics approval and consent to participate, consent for publication.
The author agreed the publication of this manuscript.
Competing interests
The author declares that she has no competing interests.
Rights and permissions
Open Access This article is licensed under a Creative Commons Attribution 4.0 International License, which permits use, sharing, adaptation, distribution and reproduction in any medium or format, as long as you give appropriate credit to the original author(s) and the source, provide a link to the Creative Commons licence, and indicate if changes were made. The images or other third party material in this article are included in the article's Creative Commons licence, unless indicated otherwise in a credit line to the material. If material is not included in the article's Creative Commons licence and your intended use is not permitted by statutory regulation or exceeds the permitted use, you will need to obtain permission directly from the copyright holder. To view a copy of this licence, visit http://creativecommons.org/licenses/by/4.0/ .
Reprints and permissions
About this article
Cite this article.
Liu, S. Legal reflections on the case of genome-edited babies. glob health res policy 5 , 24 (2020). https://doi.org/10.1186/s41256-020-00153-4
Download citation
Received : 20 February 2020
Accepted : 03 May 2020
Published : 14 May 2020
DOI : https://doi.org/10.1186/s41256-020-00153-4
Share this article
Anyone you share the following link with will be able to read this content:
Sorry, a shareable link is not currently available for this article.
Provided by the Springer Nature SharedIt content-sharing initiative
- Genome-edited babies
- Global registry
Global Health Research and Policy
ISSN: 2397-0642
- Submission enquiries: Access here and click Contact Us
- General enquiries: [email protected]
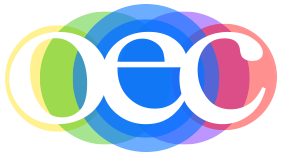
Site Search
- How to Search
- Advisory Group
- Editorial Board
- OEC Fellows
- History and Funding
- Using OEC Materials
- Collections
- Research Ethics Resources
- Ethics Projects
- Communities of Practice
- Get Involved
- Submit Content
- Open Access Membership
- Become a Partner
- Advanced Search
- Webinar Series
Case: Genome Editing & the Ethics of CRISPR-Cas9
This historical case uses the April 2015 publication on editing the genomes of non-viable human embryos by Junjiu Huang et. al. to explore the ethical issues surrounding the development of gene editing technologies and application to humans.
In April 2015, scientists in China published a paper in an online journal, Proteins & Cells , about experiments editing the genomes of non-viable human embryos (Liang et al. 2015). The research team, led by Junjiu Huang, used an engineered enzyme complex, called CRISPR-Cas9, to target and edit the HBB gene that codes for human β -globin protein. Defects in that gene can lead to β -thalassaemia, a heritable blood disorder that can be fatal.
In 2012, scientists Jennifer Doudna and Emmanelle Charpentier developed the CRISPR-Cas9 bioengineered complex that was used by the researchers in China. The technology has been used in previous research on animal and adult human cells. The technology allows researchers to target a specific gene by binding and splicing the DNA at specific locations, and replacing or repairing the segment by inserting other molecules (Cyranoski & Reardon 2015).
In their research, Huang and his team used non-viable, single-cell human embryos, which they obtained from a fertility clinic. The embryos possessed an extra set of chromosomes because they had been fertilized by two sperm and thus could not develop beyond the first stages of development. Huang and colleagues' aim was to test whether the technology could reliably target defective genes and replace these genes with repaired sequences. Their results showed that only a small fraction of the 86 embryos used in the study had the replaced genetic material at the targeted gene. The researchers also found that there were many "off-target" mutations that might have been introduced in the genome as a by-product of the technological intervention (Cyranoski & Reardon 2015). These results led the researchers to conclude that clinical applications of the technology to human embryos were still premature.
The authors of the paper also claimed that the prestigious journals, Science and Nature , rejected their paper because of ethical objections to their research on human embryos, and specifically, because of ethical objections to any kind of germ line genetic modification. The editors at the journal, Proteins & Cells , justified publishing the paper by claiming that they verified the researchers' institutional approval and the consent forms from the embryo donors, and confirmed that the study was compliant with Chinese laws and the Declaration of Helsinki's set of ethical principles on human experimentation (Cressey & Cyranoski 2015).
Were the editors at Science and Nature right to decline to publish the research paper? Should the editors at Protein & Cells have refrained from publishing the paper? Should the individual scientists have conducted and reported their research at all? Did they violate any moral duties or obligations? Given the paper is now published, what should members of the scientific community do?
Discussion Questions:
- Leading scientists called for a moratorium on research on human embryos using genome-editing technologies, such as CRISPR. What sorts of ethical concerns does a moratorium address? What are some goals that could be achieved? What do you think are the likely outcomes or consequences of a moratorium and of the technology?
- Why did the editors at Science and Nature decided not to publish the paper? Were the editors at Protein & Cells too hasty in publishing the research on embryos? How should academic journals deal with potentially controversial research? What are their moral and social responsibilities?
- Should non-scientist members of the public be included in the decision-making process about whether or not, and to what extent, research with genome-editing technologies should be restricted? What kinds of concerns might be overlooked if the decision-making process included only scientists? What sorts of insights may non-scientist members of the public bring to the discussion?
- What should be the social and ethical responsibilities of the individual scientists involved in research with genome-editing technology? How can they best fulfill those responsibilities?
Bibliography
Baltimore, B. D., Paul Berg, Michael Botchan, Dana Carroll, R. Alta Charo, George Church, Jacob E. Corn, et al. "A prudent path forward for genomic engineering and germline gene modification." Science 348, no. 6230 (2015): 36-38. doi: 10.1126/science.aab1028
Cressey, David, and David Cyranoski. "Human-embryo editing poses challenges for journals." Nature . April 25, 2015. Accessed December 10, 2015. http://www.nature.com/news/human-embryo-editing-poses-challenges-for-journals-1.17429
Cyranoski, David, and Sara Reardon. "Chinese scientists genetically modify human embryos." Nature. April 22, 2015. Accessed December 10, 2015. http://www.nature.com/news/chinese-scientists-genetically-modify-human-embryos-1.17378
Doudna, Jennifer A., and Emmanuelle Charpentier. "The new frontier of genome engineering with CRISPR-Cas9." Science 346, no. 6213 (2014): 1258096. doi: 10.1126/science.1258096
Jasanoff, Sheila, J. Benjamin Hurlbut, and Krishanu Saha. "Human genetic engineering demands more than a moratorium." The Guardian . April 7, 2015. Accessed December 10, 2015. http://www.theguardian.com/science/political-science/2015/apr/07/human-genetic-engineering-demands-more-than-a-moratorium
Lanphier, Edward, Fyodor Urnov, Sarah Ehlen Haecker, Michael Werner, and Joanna Smolenski. "Don't edit the human germ line." Nature 519, no. 7544 (2015): 410. Accessed December 10, 2015. http://www.nature.com/news/don-t-edit-the-human-germ-line-1.17111
Ledford, Heidi. "CRISPR, the disruptor." Nature 522. June 8, 2015. Accessed December 10, 2015. http://www.nature.com/news/crispr-the-disruptor-1.17673
Liang, Puping, Yanwen Xu, Xiya Zhang, Chenhui Ding, Rui Huang, Zhen Zhang, Jie Lv et al. "CRISPR/Cas9-mediated gene editing in human tripronuclear zygotes." Protein & cell (2015): 1-10. doi: 10.1007/s13238-015-0153-5
Reardon, Sara. "Ethics of Embryo Editing Paper Divides Scientists." Nature . April 24, 2015a. Accessed December 10, 2015. http://www.nature.com/news/ethics-of-embryo-editing-paper-divides-scientists-1.17410
Reardon, Sara. "US science academies take on human-genome editing." Nature . May 18, 2015b. Accessed December 10, 2015. http://www.nature.com/news/us-science-academies-take-on-human-genome-editing-1.17581
Sarewitz, Daniel. "CRISPR: Science can't solve it." Nature 522. June 23, 2015. Accessed December 10, 2015. http://www.nature.com/news/crispr-science-can-t-solve-it-1.17806
Vogel, Gretchen. "Embryo engineering alarm." Science 347, no. 6228 (2015): 1301-1301. doi: 10.1126/science.347.6228.1301
Embryo-Editing: The Ethics of CRISPR on Flipboard: https://flipboard.com/@naturenewsteam/embryo-editing%3A-the-ethics-of-crispr-27j1164kz
The National Academies of Sciences, Engineering, and Medicine - On Human Gene Editing: International Summit Statement : http://www8.nationalacademies.org/onpinews/newsitem.aspx?RecordID=12032015a
The author wishes to acknowledge the contributions of Karin Ellison, OEC - Life and Environmental Sciences Editor, and Joseph Herkert, OEC Engineering co-Editor. They provided valuable input in selecting topics and crafting the resources.
The publication of Huang and colleagues’ research caused a stir in the scientific community and generated many editorials and opinion pieces in scientific publications warning about the ethical issues that must be addressed before this research is pursued any further.
Scientists were quick to call for a moratorium on all genome editing of human embryos, and invoked similarities to the technological innovation that led to recombinant DNA in the 1970s and the meeting at Asilomar in 1975, where molecular biologists met to discuss and set guidelines to ensure that genetic research would develop in a safe and ethical manner (Vogel 2015).
However, many are critical of the comparisons with the Asilomar meeting and the attempt to use that conference as a model on which to build bioethical guidelines for future research with genome editing technologies (Jasanoff et al. 2015). Critics claim that the 1975 Asilomar conference was not an inclusive meeting because many of the stakeholders were not invited, such as ethicists, politicians, religious groups, and representatives of human-rights organizations or patient-interest groups (Reardon 2015b). Because of the lack of representation from non-scientists in the discussions, critics claim that Asilomar was merely an effort by scientists to resist government restrictions and promote public trust in the idea that scientists are able to regulate themselves (Reardon 2015b).
In response to calls for a moratorium, the US National Academy of Sciences (NAS) and the National Academy of Medicine (NAM) have launched an initiative to develop new guidelines to address the use of technology which makes germ line genetic modification possible, and called for members of the scientific community to attend an international summit on the topic set in December 2015 (Reardon 2015b).
The International Summit on Human Gene Editing held in Washington, D.C., in December 2015, was hosted by the National Academy of Sciences, the National Academy of Medicine, the Chinese Academy of Sciences, and the U.K.'s Royal Society. Members of the Summit’s organizing committee submitted a public statement shortly after the meeting, outlining four recommendations. First, basic and preclinical research on gene-editing technologies is needed and should proceed. Second, clinical use of the technologies on somatic cells should be explored. Third, it is irresponsible to pursue clinical applications of gene-editing technologies on germline cells at this time. And, fourth, there is a need for ongoing discussions regarding the clinical use of germline gene editing, so the national academies should create a forum to allow for discussions which are inclusive and which engage with a variety of perspectives and expertise.
Some science policy experts have argued that the complexity of the issues surrounding germ line genetic modification cannot be adequately addressed from a scientific perspective. For example, Daniel Sarewitz, co-director of Arizona State University’s Consortium for Science, Policy, and Outcomes, argues:
The idea that the risks, benefits and ethical challenges of these emerging technologies are something to be decided by experts is wrong-headed, futile and self-defeating. It misunderstands the role of science in public discussions about technological risk. It seriously underestimates the democratic sources of science's vitality and the capacities of democratic deliberation. And it will further delegitimize and politicize science in modern societies (Sarewitz 2015).
Sarewitz’s comment signifies the importance of a democratic deliberative process when identifying and addressing ethical issues about emerging technologies, as well as developing guidelines that will help to decide how these technologies will be further developed and used. In this particular case, there is worry that germ line genetic modification on human embryos to replace defective genes may lead to a slippery slope to eugenics, or attempts to create perfect designer babies.
Lastly, the decision by Science and Nature to decline to publish the research paper because of undisclosed ethical objections raised further ethical issues about the dissemination of scientific research within a global context. The managing editor of Protein & Cells , Xiaoxue Zhang, has claimed that their editorial board was not blind to the potential ethical objections to the research, but decided to publish the article as a way to “sound an alarm” to begin discussions about the future direction of genome editing technologies (Cressey & Cyranoski 2015). Whether these discussions should come before or after the scientific research is conducted or published raises important questions about how best to regulate innovative scientific research with uncertain outcomes or potential dual-use applications.
Related Resources
Submit Content to the OEC Donate
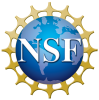
This material is based upon work supported by the National Science Foundation under Award No. 2055332. Any opinions, findings, and conclusions or recommendations expressed in this material are those of the author(s) and do not necessarily reflect the views of the National Science Foundation.

Want to create or adapt books like this? Learn more about how Pressbooks supports open publishing practices.
5.1 Case Study: Genes and Inheritance
Created by: CK-12/Adapted by Christine Miller
Case Study: Cancer in the Family
People tend to carry similar traits to their biological parents, as illustrated by the family tree. Beyond just appearance, you can also inherit traits from your parents that you can’t see.
Rebecca becomes very aware of this fact when she visits her new doctor for a physical exam. Her doctor asks several questions about her family medical history, including whether Rebecca has or had relatives with cancer. Rebecca tells her that her grandmother, aunt, and uncle — who have all passed away — had cancer. They all had breast cancer, including her uncle, and her aunt also had ovarian cancer. Her doctor asks how old they were when they were diagnosed with cancer. Rebecca is not sure exactly, but she knows that her grandmother was fairly young at the time, probably in her forties.
Rebecca’s doctor explains that while the vast majority of cancers are not due to inherited factors, a cluster of cancers within a family may indicate that there are mutations in certain genes that increase the risk of getting certain types of cancer, particularly breast and ovarian cancer. Some signs that cancers may be due to these genetic factors are present in Rebecca’s family, such as cancer with an early age of onset (e.g., breast cancer before age 50), breast cancer in men, and breast cancer and ovarian cancer within the same person or family.
Based on her family medical history, Rebecca’s doctor recommends that she see a genetic counselor, because these professionals can help determine whether the high incidence of cancers in her family could be due to inherited mutations in their genes. If so, they can test Rebecca to find out whether she has the particular variations of these genes that would increase her risk of getting cancer.
When Rebecca sees the genetic counselor, he asks how her grandmother, aunt, and uncle with cancer are related to her. She says that these relatives are all on her mother’s side — they are her mother’s mother and siblings. The genetic counselor records this information in the form of a specific type of family tree, called a pedigree, indicating which relatives had which type of cancer, and how they are related to each other and to Rebecca.
He also asks her ethnicity. Rebecca says that her family on both sides are Ashkenazi Jews (Jews whose ancestors came from central and eastern Europe). “But what does that have to do with anything?” she asks. The counselor tells Rebecca that mutations in two tumor-suppressor genes called BRCA1 and BRCA2 , located on chromosome 17 and 13, respectively, are particularly prevalent in people of Ashkenazi Jewish descent and greatly increase the risk of getting cancer. About one in 40 Ashkenazi Jewish people have one of these mutations, compared to about one in 800 in the general population. Her ethnicity, along with the types of cancer, age of onset, and the specific relationships between her family members who had cancer, indicate to the counselor that she is a good candidate for genetic testing for the presence of these mutations.
Rebecca says that her 72-year-old mother never had cancer, nor had many other relatives on that side of the family. How could the cancers be genetic? The genetic counselor explains that the mutations in the BRCA1 and BRCA2 genes, while dominant, are not inherited by everyone in a family. Also, even people with mutations in these genes do not necessarily get cancer — the mutations simply increase their risk of getting cancer. For instance, 55 to 65 per cent of women with a harmful mutation in the BRCA1 gene will get breast cancer before age 70, compared to 12 per cent of women in the general population who will get breast cancer sometime over the course of their lives.
Rebecca is not sure she wants to know whether she has a higher risk of cancer. The genetic counselor understands her apprehension, but explains that if she knows that she has harmful mutations in either of these genes, her doctor will screen her for cancer more often and at earlier ages. Therefore, any cancers she may develop are likely to be caught earlier when they are often much more treatable. Rebecca decides to go through with the testing, which involves taking a blood sample, and nervously waits for her results.
Chapter Overview: Genetics
At the end of this chapter, you will find out Rebecca’s test results. By then, you will have learned how traits are inherited from parents to offspring through genes, and how mutations in genes such as BRCA1 and BRCA2 can be passed down and cause disease. Specifically, you will learn about:
- The structure of DNA.
- How DNA replication occurs.
- How DNA was found to be the inherited genetic material.
- How genes and their different alleles are located on chromosomes.
- The 23 pairs of human chromosomes, which include autosomal and sex chromosomes.
- How genes code for proteins using codons made of the sequence of nitrogen bases within RNA and DNA.
- The central dogma of molecular biology, which describes how DNA is transcribed into RNA, and then translated into proteins.
- The structure, functions, and possible evolutionary history of RNA.
- How proteins are synthesized through the transcription of RNA from DNA and the translation of protein from RNA, including how RNA and proteins can be modified, and the roles of the different types of RNA.
- What mutations are, what causes them, different specific types of mutations, and the importance of mutations in evolution and to human health.
- How the expression of genes into proteins is regulated and why problems in this process can cause diseases, such as cancer.
- How Gregor Mendel discovered the laws of inheritance for certain types of traits.
- The science of heredity, known as genetics, and the relationship between genes and traits.
- How gametes, such as eggs and sperm, are produced through meiosis.
- How sexual reproduction works on the cellular level and how it increases genetic variation.
- Simple Mendelian and more complex non-Mendelian inheritance of some human traits.
- Human genetic disorders, such as Down syndrome, hemophilia A, and disorders involving sex chromosomes.
- How biotechnology — which is the use of technology to alter the genetic makeup of organisms — is used in medicine and agriculture, how it works, and some of the ethical issues it may raise.
- The human genome, how it was sequenced, and how it is contributing to discoveries in science and medicine.
As you read this chapter, keep Rebecca’s situation in mind and think about the following questions:
- BCRA1 and BCRA2 are also called Breast cancer type 1 and 2 susceptibility proteins. What do the BRCA1 and BRCA2 genes normally do? How can they cause cancer?
- Are BRCA1 and BRCA2 linked genes? Are they on autosomal or sex chromosomes?
- After learning more about pedigrees, draw the pedigree for cancer in Rebecca’s family. Use the pedigree to help you think about why it is possible that her mother does not have one of the BRCA gene mutations, even if her grandmother, aunt, and uncle did have it.
- Why do you think certain gene mutations are prevalent in certain ethnic groups?
Attributions
Figure 5.1.1
Family Tree [all individual face images] from Clker.com used and adapted by Christine Miller under a CC0 1.0 public domain dedication license (https://creativecommons.org/publicdomain/zero/1.0/).
Figure 5.1.2
Rebecca by Kyle Broad on Unsplash is used under the Unsplash License (https://unsplash.com/license).
Wikipedia contributors. (2020, June 27). Ashkenazi Jews. In Wikipedia. https://en.wikipedia.org/w/index.php?title=Ashkenazi_Jews&oldid=964691647
Wikipedia contributors. (2020, June 22). BRCA1. In Wikipedia . https://en.wikipedia.org/w/index.php?title=BRCA1&oldid=963868423
Wikipedia contributors. (2020, May 25). BRCA2. In Wikipedia. https://en.wikipedia.org/w/index.php?title=BRCA2&oldid=958722957
Human Biology Copyright © 2020 by Christine Miller is licensed under a Creative Commons Attribution-NonCommercial 4.0 International License , except where otherwise noted.
Share This Book
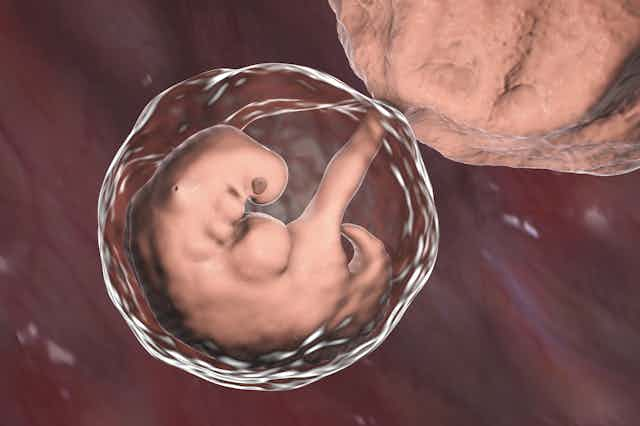
China’s failed gene-edited baby experiment proves we’re not ready for human embryo modification
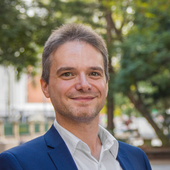
Senior Lecturer, Queensland University of Technology
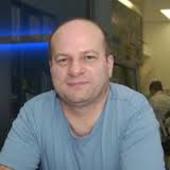
Geneticist and Group Leader, The John Curtin School of Medical Research, Australian National University
Disclosure statement
Dimitri Perrin has received funding from the Australian Research Council (ARC), the Australian-French Association for Innovation and Research (AFRAN), and the Advance Queensland programme.
Gaetan Burgio receives funding from the National Health and Medical Research Council (NHMRC), the Australian Research Council (ARC), the National Collaborative Research Infrastructure Strategy (NCRIS) via the Australian Phenomics Network (APN) ,Universities Australia and the Natural Science Foundation in China (NSFC).
Queensland University of Technology and Australian National University provide funding as members of The Conversation AU.
View all partners
- Bahasa Indonesia
More than a year ago, the world was shocked by Chinese biophysicist He Jiankui’s attempt to use CRISPR technology to modify human embryos and make them resistant to HIV, which led to the birth of twins Lulu and Nana.
Now, crucial details have been revealed in a recent release of excerpts from the study, which have triggered a series of concerns about how Lulu and Nana’s genome was modified.
How CRISPR works
CRISPR is a technique that allows scientists to make precise edits to any DNA by altering its sequence.
When using CRISPR, you may be trying to “knock out” a gene by rendering it inactive, or trying to achieve specific modifications, such as introducing or removing a desired piece of DNA.
Read more: What is CRISPR gene editing, and how does it work?
Gene editing with the CRISPR system relies on an association of two molecules. One is a protein, called Cas9, that is responsible for “cutting” the DNA. The other molecule is a short RNA (ribonucleic acid) molecule which works as a “guide” that brings Cas9 to the position where it is supposed to cut.
The system also needs help from the cells being edited. DNA damage is frequent, so cells regularly have to repair the DNA lesions. The associated repair mechanisms are what introduce the deletions, insertions or modifications when performing gene editing.
How the genomes of Lulu and Nana were modified
He Jiankui and his colleagues were targeting a gene called CCR5, which is necessary for the HIV virus to enter into white blood cells ( lymphocytes ) and infect our body.
One variant of CCR5, called CCR5 Δ32, is missing a particular string of 32 “letters” of DNA code. This variant naturally occurs in the human population, and results in a high level of resistance to the most common type of HIV virus.
The team wanted to recreate this mutation using CRISPR on human embryos, in a bid to render them resistant to HIV infection. But this did not go as planned, and there are several ways they may have failed.
First, despite claiming in the abstract of their unpublished article that they reproduced the human CCR5 mutation, in reality the team tried to modify CCR5 close to the Δ32 mutation.
As a result, they generated different mutations, of which the effects are unknown. It may or may not confer HIV resistance, and may or may not have other consequences.
Worryingly, they did not test any of this, and went ahead with implanting the embryos. This is unjustifiable.
The mosaic effect
A second source of errors could have been that the editing was not perfectly efficient. This means that not all cells in the embryos were necessarily edited.
When an organism has a mixture of edited and unedited cells, it is called a “mosaic”. While the available data are still limited, it seems that both Lulu and Nana are mosaic.
This makes it even less likely that the gene-edited babies would be resistant to HIV infection. The risk of mosaicism should have been another reason not to implant the embryos.
Read more: 'Designer' babies won't be common anytime soon – despite recent CRISPR twins
Moreover, editing can have unintended impacts elsewhere in the genome.
When designing a CRISPR experiment, you choose the “guide” RNA so that its sequence is unique to the gene you are targeting. However, “off-target” cuts can still happen elsewhere in the genome, at places that have a similar sequence.
He Jiankui and his team tested cells from the edited embryos, and reported only one off-target modification. However, that testing required sampling the cells, which were therefore no longer part of the embryos - which continued developing.
Thus, the remaining cells in the embryos had not been tested, and may have had different off-target modifications.
This is not the team’s fault, as there will always be limitations in detecting off-target and mosaicism, and we can only get a partial picture.
However, that partial picture should have made them pause.
A bad idea to begin
Above, we have described several risks associated with the modifications made on the embryos, which could be passed on to future generations.
Embryo editing is only ethically justifiable in cases where the benefits clearly outweigh the risks.
Technical issues aside, the researchers did not even address an unmet medical need.
While the twins’ father was HIV-positive, there is already a well-established way to prevent an HIV-positive father from infecting embryos. This “ sperm washing ” method was actually used by the team.
The only benefit of the attempted gene modification, if proven, would have been a reduced risk of HIV infection for the twins later in life.
But there are safer existing ways to control the risk of infection, such as condoms and mandatory testing of blood donations.
Implications for gene editing as a field
Gene editing has endless applications. It can be used to make plants such as the Cavendish banana more resistant to devastating diseases . It can play an important role in the adaptation to climate change.
In health, we are already seeing promising results with the editing of somatic cells (that is, non-heritable modifications of the patient’s own cells) in beta thalassemia and sickle cell disease.
However, we are just not ready for human embryo editing. Our techniques are not mature enough, and no case has been made for a widespread need that other techniques, such as preimplantation genetic testing, could not address.
Read more: Experts call for halt to CRISPR editing that allows gene changes to pass on to children
There is also much work still needed on governance. There have been individual calls for a moratorium on embryo editing, and expert panels from the World Health Organisation to UNESCO .
Yet, no consensus has emerged.
It is important these discussions move in unison to a second phase, where other stakeholders, such as patient groups, are more broadly consulted (and informed). Engagement with the public is also crucial.
Correction: this article originally described RNA (ribonucleic acid) as a protein, rather than a molecule.
- CRISPR/Cas9
- Gene editing
- Human embryos
- Gene editing summit
- Editing human embryos
- embryo editing
- Global perspectives
- Gene mutation
- CRISPR edited baby
- Gene edited babies

Director of STEM

Community member - Training Delivery and Development Committee (Volunteer part-time)

Chief Executive Officer

Finance Business Partner

Head of Evidence to Action

An official website of the United States government
The .gov means it’s official. Federal government websites often end in .gov or .mil. Before sharing sensitive information, make sure you’re on a federal government site.
The site is secure. The https:// ensures that you are connecting to the official website and that any information you provide is encrypted and transmitted securely.
- Publications
- Account settings
Preview improvements coming to the PMC website in October 2024. Learn More or Try it out now .
- Advanced Search
- Journal List
- Eur J Hum Genet
- v.26(1); 2018 Jan
One small edit for humans, one giant edit for humankind? Points and questions to consider for a responsible way forward for gene editing in humans
Heidi c. howard.
1 Centre for Research Ethics and Bioethics, Uppsala University, Uppsala, Sweden
Carla G. van El
2 Department of Clinical Genetics, Section Community Genetics and EMGO Institute for Health and Care Research, VU University Medical Center, Amsterdam, The Netherlands
Francesca Forzano
3 Department of Clinical Genetics, Great Ormond Street Hospital, London, UK
Dragica Radojkovic
4 Laboratory for Molecular Genetics, Institute of Molecular Genetics and Genetic Engineering, University of Belgrade, Belgrade, Serbia
Emmanuelle Rial-Sebbag
5 UMR 1027, Inserm, Faculté de médecine Université Toulouse 3, Paul Sabatier, Toulouse France
Guido de Wert
7 Department of Health, Ethics and Society, Research Schools CAPHRI and GROW, Maastricht University, Maastricht, The Netherlands
Pascal Borry
6 Centre for Biomedical Ethics and Law, Department of Public Health and Primary Care, Leuven Institute for Genomics and Society, KU Leuven, Kapucijnenvoer 35 Box 7001, 3000 Leuven, Belgium
Martina C. Cornel
Gene editing, which allows for specific location(s) in the genome to be targeted and altered by deleting, adding or substituting nucleotides, is currently the subject of important academic and policy discussions. With the advent of efficient tools, such as CRISPR-Cas9, the plausibility of using gene editing safely in humans for either somatic or germ line gene editing is being considered seriously. Beyond safety issues, somatic gene editing in humans does raise ethical, legal and social issues (ELSI), however, it is suggested to be less challenging to existing ethical and legal frameworks; indeed somatic gene editing is already applied in (pre-) clinical trials. In contrast, the notion of altering the germ line or embryo such that alterations could be heritable in humans raises a large number of ELSI; it is currently debated whether it should even be allowed in the context of basic research. Even greater ELSI debates address the potential use of germ line or embryo gene editing for clinical purposes, which, at the moment is not being conducted and is prohibited in several jurisdictions. In the context of these ongoing debates surrounding gene editing, we present herein guidance to further discussion and investigation by highlighting three crucial areas that merit the most attention, time and resources at this stage in the responsible development and use of gene editing technologies: (1) conducting careful scientific research and disseminating results to build a solid evidence base; (2) conducting ethical, legal and social issues research; and (3) conducting meaningful stakeholder engagement, education and dialogue.
Introduction
Gene editing, which allows for specific location(s) in the genome to be targeted and changed by deleting, adding or substituting nucleotides, is currently the subject of much academic, industry and policy discussions. While not new per se, gene editing has become a particularly salient topic primarily due to a relatively novel tool called CRISPR-Cas9. This specific tool distinguishes itself from its counterparts, (e.g., zinc-finger nucleases and TAL effector nucleases (TALENs)) due to a mixture of increased efficiency (number of sites altered), specificity (at the exact location targeted), ease of use and accessibility for researchers (e.g., commercially available kits), as well as a relatively affordable price [ 1 ]. These attributes make CRISPR-Cas9 an extremely useful and powerful tool that can (and has) been used in research in order to alter the genes in cells from a large range of different organisms, including plants, non-human animals and microorganisms, as well as in human cells [ 2 ]. Ultimately, CRISPR-Cas9 is becoming increasingly available to a larger number of scientists, who have used it, or intend to use it for a myriad of reasons in many different research domains. When such powerful and potentially disruptive technologies or tools (begin to) show a tendency to become widely used, it is common for debate and discussion to erupt. Germane to this debate is the fact that with the advent of CRISPR-Cas9 and other similar tools (e.g., CRISPR Cpf1), the possibility of using the technique of gene editing in a potentially safe and effective manner in humans—whether for somatic or germ line/heritable 1 gene editing—has become feasible in the near to medium future.
With some clinical trials underway, somatic genetic editing for therapeutic purposes is certainly much closer to being offered in the clinic. For example, several clinical trials on HIV are ongoing [ 3 , 4 ]; in 2015 an infant with leukaemia was treated with modified immunes cells (using TALENs) from a healthy donor [ 5 ]. Moreover, in the autumn of 2016, a Chinese group became 'the first to inject a person with cells that contain genes edited using the CRISPR-Cas9 technique' within the context of a clinical trial for aggressive lung cancer [ 6 ]. With such tools, gene editing is being touted as a feasible approach to treat or even cure certain single-gene diseases such as beta-thalassaemia and sickle-cell disease through somatic gene editing [ 3 ].
Beyond somatic cell gene editing, there is also discussion that through the manipulation of germ line cells or embryos, gene editing could be used to trans-generationally 'correct' or avoid single-gene disorders entirely. Notably, (ethical) concerns about heritable gene editing in humans were heightened when in April 2015, a group at Sun Yat-sen University in Guangzhou, China, led by Dr. Junjiu Huang reported they had successfully used gene editing in human embryos [ 7 ]. They used CRISPR-Cas9 to modify the beta-globin gene in non-viable (triplonuclear) spare embryos from in vitro fertility treatments. The authors concluded that while the experiments were successful overall, it is difficult to predict all the intended and unintended outcomes of gene editing in embryos (e.g., mosaicism, off-target events) and that 'clinical applications of the CRISPR-Cas9 system may be premature at this stage' [ 7 ]. Partly in anticipation/response to these experiments and to the increasing use of CRISPR-Cas9 in many different areas, a number of articles were published [ 2 , 8 – 14 ] and meetings were organized [ 9 , 10 , 15 – 17 ] in order to further discuss the scientific, ethical, legal, policy and social issues of gene editing, particularly regarding heritable human gene editing and the responsible way forward.
Internationally, some first position papers on human gene editing were published in 2015 and 2016. Interestingly, these different recommendations and statements do not entirely concur with one another. The United Nations Educational, Scientific and Cultural Organisation (UNESCO) called for a temporary ban on any use of germ line gene editing [ 18 ]. The Society for Developmental Biology 'supports a voluntary moratorium by members of the scientific community on all manipulation of pre- implantation human embryos by genome editing ' [ 19 ]. The Washington Summit (2015) organizers (National Academy of Sciences, the U.S. National Academy of Medicine, the Chinese Academy of Sciences and the U.K.’s Royal Society) recommended against any use of it in the clinic at present [ 17 ] and specified that with increasing scientific knowledge and advances, this stance 'should be revisited on regular basis' [ 17 ]. Indeed, this was done, to some extent, in a follow-up report by the US National Academy of Sciences and National Academy of Medicine, in which the tone of the recommendations appear much more open towards allowing germ line modifications in the clinic [ 20 , 21 ]. Meanwhile, the 'Hinxton group' also stated that gene editing 'is not sufficiently developed to consider human genome editing for clinical reproductive purposes at this time' [ 22 ] and they proposed a set of general recommendations to move the science of gene editing ahead in an established and accepted regulatory framework. Despite these differences, at least two arguments are consistent throughout these guidance documents: (1) the recognition of the need for further research regarding the risks and benefits; and (2) the recognition of the need for on-going discussion and/or education involving a wide range of stakeholders (including lay publics) regarding the potential clinical use and ethical and societal issues and impacts of heritable gene editing. It should be noted, however, that in the 2017 National Academies of Science, and of Medicine Report, the role of public engagement (PE) and dialogue was presented within the context of having to discuss the use of gene editing for enhancement vs. therapy (rather than somatic vs. heritable gene editing, which was the case in the 2015 summit report) [ 20 , 21 ].
Although many stakeholders, including scientists, clinicians and patients are enthusiastic about the present and potential future applications of these more efficient tools in both the research and clinical contexts, there are also important concerns about moving forward with gene editing technologies for clinical use in humans, and to some extent, for use in the laboratory as well. As we have learned from other ethically sensitive areas in the field of genetics and genomics, such as newborn screening, reproductive genetics or return of results, normative positions held by different stakeholders may be dissimilar and even completely incompatible. This might be influenced by various factors, such as commercial pressure, a technological imperative, ideological or political views, or personal values. Furthermore, it is clear that associated values often differ between different stakeholder groups, different cultures and countries (e.g., where some may be more/less liberal), making widespread or global agreement on such criteria very difficult, if not impossible to reach [ 23 , 24 ].
From this perspective, it was important to study the opportunities and challenges created by the use of gene editing (with CRISPR-Cas9 and other similar tools) within the Public and Professional Policy Committee (PPPC) 2 of the European Society of Human Genetics (ESHG; https://www.eshg.org/pppc.0.html ). Our committee advances that ESHG members and related stakeholders should be aware of, and if possible, take part in the current debates surrounding gene editing. Although not all genetics researchers will necessarily use gene editing in their research, and while gene editing as a potential treatment strategy, may appear, initially, somewhat separate from the diagnostics-focused present day Genetics Clinic, we believe that these stakeholders have an important role to play in the discussions around the development of these tools. For one, their expertise in the science of genetics and in dealing with patients with genetic diseases makes them a rare set of stakeholders who are particularly well placed to not only understand the molecular aspects and critically assess the scientific discourse, but also understand current clinic/hospital/health system resources, as well as human/patient needs. Furthermore, in more practical terms, one could consider that clinical genetics laboratories could be involved in the genome sequencing needed to verify for off-target events in somatic gene editing; and that clinical geneticists and/or genetic counsellors could be involved in some way in the offer of such treatment, especially in any counselling related to the genetic condition for which treatment is sought.
The PPPC is an interdisciplinary group of clinicians and researchers with backgrounds in different fields of expertise including Genetics, Health Law, Bioethics, Philosophy, Sociology, Health Policy, Psychology, as well as Health Economics. As a first step, a sub-committee was assigned the task to specifically study the subject of gene editing (including attending international meetings on the subject) and report back to the remaining members. Subsequently, all PPPC members contributed to a collective discussion during the January 2016 PPPC meeting in Zaandam, The Netherlands (15–16 January 2016). At this meeting, a decision was reached to develop an article outlining the main areas that need to be addressed in order to proceed responsibly with human gene editing, including a review of the critical issues for a multidisciplinary audience and the formulation of crucial questions that require answers as we move forward. A first draft of the article was developed by the sub-committee. This draft was further discussed during the 2016 ESHG annual meeting in Barcelona (21–24 May 2016). A second draft was developed and sent out for comments by all PPPC members and a final draft of the article was concluded based on these comments. Although the work herein acts as guidance for further discussion, reflection and research, the ESHG will be publishing separate recommendations on germ line gene editing (accepted during the 2017 annual meeting in Copenhagen, Denmark).
In the context of the ongoing discussion and debate surrounding gene editing, we present herein three crucial areas that merit the most attention at this stage in the responsible development and use of these gene editing technologies, particularly for uses that directly or indirectly affect humans:
- Conducting careful scientific research to build an evidence base.
- Conducting ethical, legal and social issues (ELSI) research.
- Conducting meaningful stakeholder engagement, education, and dialogue (SEED).
Although the main focus of this discussion article is on the use of gene editing in humans (or in human cells) in research and in the clinic for both somatic and heritable gene editing, we also briefly mention the use of gene editing in non-humans as this will also affect humans indirectly.
Conduct ongoing responsible scientific research to build a solid evidence base
The benefits, as well as risks and negative impacts encountered when conducting gene editing in any research context should be adequately monitored and information about these should be made readily available. Particular attention should be paid to the dissemination of the information by reporting and/or publishing both the 'successful' and 'unsuccessful' experiments including the benefits and risks involved in experiments using gene editing in both human and non-human cells and organisms (Table 1 ).
Example of questions that should be addressed regarding building a scientific evidence base for gene editing
Example of questions | |
---|---|
Conduct ongoing responsible scientific research to build a solid evidence base, especially regarding risk and benefits | Are the current expectations and practices of sharing the results of academic and commercial research, especially with respect to the risks and benefits, adequate for the current and future field of gene editing? |
Should there be a specific system established for the (systematic) monitoring of some types of basic and (pre-) clinical research? | |
If so, which type of research would be required to adhere to this? All research, or only the research conducted in human cells? | |
Who should/will take responsibility for this monitoring or reporting? And, where will the money to organize and sustain these efforts come from? | |
How could or should an informative long-term medical surveillance of human patients be organized? | |
Following treatment, would patients be obliged to commit to lifelong follow-up? How would this be done while still respecting individual autonomy? | |
For each of the above questions, we can also ask, who should decide the answers to these questions? Based on what criteria? |
An evidence base regarding actual (and potential) health risks and benefits relevant to the use of gene editing in the human context still needs to be built. Therefore, a discussion needs to be held regarding what type of monitoring, reporting and potential proactive search for any physically based risks and benefits should be conducted by researchers using gene editing. Hereby, various questions emerge: are the current expectations and practices of sharing the results of academic and commercial research adequate for the current and future field of gene editing? Should there be a specific system established for the (systematic) monitoring of some types of basic and (pre-) clinical research? If so, which stakeholders/agencies should or could be responsible for this? How could or should an informative long-term medical surveillance of human patients be organized? Following treatment, would patients be obliged to commit to lifelong follow-up? And, if relevant, how could long-term consequences be monitored for future generations? For example, if heritable gene editing was allowed, from logistical and ELSI perspectives, there would be many challenges in attempting to ensure that the initial patients (in whom gene editing was conducted), as well as their offspring would report for some form of follow-up medical check-ups to assess the full impact of gene editing on future generations while still respecting these individuals’ autonomy.
Although the availability of results and potential monitoring are especially important in a biomedical context for all experiments and assays conducted in human cells, and especially in any ex vivo or in vivo trials with humans, relevant and useful information (to the human context and/or affecting humans) can also be gleaned from the results of experiments with non-human animals and even plants. Furthermore, as clearly explained by Caplan et al. [ 2 ], gene editing in insects, plants and non-human animals are currently taking place and may have very concrete and important impacts on human health long before any gene editing experiments are used in any regular way in the health-care setting. As such, while keeping a focus on human use, there should also be monitoring of the results in non-human and non-model organism experiments and potential applications [ 2 ]. Effects might include change of the ecosystem, of microbial environment, (including the microbiome, of parasites and zoonosis, which can involve new combinations with some disappearing, and/or new unexpected ones appearing), change to vegetation, which has a reflection on our vegetal food and on animals’ food and natural niche [ 25 ]. All this will have an impact on the environment, and consequently on organisms (including humans) who are exposed to this altered environment, hence the monitoring of risks and benefits is very important. Especially with gene editing of organisms for human consumption (in essence, genetically modified organisms), it will be important to note that the absence of obvious harms does not mean that there are no harms. Proper studies must be conducted and information regarding these should be made readily available.
Ongoing reflection, research and dialogue on the ELSI of gene editing as it pertains to humans
Research on the ELSI and impacts of human gene editing should be conducted in tandem with the basic scientific research, as well as with any implementations of gene editing in the clinic. Appropriate resources and priority should be granted to support and promote ELSI research; it should be performed unabated, in a meaningful way and by individuals from a diverse range of disciplines (Table 2 ).
Example of questions needed to be addressed for the ethical, legal, and social issues research (ELSI) of gene editing
Area | Example of questions |
---|---|
ELSI of somatic cell gene editing | Does the current legal framework need amendments/additions to address somatic gene editing? If so, who will further shape the legal framework for somatic gene editing? |
Do present clinical trial principles and protocols suffice? | |
How will trials in somatic gene editing be conducted and evaluated? | |
Do we need particular protection or status for patients in such trials? | |
What procedures will be instilled for patients receiving such treatments (e.g., consent, genetic counselling, follow-up monitoring)? | |
To what extent will commercial companies be able to, or be allowed to offer, potentially upon consumer request, treatments based on techniques where so much uncertainty regarding harms remains? | |
Which health-care professionals will be involved in the provision of somatic gene therapy and the care of patients who undergo such treatments? | |
How will we ensure fair access to such technology? | |
How will we ensure that the use is driven by need and not the technological imperative? | |
Who will decide on roles and responsibilities in this novel context? | |
Based on what criteria will the eligible diseases/populations to be treated be chosen? | |
How can we ensure that research funds are allocated to ELSI research proportional (in some way) to the amount of research on gene editing. | |
ELSI of heritable gene editing | Should gene editing of human germ line cells, gametes and embryos be allowed in basic research—for the further understanding of human biology (e.g., human development) and without the intention of being used for creating modified human life? |
Should gene editing of germ line cells, gametes or embryos or any other cell that results in a heritable alteration be allowed in humans in a clinical setting? | |
What, if any principles or reasoning would justify the use of hereditary gene editing in humans in a clinical context given the current ban on such techniques in many jurisdictions? | |
Why should we consider using heritable gene editing in the clinic if there are alternative ways for couples to have healthy (biologically related) children? Who will decide? Based on what criteria? | |
Should we first understand the risks and benefits of somatic gene editing before even seriously considering heritable gene editing? | |
What are the roles, and responsibilities of different actors in these decisions? | |
How do commercial incentives and the technological imperative play a role in these decisions? | |
If we do entertain its use, what, if any criteria, will be safe enough according to different stakeholders (scientists, ethicists, clinicians, policy makers, patients, general public) for it to be legitimate to consider using gene editing for reproductive use? Who will set this safety threshold and based on what risk/benefit calculations? | |
If heritable gene editing was allowed, how would the fact that for the first time, a human (scientist or clinician) would be directly editing the nuclear DNA of another human in a heritable way cause some form of segregation of types of humans? Creators and the created? | |
If ever allowed, should heritable human gene editing be permitted only for specific medical purposes with a particular high chance of developing a disease (e.g., only when parents have a-near-100% risk of having a child affected with a serious disorder), and if so, would it matter if the risk is not 100%, but (much) lower? | |
How can we, or should we define/demarcate medical reasons from enhancement? And, as was posed above for the use in somatic cells, for what medical conditions will gene editing be considered appropriate for use? What will the criteria be and who will decide? |
Ongoing research, reflection and dialogue should address all ELSI 3 salient to gene editing. With respect to gene editing in humans, both somatic and germ line/heritable embryonic gene editing contexts should be addressed. As stated above, we should also study the ELSI of gene editing in non-human and non experimental/model organisms, including issues surrounding the potential (legal and logistical related to implementation) confusions surrounding the use of the terms genetically modified organisms vs. the term gene-edited organisms.
Somatic gene editing
Although somatic gene editing is not free from ethical, legal and social implications—it is, in many respects, similar to more traditional 'gene therapy' approaches in humans—it has been suggested that in many cases, the use of somatic gene editing does not challenge existing ethical, legal and social frameworks as much as heritable gene editing. However, as with any new experimental therapeutic, the unknowns still outweigh what is known and issues of risk assessment and safety, risk/benefit calculation, patient monitoring (potentially for long periods), reimbursement, equity in access to new therapies and the potential for the unjustified draining of resources from more pressing (albeit less novel) therapies, particular protection for vulnerable populations (e.g., fetuses, children (lacking competencies)), and informed consent remain important to study further [ 26 ].
Furthermore, as with any new (disruptive) technology or application, there often remains a gap to be filled between the setting of abstract principles or guidelines and how to apply these in practice. Indeed, important questions and uncertainties surrounding somatic gene editing both in research and in the clinic remain, including, but not limited to: do the established (national and international) legal and regulatory frameworks (e.g., Regulation (EC) no. 1394/2007 on advanced therapy medicinal products) need further shaping/revisions to appropriately address somatic gene editing (including not just issues with the products per se but also for issues related to potential health tourism)? And if so, how would this best be accomplished? Do present clinical trial principles and protocols suffice? How exactly will trials in somatic gene editing be conducted and evaluated? Do we need particular protection or status for patients in such trials? What procedures will be instilled for patients receiving such treatments (e.g., consent, genetic counselling, follow-up monitoring)? Furthermore, to what extent will commercial companies be able to, or be allowed to offer, potentially upon consumer request, treatments based on techniques where so much uncertainty regarding harms remains? Importantly, which health-care professionals will be involved in the provision of somatic gene therapy and the care of patients who undergo such treatments? Who will decide on roles and responsibilities in this novel context? And, based on what criteria will the eligible diseases/populations to be treated be chosen? Indeed, these questions can also all be applied to the context of heritable gene editing, which is discussed below.
Germ line/heritable gene editing
With respect to germ line or heritable gene editing in humans, the ELSI are more challenging than for somatic gene editing, yet they are not all new per se either. Some of these previously discussed concerns include, but are not limited to: issues addressing sanctity of human life, and respect for human dignity, the moral status of the human embryo, individual autonomy, respect and protection for vulnerable persons, respect for cultural and biological diversity and pluralism, disability rights, protection of future generations, equitable access to new technologies and health care, the potential reduction of human genetic variation, stakeholder roles and responsibilities in decision making, as well as how to conduct 'globally responsible' science [ 16 , 2 , 11 , 18 ]. Discussions and debates over some of these topics have been held numerous times in the last three decades, especially within the context of in vitro fertilization, transgenic animals, cloning, pre-implantation genetic diagnosis (PGD), research with stem cells and induced pluripotent stem cells, as well as related to the large scope of discussion around 'enhancement' [ 13 ]. Although it is important to identify and reflect on more general ELSI linked with heritable gene editing and these different contexts, it is also vital to reflect on the ELSI that may be (more) specific to this novel approach. For example, would the fact that for the first time a human (scientist or clinician) would be directly editing the nuclear DNA of another human in a heritable way cause some form of segregation of types of humans? Creators and the created? [ 27 ] Clearly, we need time for additional reflection and discussion on such topics. Distinguishing the ELSI between different yet related contexts will allow for a deeper understanding of the issues and the rationale behind their (un)acceptability by different stakeholders.
A major contextual difference in the current discussions regarding germ line/heritable gene editing is that we have never been so close to having the technology to perform it in humans in a potentially safe and effective manner. Hence, as we move closer to this technical possibility and as we work out the scientific issues of efficiency and safety, the discussions orient themselves increasingly towards the ELSI regarding whether or not we want to even use heritable gene editing in a laboratory or clinical setting, and if so, how we want it to be used, by whom and based on which criteria? This includes, but is not limited to the following questions: should gene editing of human germ line cells, gametes and embryos be allowed in basic research—for the further understanding of human biology (e.g., human development) and without the intention of being used for creating modified human life? Some jurisdictions, such as the UK, have already answered this question, and are allowing this technique in the research setting in human cells in vitro (they will not be placed in a human body, the research will only involve studying the human embryos outside of the body) whereby researchers need to apply for permission to conduct such research. Some believe that allowing this will inevitably lead to the technology being used in the clinic (the so-called 'slippery slope' argument). This, then, brings us to the question at the centre of the debate: should gene editing of germ line cells, gametes or embryos or any other cell that results in a heritable alteration be allowed in humans in a clinical setting? Germane to this issue is another vital question: what, if any, principles or reasoning would justify the use of hereditary gene editing in humans in a clinical context given the current ban on such techniques in many jurisdictions? The new EU clinical trial Regulation (536/2014 Art 90 al.2.) does not allow germ line modification in humans. Should there be leeway for reconsidering this ban in the future in view of the possible benefits of therapeutic germ line gene editing? Should we first understand the risks and benefits of somatic gene editing before even seriously considering heritable gene editing? If we consider that it could be used in some situations, should we only consider using germ line gene editing in the clinic if there are absolutely no other alternatives? Should already established and potentially safer 4 reproductive alternatives, like PGD, be the approaches of choice before even considering germ line gene editing? If we do entertain its use, what, if any criteria, will be safe enough according to different stakeholders (scientists, ethicists, clinicians, policy makers, patients, general public) for it to be legitimate to consider using gene editing for reproductive use? Who will set this safety threshold and based on what risk/benefit calculations? Furthermore, if ever allowed, should heritable human gene editing be permitted only for specific medical purposes with a particular high chance of developing a disease (e.g., only when parents have a-near-100% risk of having a child affected with a serious disorder), and if so, would it matter if the risk is not 100%, but (much) lower? In addition, how can we, or should we define/demarcate medical reasons from enhancement? And, as was posed above for the use in somatic cells, for what medical conditions will gene editing be considered appropriate for use? What will the criteria be and who will decide?
Taking a step back and looking at the issues from a more general perspective, such ELSI research and reflection will need to address, among others, questions that fall under the following themes:
- the balance of risks and benefits for individual patients and also for the larger community and ecosystem as a whole;
- the ethical, governance and legislative frameworks;
- the motivations and interests 'pushing' gene editing to be used;
- the roles and responsibilities of different stakeholders in ensuring the ethically acceptable use of gene editing, including making sure that every stakeholder voice is heard;
- the commercial presence, influence, and impact on (the use of) gene editing;
- the rationale behind the allocation of resources for health care and research and if and which kind of shift might be expected with the new technologies on the rise.
Additional overarching issues relating to ELSI include the need to take a historical perspective and consider previous attempts to deal with genetic technologies and what or how we can learn from these; the need to consider how group actors could or should accept a shared global responsibility when it comes to the governance of gene editing; the potential eugenic tendencies related to new technologies used to eliminate disease phenotypes; the responsibility of current society for future generations; the way different stakeholders may perceive and desire to eliminate (genetic) risk and/or uncertainty by using new technologies such as gene editing; and the potential role(s) different stakeholders, including 'experts', may inadvertently play in propagating a false sense of control over human health.
Although the human context is where much of the attention currently resides, and is indeed, the focus of this article, as mentioned above, we also stress that many concerns and ELSI also stem from the use of gene editing in non-human organisms (plants, insects and microorganisms), the study of which, could inform the human context. More importantly, given that the use of gene editing in these organisms is currently taking place in laboratories and, if released, some of these gene-edited organisms could have a large impact on the environment and society [ 2 ], the ELSI of gene editing in non-human organisms should also be seriously addressed. In this respect, the current debates over definitions and whether plants and non-human animals in which gene editing is performed are considered (legally) genetically modified organisms (GMOs) are particularly important to consider; indeed, this legal stance may be a misleading way to describe the scientific differences in practice. Moreover, the manipulation of definitions may also be used to circumvent the negative press and opinions surrounding GMOs in Europe. Last, but not least, the use of gene editing for the creation of biologic weapons is a possibility that must be discussed and adequately managed [ 2 ].
In order to ensure that the appropriate ELSI research is conducted to answer these myriad questions, ELSI researchers must ensure adequate understanding of scientific facts and possibilities of gene editing, ensure appropriate use of robust methods [ 29 ] to answer specific ELSI questions, as well as learn from previous research on related themes such as (traditional) gene therapy, reproductive technologies, and GMOs. Furthermore, funding will have to be prioritized for ELSI research. National and European funding agencies should ensure that ELSI funding is given in certain proportion to how much gene editing research is being conducted in the laboratory and (pre) clinical domain. In practice, this will mean ensuring that there are adequate review panels for stand-alone ELSI grants, which do not usually fall within any one traditional academic field (e.g., philosophy, law or social sciences). The requirement of including ELSI work packages within science grants may also be useful if such work packages are conducted by ELSI experts (and this is verified by the funding agencies), that they are given enough budget to conduct research and not only offer services, and that the ELSI work package is not co-opted by the science agenda. Spending money on ELSI research has already allowed for the information to be used in more applied ways. Among others, ELSI research has contributed to helping individual researchers understand what kind of research they are (not) allowed to do in certain countries or regions; helped to design appropriate consent forms for research and clinic; and has helped inform policy decisions.
As ELSI are identified, studied and discussed, it will be of utmost importance to communicate these with as many publics as relevant and possible in a clear and comprehensive way so that the largest number of different stakeholders can understand and engage in a discussion about these issues. With respect to engaging non-academic and non-expert audiences in meaningful dialogue, the challenges are greater. Yet, as this is a vital element of conducting science and preparing clinical applications in a responsible manner and stretches beyond the academic focus of ELSI we propose to distinguish a third domain dedicated to such stakeholder engagement, education and dialogue (SEED) described below.
Stakeholder engagement, education and dialogue (SEED)
To deliver socially responsible research (and health care), an ongoing robust and meaningful multidisciplinary dialogue among a diverse group of stakeholders, including lay publics, should be initiated and maintained to discuss scientific and ethically relevant issues related to gene editing. Publics must not only be asked to engage in the discussion, but they should also be given proper information and education regarding the known facts, as well as the uncertainties regarding the use of gene editing in research and in the clinic. In this way, the two focal areas described above will feed into these SEED goals. Stakeholders should also be given the tools to be able to reflect on the ethically relevant issues in order to help informed decision making. Appropriate resources and prioritization should be granted to support and promote SEED (Table 3 ).
Examples of questions to be answered regarding stakeholder, engagement, education and dialogue (SEED) for gene editing
Example of questions | |
---|---|
Planting SEEDs for gene editing | What are the roles and responsibilities of different stakeholders in setting up and maintaining responsible engagement, education and dialogue? |
What will, and what should be the role of scientists and other academics in this type of popular media communications, and engagement activties? | |
Since PE can have different purposes, before each activity, we must consider: what are our goals? And, what method of engagement will best meet these goals? | |
How will the multitude of voices we want to involve in PE be 'weighted' against each other? | |
What role will different stakeholders’ inputs and 'preferences' play in the debate and in the decision-making process? | |
How do we make sure that all voices are heard? | |
How will these voices be weighed and considered, if at all, in policy making? | |
How can we ensure that public education will not be reduced to a token work package in science grants and/or to campaigns that try to convince for or against gene editing? | |
How can we make sure that such public education and engagement is accessible to all, including in countries that may currently not have the resources to take on such 'SEED' activities? |
As mentioned in the introduction, the statements addressing gene editing published by different groups and organizations have highlighted the need for an ongoing discussion about human gene editing among all stakeholders, including experts, and the general public(s) [ 8 , 9 , 17 ], In calling for an 'ongoing international forum to discuss the potential clinical uses of gene editing', the organizing committee of the International Summit on Human Gene Editing stated that
'The forum should be inclusive among nations and engage a wide range of perspectives and expertise – including from biomedical scientists, social scientists, ethicists, health care providers, patients and their families, people with disabilities, policymakers, regulators, research funders, faith leaders, public interest advocates, industry representatives, and members of the general public' [ 17 ].
Hence, this implies that not only should different expertise be represented in this ongoing discussion, but lay publics should also be included. For this to be a meaningful and impactful endeavour, all stakeholders involved should be appropriately informed and educated about the basic science and possibilities of gene editing. Academic/professional silos, differences in language, definitions, approaches and general lack of experience with multi- and inter-disciplinary work are all barriers to involving different expert stakeholders in meaningful exchange and dialogue. Some first constructive steps have included the posting online of meeting and conference presentations on gene editing (e.g., the 3 days of the Washington Summit ( http://www.nationalacademies.org/gene-editing/Gene-Edit-Summit/index.htm .), Eurordis webinars and meetings aimed at informing patients, http://www.eurordis.org/tv ). Beyond this, one important barrier to having a truly meaningful and inclusive multidisciplinary discussion about new technologies is the (potential) lack of knowledge and/or understanding of different publics [ 30 ]. Indeed, it is not reasonable for experts to expect that all concerned stakeholders are properly informed about the science and/or the social and ethical issues, which are important requisites for having meaningful and productive conversations about responsible gene editing. Furthermore, a pitfall we must avoid is using PE with the aim of persuading or gaining acceptance of technologies instead of 'true participation' [ 31 ] and as a means to allow for supporting informed opinions.
Another critical issue is the role and influence of different stakeholders, including the media, in educating and informing the public. What are the roles and responsibilities of different stakeholders in setting up and maintaining responsible engagement and dialogue? What will, and what should be the role of scientists in popular media communications and other SEED activities? Where will the funding for these activities come from? Financial and temporal resources will have to be reserved for such SEED regarding gene editing. Resources will also be needed to conduct further research on the best way to engage different publics and to study whether engagement strategies are successful.
Moreover, before engaging different publics and asking for their feedback, whichever stakeholders take on this task must seriously reflect on the precise reasons for which lay publics are being engaged. What is the goal? And, what method of engagement will best meet these goals? There is also a need for honest evaluation of engagement efforts to report on their impacts and outcomes. Indeed, the purposes of PE in science can vary widely, including, among others, informing, consulting and/or collaborating; [ 32 ] clearly each of these implies different levels of participation by publics, and by extension, different levels of influence on a topic. Importantly, there are a long list of questions that also need to be answered for PE (Table 3 ), including but not limited to how different voices will be weighed and if or how they will be used in any policy or decision making.
The value of PE in the form of public dialogue in a democratic society, (and we would specify its contribution to responsible science) is very well summarized by Mohr and Raman (2012) in a perspective piece on the UK Stem Cell Dialogue: [ 31 ]
'The value of public dialogue in a democratic society is twofold. From a normative perspective, the process of PE is in itself a good thing in that the public should be consulted on decisions in which they have a stake. From a substantive standpoint, PE generates manifold perspectives, visions, and values that are relevant to the science and technologies in question, and could potentially lead to more socially robust outcomes (which may differ from the outcomes envisaged by sponsors or scientists)' [ 31 ].
Particularly for the purposes of gene editing, we consider SEED a way to try to ensure that decisions on a subject that is filled with uncertainties, and could have important implications for society for generations to come, is not left in the hands of a few. We want to underline the need for: lay publics to be informed to support transparency; lay publics to be educated to support autonomy and informed opinion/decision making; different voices and concerns to be heard and considered through ongoing dialogue to help ensure that no one stakeholder group pursue their interests unchecked. Although it is beyond the scope of this article to go into any detail, it is important to take the time to learn from past and ongoing engagement efforts in science in general [ 32 ], as well as in biomedicine, including areas like stem cell research [ 31 ] and genetics [ 30 , 33 ]. For example, we can learn about: how PE can generate value and impact for a society, as well as how to conceive of and evaluate a PE programme [ 32 ]; the nuances around 'representative samples' and if they really are representative [ 31 ]; how letting citizens be the 'architects' rather than just participants of engagement (activities) could help to ward against the generation of 'predetermined outcomes' [ 31 ]; the utility of deliberative PE to 'offer useful information to policy makers [ 30 ]. Given all the different reasons for PE, and given the higher standards expected for PE in recent years [ 34 ] it is to be expected that each PE activity will have to be adjusted for the specific context. There are, also, useful tools for PE from a European funded project called 'PE2020, Public Engagement Innovations for Horizon 2020' [ 35 ], which has as an aim to 'to identify, analyse and refine innovative public engagement (PE) tools and instruments for dynamic governance in the field of Science in Society (SiS)' [ 35 ].
As already mentioned above for ELSI research, funding agencies will have to prioritize resources for these SEED activities, and the strategies we outlined for ELSI, could also apply for SEED.
In the midst of a plethora of debate over gene editing, different stakeholder views, preferences, agendas and messages, it is crucial to focus our limited resources, including human resources, time and finances on the most important areas that will enable and support the responsible use of gene editing. We have identified the following three areas that merit an equitable distribution of attention and resources in the immediate and medium-term future:
- Conducting ELSI research.
Indeed, one way to ensure that each of these three important areas receive adequate financial support to conduct the necessary work would be for international and national funding agencies to announce specific funding calls on gene editing. They could also encourage or require that scientific projects focused on gene editing include ELSI and SEED along with the scientific work packages. Furthermore, understandably, priorities need to be made with respect to resource allocation in the biomedical sciences, especially in such uncertain financial contexts, however, as expressed at the World Science Forum in Budapest in November 2011, we must ward against scarce funding being funnelled to single disciplines since it is common knowledge that much of the most valuable work is now multidisciplinary [ 36 ]. Moreover, at such a time funding entities must not 'expel' the social sciences 'from the temple' but rather, the hard sciences should 'invite them in to help public engagement' [ 36 ].
Acknowledgements
We thank all members of the Public and Professional Policy Committee of the ESHG for their valuable feedback and generosity in discussions. Members of PPPC in 2015–2017 were Caroline Benjamin, Pascal Borry, Angus Clarke, Martina Cornel, Carla van El, Florence Fellmann, Francesca Forzano, Heidi Carmen Howard, Hulya Kayserili, Bela Melegh, Alvaro Mendes, Markus Perola, Dragica Radijkovic, Maria Soller, Emmanuelle Rial-Sebbag and Guido de Wert. We also thank the anonymous reviewers for their constructive comments, which have helped to improve the article. Part of this work has been supported by the Swedish Foundation for Humanities and Social Science under grant M13-0260:1, and the CHIP ME COST Action IS1303.
Compliance with ethical standards
Conflict of interest.
The authors declare that they have no competing interests.
1 In this category, we include the editing of germ line cells, or embryonic cells, or even somatic cells that are edited and promoted to then become germ line cells in such a way that the alterations would be heritable.
2 This group studies salient ethical, legal, social, policy and economic aspects relating to genetics and genomics.
3 Herein, the terms 'ethical', 'legal' and 'social' are used in a broad sense, where, for example, issues such as economic evaluations, public health prioritization and other related areas would also be included. Indeed the first goal of 'SEED' (see below) is also, to some extent, part of ELSI research, however, given the paucity of meaningful PE in the past, combined with strong consensus regarding the current need and importance of such activities, we have chosen to highlight it separately. We also wish to stress the difference between academic ELSI research and the work of ethics review committees. Although both deal with ethical and legal issues, the former has as a main goal to advance research and does not act as a policing body, nor does it have an agenda per se. Furthemore, ELSI research does not only identify issues to be addressed but also works with scientists and policy makers to address the issues responsibly.
4 It is important to note that despite attempts at addressing these issues, even for technologies such as PGD [ 28 ].

IMAGES
VIDEO
COMMENTS
than 10,000 inherited diseases might be p revented by correcting harmful genetic mutations. Many such diseases are serious, such as cystic fibrosis, Huntington's disease, and breast cancer linked to mutations in the BRCA gene. Purpose: Using genome editing to correct a genetic mutation in human embryos that can be passed down from parents .
Genetic engineering opens new possibilities for biomedical enhancement requiring ethical, societal and practical considerations to evaluate its implications for human biology, human evolution and our natural environment. In this Commentary, we consider human enhancement, and in particular, we explore genetic enhancement in an evolutionary context.
To help demonstrate the power of biotechnology, consider the following analogy: Imagine you have two decks of cards, one red and one blue, and each deck contains all the genes of a potato.
For the case study presented by Peter Kareiva and Michelle Marvier, I will introduce a different set of philosophical concerns that focus on ways of framing (or interpreting) risks involved with genetically engineered food.
It is typically diagnosed in infants and toddlers. The underlying cause is a genetic mutation that inhibits production of a protein involved in building and maintaining those motor neurons. The ...
The case studies are first described in a preliminary fashion in this chapter. Other chapters build on these case studies with deeper discussion of issues pertinent to value-based concerns, scientific techniques to mitigate harms, risk assessment, public engagement, and governance. ... It remains to be seen if such genetic engineering ...
Genetic engineering is the act of modifying the genetic makeup of an organism. Modifications can be generated by methods such as gene targeting, nuclear transplantation, transfection of synthetic ...
Read chapter 3 Case Studies to Examine Questions About Gene-Drive Modified Organisms: Research on gene drive systems is rapidly advancing. ... Other research aims to use genetic engineering approaches to control rodent populations including RNA interference and developing transgenes that cause female progeny to develop as males or prevent all ...
Genetic engineering has provided humans the ability to transform organisms by direct manipulation of genomes within a broad range of applications including agriculture (e.g., GM crops), and the ...
Genetic Engineering in Plants Explore the process of developing genetically engineered crops, from gene identification to crop transformation, plant testing, and regulatory approval. ... His laboratory study was the first to perform a large-scale functional genomics project in any organism, and has launched many technologies in genomics and ...
In each case, the efficiency of ... These studies demonstrate how genetic engineering and genome editing can be used to produce fruits with enhanced flavor, texture, and nutrient levels. Trans ...
The simple, ethical case for gene editing. Gene editing is a form of 'ultimate cure': it treats disease at its very root. This edited extract from the new book, Genes for Life, explores the ethical implications of this rapidly evolving technology. For over three decades, scientists have had the ability to alter the genomes of other species ...
Human Genetic Engineering (HGE) - gene editing applied to human cells. CRISPR/Cas9 - gene editing technology derived from bacterial immune system. Germline gene editing - a process which alters the genome of an embryo, so that the entire organism has altered genes and can pass those genes down to offspring.
Harvard researchers, others share their views on key issues in the field. Medicine is at a turning point, on the cusp of major change as disruptive technologies such as gene, RNA, and cell therapies enable scientists to approach diseases in new ways. The swiftness of this change is being driven by innovations such as CRISPR gene editing, which ...
Genetic engineering, which refers to the direct alteration of an organism's genetic material using biotechnology (Christou, ... Are the dominant and recessive plant disease resistance genes similar? A case study of rice R genes and Xanthomonas oryzae pv. oryzae races. Genetics 159: 757-765 [PMC free article] ...
Human genome-editing is banned by guidelines, laws and regulations in most countries. However, the first criminal case on genome-edited babies was sentenced in China in 2019. In this commentary we discuss our legal reflections on this case. Genome-editing on healthy embryos of human may lead to irreversible mutations and serious consequences on the heredity of future generations, while its ...
By using genetically engineered animals as a case study, Schicktanz argues that genetic engineering presents "a troubling shift for all human-animal relationships." Opinions regarding whether limits can, or should, be placed on genetic engineering are often dependent on people's broader worldview.
In April 2015, scientists in China published a paper in an online journal, Proteins & Cells, about experiments editing the genomes of non-viable human embryos (Liang et al. 2015). The research team, led by Junjiu Huang, used an engineered enzyme complex, called CRISPR-Cas9, to target and edit the HBB gene that codes for human β -globin protein.
How Gregor Mendel discovered the laws of inheritance for certain types of traits. The science of heredity, known as genetics, and the relationship between genes and traits. How gametes, such as eggs and sperm, are produced through meiosis. How sexual reproduction works on the cellular level and how it increases genetic variation.
Published: December 5, 2019 10:52pm EST Updated: December 8, 2019 8:39pm EST. A number of things may have gone wrong when researchers edited Chinese twins Lulu and Nana's genome. Either way, the ...
It should be noted, however, that in the 2017 National Academies of Science, and of Medicine Report, the role of public engagement (PE) and dialogue was presented within the context of having to discuss the use of gene editing for enhancement vs. therapy (rather than somatic vs. heritable gene editing, which was the case in the 2015 summit ...
1 The term broadly encompasses both genetics—the study of genes and ... 3 A gene drive is a genetic engineering technology—adding, deleting, disrupting,
Through its various case studies, As Gods shows that the ethical minefield is not some rhetorical flourish—it is the very world of everyday genetic engineering research. "When scientists muse about the political and sociological consequences of their work, the results are often unedifying," Cobb complains. His moral elbows are sharp, and ...
While the benchmarks in Table 1 capture challenging characteristics, not all of them replicate real-world complexity.. Despite MOEAs' potential in unbiased decision support (Eiben and Smith Citation 2015), a gap exists between benchmark test problems and real-world applications.Studies in water resources, with diverse complexities, reveal MOEAs often struggle (Gupta et al. Citation 2020; Reed ...