An official website of the United States government
Official websites use .gov A .gov website belongs to an official government organization in the United States.
Secure .gov websites use HTTPS A lock ( Lock Locked padlock icon ) or https:// means you've safely connected to the .gov website. Share sensitive information only on official, secure websites.
- Publications
- Account settings
- Advanced Search
- Journal List


New developments in tuberculosis diagnosis and treatment
Cara m gill, lorraine dolan, laura m piggott, anne marie mclaughlin.
- Author information
- Article notes
- Copyright and License information
Corresponding author: Cara M. Gill ( [email protected] )
Received 2021 Oct 1; Accepted 2021 Nov 16; Issue date 2022 Mar.
Breathe articles are open access and distributed under the terms of the Creative Commons Attribution Non-Commercial Licence 4.0.
Tuberculosis (TB) is a major cause of morbidity and mortality worldwide. It is estimated that 25% of the world's population are infected with Mycobacterium tuberculosis , with a 5–10% lifetime risk of progression into TB disease. Early recognition of TB disease and prompt detection of drug resistance are essential to halting its global burden. Culture, direct microscopy, biomolecular tests and whole genome sequencing are approved methods of diagnosis; however, their widespread use is often curtailed owing to costs, local resources, time constraints and operator efficiency. Methods of optimising these diagnostics, in addition to developing novel techniques, are under review. The selection of an appropriate drug regimen is dependent on the susceptibility pattern of the isolate detected. At present, there are 16 new drugs under evaluation for TB treatment in phase I or II clinical trials, with an additional 22 drugs in preclinical stages. Alongside the development of these new drugs, most of which are oral medications, new shorter regimes are under evaluation. The aim of these shorter regimens is to encourage patient adherence, and prevent relapse or the evolution of further drug resistance. Screening for TB infection, especially in vulnerable populations, provides an opportunity for intervention prior to progression towards infectious TB disease. New regimens are currently under evaluation to assess the efficacy of shorter durations of treatment in this population. In addition, there is extensive research into the use of post-exposure vaccinations in this cohort. Worldwide collaboration and sharing of expertise are essential to our ultimate aim of global eradication of TB disease.
Educational aims
Differentiate between TB infection and TB disease.
Understand the different methods of diagnosing TB disease and resistance.
Recognise the different drugs and regimens currently in use for TB disease.
Be able to discuss risk of TB disease in TB infection, and assist patients in making an informed decision on treatment for TB infection.
Short abstract
Early detection of drug resistance is essential to our goal of global eradication of TB. Tolerable drugs and shorter regimens promote patient adherence. Treating TB infection in vulnerable groups will prevent further global spread of TB disease. https://bit.ly/3oUW0SN
Introduction
Tuberculosis (TB) is a major cause of morbidity and mortality worldwide. TB is caused by the bacillus Mycobacterium tuberculosis (Mtb ), which is spread via airborne droplets. Approximately one in four people worldwide demonstrate an immunological response to Mtb infection, which can remain dormant or progress into active disease forms [ 1 ]. Patients infected with TB who have no active signs or symptoms of disease were previously deemed to have latent TB, more recently changed to TB infection [ 2 ]. Whereas patients with active disease are termed to have TB disease. Patients with TB infection have a 5–10% lifetime risk of developing TB disease, which increases in varying states of immunodeficiency up to a 16% annual risk of activation of TB infection into TB disease in HIV patients [ 3 ]. In 2019, there were an estimated 10 million new incident cases of active TB disease worldwide [ 1 ]. Approximately two-thirds of all cases arise in eight countries alone, the vast majority of which have overwhelmed health services with limited resources [ 1 ]. This significant global burden of disease has been recognised by the World Health Organization (WHO) who launched the End TB initiative in 2016. Their aim is to reduce incidence, morbidity and mortality of this disease by improving diagnostic and therapeutic practices, as well as developing preventative strategies, through innovative research and education. By 2035, the goal is to reduce TB mortality by 95% and reduce overall incidence of TB by 90% worldwide [ 4 ]. Owing to the work of our predecessors, it has been estimated that 60 million lives have been saved globally in the 21st century so far [ 5 ].
Effective TB treatment is dependent on:
Prompt diagnosis of TB and recognition of drug resistance;
Promoting and ensuring patient adherence to regimens;
Robust contact tracing and prophylactic treatment of contacts; and
Screening for TB infection in high-risk groups.
There is ongoing extensive research into developing accurate, timely methods of detecting drug resistance, even in resource poor settings. Many effective, less toxic medications are under development. Furthermore, methods of promoting and ensuring drug adherence are being reviewed. In addition, there is vital research ongoing in proactive areas of TB prevention, such as screening for, and treatment of, TB infection and developing efficacious vaccines to halt the spread of this killer disease.
The aim of this article is to: review current practice in the diagnosis and treatment of TB; outline new diagnostic techniques under development; discuss new drug therapies and treatment regimens under review; and review the evidence for vaccination.
Improving the efficiency and accuracy of TB diagnosis contributes to treatment efficacy. Pulmonary TB should be suspected when patients present with classical symptoms such as non-resolving cough, haemoptysis, fevers, night sweats and weight loss. Extrapulmonary TB, including TB lymphadenitis, TB meningitis, laryngeal TB, Pott's disease and abdominal TB, presents in a variety of manners. Special consideration should always be given to patients who have potential TB exposure, as well as immunocompromised patients who may present atypically. The diagnosis must be made by confirming the presence of the causative pathogen, Mtb . A variety of methods are employed to confirm the diagnosis. In addition, it is essential that there is emphasis on early detection of potential drug resistance.
Drug resistance is a growing issue that threatens TB care worldwide. Traditionally this was categorised into rifampicin-resistant TB (RR-TB), multidrug-resistant TB (MDR-TB) or extensively drug-resistant TB (XDR-TB). MDR-TB is resistant to both rifampicin (RIF) and isoniazid (INH). Recently definitions have been updated to include pre-XDR-TB, which is TB that fulfils the definition for MDR-TB and RR-TB that is also resistant to any fluoroquinolone (FLQ). The updated definition for XDR-TB is strains that fulfil the definition for MDR-TB/RR-TB which are also resistant to any group A drug (namely levofloxacin (LFX), moxifloxacin (MFX), bedaquiline (BDQ) and linezolid (LZD)) [ 6 ]. Replacing the old XDR-TB definition referencing second-line injectable drugs (SLID), it highlights the trend towards use of oral regimens comprising recently developed or repurposed drugs. Despite the importance of early recognition, only 61% of patients with a new diagnosis of bacteriologically confirmed TB disease in 2019 were tested for RIF resistance [ 1 ]. This is in part related to access to diagnostics in resource-limited settings. There are numerous methods currently available, and under development, to determine drug resistance. For these diagnostics to be beneficial on a global scale they need to provide timely, accurate, cost-effective results in centres where access to power, equipment and technical expertise remains limited.
Culture of Mtb in a suitable medium remains the gold standard diagnostic test. The specimen can be cultured in solid ( e.g. Löwenstein–Jensen or Middlebrook 7H11) or liquid media ( e.g. for use with the BACTEC Mycobacterium Growth Indicator Tube (MGIT) 960 system). Sensitivity, specificity, contamination rates and time to detection vary widely amongst both media, with the WHO advocating for dual use of systems where practical. The major benefit of the advent of liquid-based systems is the rapid time to detection, often reducing time to growth by half with a mean time to detection of 12.8 days compared with 25.1–25.5 days for the previously mentioned solid media [ 7 ]. However, sub-optimal laboratory facilities in resource-limited settings often restrict its practical use [ 8 ]. While culture is not recommended for use as a first-line test, it remains an important part of TB diagnostics where persistent culture positivity can predict likelihood of relapse [ 9 ].
Direct microscopy
Direct microscopy is a fast and inexpensive method to identify acid-fast bacilli (AFB), the majority of which are mycobacteria [ 10 ]. Traditionally, Ziehl–Neelsen (ZN) stain was applied and the sample termed “smear positive” or “smear negative”, depending on the presence or absence of AFB. Efficacy is operator dependent, resulting in a broad range of sensitivities and specificities reported in international studies, 25.3–81.6% and 83.4–99%, respectively [ 11 , 12 ]. It is even less sensitive in high-risk groups, such as patients with HIV, and children [ 1 ]. Methods to improve efficacy include use of mercury vapour fluorescence and light-emitting diode (LED) microscopy, which have largely replaced traditional ZN staining [ 13 ]. Education and quality assurance for laboratory technicians is one of the most useful ways to ensure accurate diagnosis, as direct microscopy often remains the only method of diagnosis available in resource-limited settings [ 14 ]. Similar to culture, direct microscopy remains an integral part of monitoring response to treatment, measuring infectiousness, and predicting likelihood of relapse in patients who are smear positive at diagnosis.
Molecular tests
Given the limitations of culture and direct microscopy, the WHO recommends a biomolecular test as the initial diagnostic test in a suspect patient [ 1 ]. Current molecular tests endorsed by WHO include: Xpert MTB/RIF and Xpert MTB/RIF Ultra assays (Cepheid, Sunnyvale, USA); loop-mediated isothermal amplification test (TB-LAMP; Eiken Chemical, Tokyo, Japan); Truenat MTB, MTB Plus and MTBRIF Dx tests (Molbio Diagnostics, Goa, India) and lateral flow urine lipoarabinomannan assay (LF-LAM; Alere Determine TB LAM Ag, Abbott, San Diego, USA).
The WHO currently recommends Xpert (MTB/RIF or MTB/RIF Ultra) or Truenat (MTB or MTB Plus) as the initial diagnostic test of choice in suspected pulmonary TB [ 1 ]. They are cartridge based nucleic acid amplification tests (NAAT) that detect the presence of TB DNA, as well as common mutations associated with RIF resistance along the rpoB gene, within 2 h [ 15 ]. The Xpert MTB/RIF and Xpert MTB/RIF Ultra assays are also endorsed by the WHO for diagnosing extrapulmonary TB and TB in children [ 1 ]. When compared with culture diagnosis, the Xpert assays have demonstrated 89% sensitivity and 99% specificity at diagnosing pulmonary TB in adults [ 16 ]. The Xpert MTB/RIF Ultra assay has a higher sensitivity but lower specificity than the Xpert MTB/RIF assay, owing to its inability to accurately differentiate between dormant and active TB DNA [ 17 , 18 ]. While recommended for use, it is important to remember these assays have reduced sensitivity in certain populations such as children and patients coinfected with HIV, as well as in extrapulmonary TB [ 16 , 19 ]. Moreover, this technology is expensive and requires laboratory facilities with continuous access to power. To overcome this obstacle in resource-limited settings, there are a number of smaller, battery-operated technologies in development. To date, the GeneXpert Omni (Omni; Cepheid) appears to be the most promising potential candidate for widespread use. In a real-world analysis, it has been shown to be a cost-effective method when used in peripheral healthcare settings [ 20 ]. It allows diagnosis to be at/near the point of care, and thus avoids further delays and costs associated with transporting samples to specialised centres.
As well as the Omni, Cepheid is also developing the Xpert MTB/XDR assay. It aims to also detect resistant to INH, FLQ, ethionamide (ETH) and SLID. Similar to other Xpert assays, it is a NAAT that detects 16 clinically relevant mutations associated with resistance in under 90 min [ 21 ]. When compared with phenotypic drug sensitivity testing (pDST), it has a 94% sensitivity and 100% specificity at detecting drug resistance [ 21 ]. There are large scale multicentre clinical trials ongoing to establish its real-world efficacy as a follow-on test to current Xpert MTB/RIF and MTB/RIF Ultra assays, prior to consideration for WHO recommendation. This assay is of paramount importance as the early recognition of drug resistance is a prerequisite to shorter drug regimens, which will be discussed in further detail elsewhere in this review.
While most biomolecular tests are NAAT detecting the presence of Mtb DNA, the LF-LAM test detects a lipopolysaccharide present in mycobacterial cell walls. While not in use in most countries in the developed world, the LF-LAM assay has been recommended for use in HIV-coinfected patients. It is a urinary antigen test that is often employed in resource-limited settings, and is of particular benefit in cases where a sputum sample cannot be obtained. It has a 42% sensitivity in HIV patients with TB symptoms [ 22 ]. However, it cannot distinguish between mycobacterial species, and can cross react with other fungal diseases. As such, it is used as an initial test in peripheral primary care centres in areas of high TB endemicity only, to determine whether symptomatic patients with HIV should be referred for further confirmatory testing [ 23 ].
Line probe assays
Another method of molecular detection of Mtb resistance is line probe assay (LPA). Genotype MTBDR plus and Genotype MTBDR sl (Hain LifeScience GmbH, Nehren, Germany) are used for the detection of Mtb and its associated drug resistance. The WHO approved Genotype MTBDR plus employs a series of steps to detect Mtb and mutations in rpoB and katG , which confer RIF and INH resistance, respectively [ 24 ]. Additionally, it can detect the presence of inhA promoter genes that confer resistance to low dose INH, which are also typically associated with ETH and prothionamide resistance [ 25 ]. This in vitro test delivers results in <6 h [ 26 ]. When compared with traditional culture-based drug sensitivity, it is 78.5% sensitive and 100% specific at detecting RIF and INH resistance [ 27 ]. The WHO endorsed Genotype MTBDR sl 2.0 assay can also detect resistance conferring mutations of FLQ ( gyrA and gyrB ) and SLID ( rrs and eis ) [ 28 ]. Reported sensitivity and specificity are 100% and 98.9% for FLQ, and 89.2% and 98.5% for SLID [ 29 ]. Even more sensitive than NAAT at detecting FLQ resistance, this rapid test could allow for use of FLQ in patients that might otherwise have faced a lengthier regime that potentially required the interim use of SLID. However, these tests are not without limitations including low sensitivity for detecting ethambutol (ETM) and aminoglycoside resistance as demonstrated in a real-world analysis [ 30 ]. Similar in aim to the Xpert MTB/XDR assay, these LPAs provide prompt recognition of drug resistance, so patients can be started on the appropriate regimen and further drug resistance does not have an opportunity to develop while awaiting standard culture-based susceptibility results, nor are patients exposed to burdensome, longer drug regimens with higher potential for toxicity.
Whole genome sequencing (WGS)
While NAAT and LPA tests are rapid, accessible diagnostics, their efficacy at detecting drug resistance is hindered by the inability to detect clinically relevant mutations outside the rifampicin resistance determining region (RRDR) of the rpoB gene [ 31 ]. While 95% of resistant cases arise from mutations in this region, there have been a number of public health crises emerging from missed diagnosis of outbreaks that have arisen from mutations outside it [ 32 ]. One such example is the I491F mutation that has been responsible for an outbreak of MDR-TB in Eswatini and remains a grave public health concern [ 33 ]. Another limitation is the inability to differentiate silent mutations from those that hinder drug efficacy, thus delivering a higher rate of false positive resistance results [ 34 ]. WGS provides a comprehensive review of the entire Mtb genotype with a 96% concordance for culture-based sensitivity testing [ 35 ]. It provides genotypic sensitivity to most drugs required for treatment of MDR-TB [ 36 ]. While full clarification on clinical correlation between genotypic and phenotypic sensitivities remains to be shown, progress has been made in assigning probability of pDST based on genotypic results [ 37 ]. Utility was initially limited in low-income countries by cost and requirement for robust facilities and technical expertise [ 38 ]. However, with ongoing technological advancements in the microfluidic approaches to TB diagnosis, WGS is likely to be available at point of care on a global basis [ 39 ]. For some countries, it remains an important tool not only in case diagnosis, but in formulating public health policy by assisting in tracing TB contact cases in outbreaks [ 40 ]. In the future, with improved knowledge of the genomics involved in TB resistance, WGS is likely to prove revolutionary in tailoring TB treatment to each individual patient based on the particular genome identified by the Mtb strain they have contracted.
Culture-based drug sensitivity testing (DST)
As previously mentioned, the major advantage of liquid culture is rapidity of growth, which has led to more widespread use of liquid broth-based methods such as the MGIT. BACTEC MGIT 960 is a fully automated system that delivers results within 2 weeks [ 41 ]. Culture-based DST remains the gold standard for determining drug resistance at present [ 1 ]. The two approaches currently in use are the critical concentration and minimum inhibitory concentration (MIC). Classically, critical concentration was defined as the lowest concentration of a drug that inhibits growth of 95% of Mtb strain present. Owing to ongoing research, these critical concentrations are regularly updated with a recent reduction in the critical concentration required to determine RIF resistance, allowing for greater concordance between genotypic and phenotypic sensitivity results [ 42 ]. Alternatively, the MIC method is defined as the lowest concentration of a drug that results in complete inhibition of visual growth of the Mtb strain in vitro . Following extensive work completed by national reference laboratories, and international discussion and agreement, a new reference MIC protocol has been set and validated by European consortia [ 43 ].
Computer aided detection for chest radiographs
Given the limitations, in terms of time, cost and infrastructure, to the above testing methods, it has become clear that there need to be affordable, accessible methods of screening available in high-burden areas to assist with risk stratification for allocating further testing. One such proposed method is the use of computer software to digitally interpret chest radiographs, and assign a score indicating the likelihood of TB. The most commonly studied software is CAD4TB, currently on version 6. When compared with NAAT, CAD4TB has been shown to have 90–100% sensitivity, and 23–45% specificity at detecting TB disease [ 44 ]. It performs similarly to expert clinicians and radiologists, with similar pitfalls including disease obscured by musculoskeletal findings and differentiating old scarring from new disease. Its use is intended for high-burden areas, that may lack readily accessible radiological expertise on site to interpret chest radiographs in a timely fashion [ 45 ]. It may assist peripheral health centres to determine which patients require further molecular testing.
Serum biomarkers
Another potential method for triage testing is serum biomarkers. Devising an accurate biomarker that upholds sensitivity across different ethnicities, HIV status and site of TB has proven difficult. However, a nine protein biosignature has recently been discovered which appears to remain efficacious in all of these cohorts. Using fibrinogen, α 2 -macroglobulin, C-reactive protein, matrix metalloproteinase-9, transthyretin, complement factor H, interferon-γ, interferon-γ inducible protein-10 and tumour necrosis factor-α as a host biosignature demonstrated 92% sensitivity and 72% specificity for determining TB from other diseases [ 46 ]. If available on a commercial level, this serum assay could rapidly and effectively determine which patients warranted further testing. It is important to note that most of these biomarkers are markers of inflammation, and as such are widely variable amongst patients and their differing metabolic and disease states. Evaluating serum biomarkers as predictors of response to treatment, potential for relapse and predictors of TB infection versus active disease will be discussed elsewhere.
Alongside research into obtaining accurate and timely diagnostics, there is tremendous work ongoing in developing safe, efficacious, tolerable treatment regimens. The goals of treatment are not only to eradicate disease, but to prevent long-term morbidity arising from either the disease itself or as an adverse effect of the drugs in use. Successful treatment of drug-sensitive TB (DS-TB) has been reported in 85% of patients [ 1 ]. Efficacy in drug-resistant forms is lower at 57% and is likely multifactorial [ 1 ]. To reflect this, there has been a trend towards oral drug regimens, where possible, given research highlighting patient preference and cost-effectiveness of these drugs [ 47 ]. We need to deliver a regimen that will not only aid our global goal of TB eradication, but in a manner that reflects our patients’ wishes, and in doing so, promotes their compliance.
Current treatment
The current medications approved for use in TB treatment, and their notable side-effects are summarised in table 1 .
Current medications in use for TB treatment and their notable side-effects
# : given with pyridoxine prophylaxis to ameliorate risk; ¶ : beta-lactams must be given with Clavulanate for success in TB treatment; however, the only available preparations include Amoxicillin. Information from [ 50 ].
DS-TB tends to follow a standard 6-month regime. This comprises an intensive phase with 2 months treatment consisting of RIF, INH, pyrazinamide (PZA) and ETM, followed by a continuation phase with 4 months treatment of RIF and INH [ 48 ]. If the isolate is susceptible to both RIF and INH, ETM can be stopped. The continuation phase should be extended to 7 months in the presence of: cavitation on the initial chest radiograph; persistent sputum growth at 2 months; or if PZA cannot be used due to monoresistance or drug side-effects. Consideration should also be given to extending this phase to 7 months in patients who are otherwise immunosuppressed, such as patients with HIV, diabetes mellitus, malignancy or medications associated with immunosuppression [ 48 ]. Unfavourable outcomes are most associated with high grade smear positivity (at least 3+) and dependent on the size of cavities, as well as extent of disease on chest radiographs [ 49 ].
Current treatment of drug-resistant TB is more complex and is summarised in table 2 . Most notable is the longer duration of treatment involving combinations of drugs that are often poorly tolerated. There is also minor discordance between the two major international advisory bodies (the WHO and the joint ATS/CDC/ERS/IDSA clinical practice guideline) concerning optimum drug selection and durations. While the WHO recommends only four drugs need to be used in the intensive phase of treatment, the ATS/CDC/ERS/IDSA propose continuing to use five drugs in this phase. The ATS/CDC/ERS/IDSA have proposed this recommendation based on higher success rates in the five-drug group (93.9% versus 89.7%; adjusted odds ratio (aOR) 3.0 versus 1.2; risk difference 8% in both groups). Additionally, they suggest it is likely that one of the drugs may need to be withdrawn due to toxicity [ 50 ]. However, given equivocal risk differences in both groups, the WHO maintain four drugs should be sufficient, providing susceptibilities are known and toxicity is unlikely. De-escalation to a continuation phase comprising three or four drugs is based on similar evidence. Traditionally, MDR-TB required treatment for a total duration of 15–21 months [ 50 ]. Alternatively, it does allow for a shorter 9–12 month all oral regimen for patients who have not previously had more than 1 month of treatment with second-line medications, and in whom FLQ resistance has been ruled out. Additionally, patients should not have extensive disease [ 51 ]. This shorter regimen involves 4 months of six drugs (FLQ, clofazimine (CFZ), ETH, PZA, INH (high dose)), followed by 5 months of FLQ, CFZ, ETH and PZA. BDQ is used concurrently for the first 6 months of this regimen. This conditional recommendation of low certainty evidence was proposed owing to improved success and adherence rates, when compared with shorter regimens containing injectable agents (aOR 1.9 (95% CI 1.6–2.4)) [ 52 ]. (Note, INH is used regardless of susceptibility status).
Current ATS/CDC/ERS/IDSA consolidated guidelines on treating drug-resistant TB
ATS: American Thoracic Society; CDC: US Centers For Disease Control And Prevention; ERS: European Respiratory Society; IDSA: Infectious Diseases Society of America. # : in contrast, the WHO suggests only one of these drugs are required, comprising a 4-drug regimen (see text for full details); ¶ : superscript numbers refer to the order in which the WHO suggests drugs be incorporated into regimes. Information from [ 50 ].
At present, the WHO recommends treatment for RR-TB in line with MDR-TB.
Pre-XDR- and XDR-TB are more difficult to treat, owing to varying patterns of drug resistance and advice should always be sought from national and international expert TB consortia prior to commencing treatment.
New treatment: drugs
At present, there are 16 new drugs in phase I or II clinical trials, and 22 other drugs in discovery or preclinical phases of development, as outlined in figure 1 . Of those drugs undergoing clinical trial, there are 11 drugs of new chemical classes. Of the remaining drugs, TBAJ-587 and TBAJ-876 are diarylquinolines, similar to BDQ, while delpazolid, sutezolid and TBI-223 are oxazolidinones, similar to LZD and cycloserine. At the time of publication, no new drugs have reached phase III trials or been approved for market regulation since the approval of pretomanid (Pa) in 2019. A promising candidate from a new drug class is telacebec. It induces bacterial cell death by inhibiting the mycobacterial cytochrome bc1 complex responsible for ATP synthesis. A proof-of-concept trial has shown increased rates of sputum clearance, with comparable levels of adverse events to currently approved drugs. If results from ongoing clinical trials continue to reflect this, it is likely to be approved as a third new modern drug class with anti-tuberculous activity [ 53 ]. This would be an important achievement as many of the other drugs in development are classified similarly to existing drugs, and as such their use in additive or substitutive places for their relative counterparts will be precluded due to concerns regarding toxicity or resistance. It is also interesting to note that these drugs in development are largely oral preparations, owing to patient preference and thus potential for greater adherence and cure.
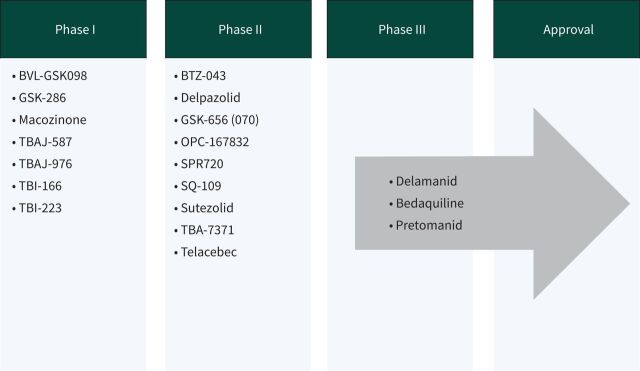
New anti-tuberculous drugs currently in development. Data from [ 104 ].
New treatment: routes of delivery
While not a novel idea, interest in inhalation routes has been re-ignited. Numerous methods of drug delivery have been shown to be effective in animals, and additional advantages include reduced dosage and systemic toxicity. However, it would likely have no benefit on extrathoracic disease, nor would it be likely to achieve adequate therapeutic serum concentrations. Similar to the use of nebulised aminoglycosides in non- Mycobacterium tuberculosis , the potential for inhalational therapies to augment TB therapy likely lies as an adjunctive therapy to oral or injectable drug regimens. To date, there has been no data published from similar trials investigating its efficacy in sputum clearance from Mtb disease.
New treatment: regimes
In a disease that has the potential to affect one quarter of the world's population, it is astounding that no advances have been made in progressing the regimen for DS-TB since the mid-20th century. At present, international consensus guidelines continue to endorse standard 6-month regimes for the majority of cases of DS-TB, with varying longer regimens requiring expert opinion for drug-resistant cases. However, much research is being done into assessing shorter regimens with the aim of improving patient adherence and reducing risk of relapse and evolution of drug resistance, as seen in table 3 .
- A shorter 4-month regimen of rifapentine (RFP) in combination with MFX has recently been shown to be non-inferior to the current standard 6-month regimen, as determined by negative smear or culture at 12 months, with no increase in major adverse events [ 54 ].
- The SimpliciTB group have evaluated a 4-month regimen comprising BDQ, Pa, MFX and PZA, in place of the standard 6-month regimen. While it has been said that no drug should ever be kept in reserve, it is unlikely that this regimen will be recommended as first-line therapy for DS-TB, given the need to preserve efficacious drug options for resistant cases [ 55 , 56 ].
- Shorter again with a 2-month regime, the TRUNCATE-TB trial is at recruitment phase. This multi-armed approach will assess combinations of 4–5 currently approved oral anti-tuberculous medications given daily for 8 weeks, with the potential to extend to 12 weeks [ 57 ].
- The RIFASHORT and ReDEFINe studies are evaluating the risk–benefit ratio of higher doses of RIF in DS-TB [ 58 , 59 ]. The evidence base for these ongoing trials has been provided by the HIRIF trial which found an increased rate of sputum clearance, with no associated increase in toxicity, in patients on higher doses of RIF than currently recommended by the WHO [ 60 ].
- • The current recommendations for RR-TB have been considered contentious for quite some time. These longer regimens likely expose patients with mono-resistance to unnecessarily long and toxic drug regimens, and also exclude the benefits of INH therapy [ 61 ]. BEAT TB is at the enrolment stage assessing the efficacy of 6 months of BDQ, LZD, delamanid (DLM), LFX and CFZ in comparison to current practices in South Africa [ 62 ].
- • The updated STREAM2 study is evaluating a shorter regimen for RR-TB and MDR-TB in a simultaneous multi-armed approach. Their four regimens are based on: current WHO practice; the Bangladesh regime; a 40-week all oral regimen; and a 28-week oral regimen after an 8 week intense regime that also involves INH and kanamycin (Kan) [ 63 , 64 ].
- • Results from the NEXT trial completed in December 2020 are awaited. This group compared 6–9 months of LZD, BDQ, LFX, PZA and ETH or INH (high dose) to current standards of care [ 65 ].
- • TB-PRACTECAL stopped early due to superior outcomes in the intervention arm, consisting of a 6-month regimen of BDQ, Pa, LZD and MFX. Full results are awaited [ 66 , 67 ].
- • SimpliciTB are also evaluating a regimen for RR-TB/MDR-TB consisting of the same drugs as the DS-TB protocol (BDQ, PZA, MFX, Pa) but for 6 months [ 56 ].
- • DELIBERATE are completing a phase II safety trial reviewing the safety and pharmacokinetics of combined BDQ and DLM therapy. Given the updated consensus guidelines, these drugs will often be given together and it is essential we have an evidence base for potential harms that may arise throughout the course of treatment [ 68 ].
- • endTB, run by Médecins Sans Frontières, are evaluating a multi-armed approach combining varying combinations of an all oral regimen for 39 weeks. Similar to the STREAM2 study, this is the only other multi-armed trial reviewing multiple combinations of novel drugs simultaneously [ 69 ].
- • The ZeNix trial is the only trial, at present, that is reviewing treatment regimens for patients with pre-XDR- or XDR-TB. Using BDQ, Pa and either LZD (BPaL) or placebo for a total duration of 26 weeks, their aim is to assess rates of sputum conversion. This trial is also one of the few to follow patients for a significant period post-treatment, and patients with be reviewed for 78 weeks following the end of treatment [ 70 ]. Data from its predecessor the Nix-TB trial has shown 88% favourable outcomes at 24 months following treatment in patients with either MDR- or XDR-TB [ 71 ]. This BPaL regime can currently be used under operational research conditions in patients with MDR-TB, in accordance with WHO guidance [ 51 ].
New drug regimens under evaluation
HD: high dose; RIF: rifampicin; INH: isoniazid; RFP: rifapentine; ETM: ethambutol; PZA: pyrazinamide; MFX: moxifloxacin; LFX: levofloxacin; LZD: linezolid; ETH: ethionamide; BDQ: bedaquiline; DLM: delamanid; Pa: pretomanid; CFZ: clofazamine; Kan: kanamycin; Pro: prothionamide; Pl: Placebo. # : ClinicalTrials.gov identifier. Information from [ 1 , 104 ].
While many of these trials demonstrate promise for an improved approach to TB treatment, it is essential that we see long-term data on their efficacy and relapse rates prior to implementing them on a global scale. The fear is that these patients may have excellent short-term results, but disease recurs soon after with the added potential for drug resistance to develop.
New treatment: adjuncts
In addition to shorter regimens, with new or re-purposed drugs, there is research into methods of modifying the host immune response to improve treatment outcomes and prevent permanent morbidity from TB disease. As previously discussed, upon infection with Mtb the host can either suppress bacillary replication into a latent state, or the host is overwhelmed and active disease develops [ 72 ]. Both deficient and hyperinflammatory states have been associated with TB disease morbidity and mortality, suggesting that tailoring a balanced immune response is of paramount importance to survival [ 73 ]. With evolving knowledge of the pathways and subcellular responses involved, new therapeutic targets are being developed to assist with bacillary quiescence in the so called “host directed therapy” approach [ 74 ]. Numerous drug targets have been suggested, largely centred on modulating macrophage activity [ 75 ]. Proposed adjunctive therapies include vitamin D, everolimus, auranofin and CC-11050, a novel anti-inflammatory compound. Preliminary results from trial data suggest none of these compounds improve rates of sputum conversion; however, patients in receipt of CC-11050 or everolimus had increased recovery of FEV 1 (forced expiratory volume in 1 s) post-treatment, perhaps solidifying the role of a balanced immune response to infection [ 76 ].
New treatment: the future
Going forward, with a combination of new drugs, altered durations and more effective testing of response to treatment, it is likely that each patient will have a tailored approach to TB treatment [ 49 ]. With studies like PredictTB, aiming to determine biomarkers and radiographic appearances that predict response and likelihood of relapse, we will be able to devise a drug combination and duration with greater specificity for each patient [ 77 ]. Similar technology may even assist with developing even more efficacious drugs in early-stage clinical trials [ 78 ]. Additionally, it is essential that any new drug or technology developed is affordable and available to all institutions, most importantly hospitals in low-resource environments, where the majority of the global TB burden persists.
Despite ongoing research, treatment for DS-TB has remained unchanged for decades. This highly effective regimen is often poorly tolerated by patients, and “drug holidays” are frequent during treatment. This, of course, increases the likelihood of relapse and evolution of drug resistance. Moreover, patients with resistant TB have to endure longer regimens with their own associated side-effects. While awaiting the development and approval of less toxic regimens, there are a number of measures we can take to ameliorate adverse effects of treatment and promote patient adherence. It has been shown that comprehensive patient-centred approaches, involving nutritional, financial and psychological support, have higher rates of completion. In addition, patients with increased contact with healthcare workers tended to have lower drop-out rates during treatment [ 79 ]. The evidence base for this is provided by systematic reviews of mostly observational case studies and case cohorts, and as such randomised research in this area is required to determine a formal link.
Directly observed therapy
Directly observed therapy (DOT) has been a standard of care in TB treatment for several years. The premise is that patients are more likely to comply if medication ingestion is witnessed multiple times per week. Current recommendations are that it should be implemented in MDR- or XDR-TB cases, or for patients with complex or vulnerable care needs, such as homelessness, comorbid psychiatric illness or addiction [ 80 ]. There have been conflicting results from systematic reviews on the efficacy of DOT [ 81 , 82 ]. What is known, is that community-based DOT appears to be the most effective strategy, as it is less disruptive for patients and thus their adherence is more likely to be maintained [ 83 ]. In recent years, attention has switched towards the use of smartphone technology. Video observed therapy (VOT) has been suggested as an even less disruptive form of monitoring adherence [ 84 ]. Patients can either upload videos of medication ingestion to a secure platform to be watched at a later date, or it can be taken while on a live feed with their healthcare team. VOT has been shown to have a higher uptake rate and patient preference rating [ 85 ]. While plausible that this will improve adherence, and thus relapse should be less likely, this study was not sufficiently powered to assess this, nor did it follow up on relapse rates at an appropriate interval. A real-world efficacy and cost-effectiveness study is ongoing in a tertiary hospital in Ireland at present [ 86 ].
Prophylaxis
Undoubtedly, a burden of TB infection will persist for years to come. However, we have a chance to prevent many of these patients from progressing to active disease. Screening for TB infection in groups at high risk of progressing to TB disease remains a cost-effective and essential component to the global initiative. Screening via either of the endorsed interferon-γ release assays (QuantiFERON-TB Gold In-Tube and T-SPOT.TB) or traditional tuberculin skin testing is recommended in certain populations. The WHO has advised that clinical judgement is paramount in interpreting these tests, and cautions that a higher rate of false negatives occurs in the most vulnerable populations [ 87 ]. Another essential component of the sustainable development goals is robust public health policy to assist in contact tracing of index cases and early treatment of contacts. In addition, prior to any prophylactic treatment being commenced, it is essential that due caution is taken to rule out the presence of active TB disease.
Currently the WHO advocates for treatment with 4 months of RIF or 6–9 months of INH in cases where the index case is known to be drug sensitive [ 87 ]. A 3-month combination of RIF and INH is also approved, although rarely used due to potential toxicity. Additionally, weekly INH and RFP for 3 months has been shown to demonstrate equal efficacy and toxicity in comparison to 6 months of INH therapy, while higher levels of adherence were noted in the INH/RFP arm [ 88 ]. Moreover, a 1-month regimen of RFP/INH therapy was non-inferior to 9 months INH monotherapy in preventing TB in HIV-infected patients [ 89 ]. However, this regimen has yet to be endorsed by major international consortia.
The recommendations for TB contacts of DS-TB cases who demonstrate evidence of TB infection are as per those above. For contacts of MDR-TB cases, the current recommendation is for 6–12 months treatment with a FLQ with or without a second drug. If a FLQ cannot be used due to resistance in the index case, treatment with ETM and PZA is to be considered [ 87 ]. Regardless of the regimen in use, it is vital that strict adherence is maintained to ensure efficacy and prevent resistance.
At present, the decision to treat is based on the potential for progression to active disease based on similar case profiles. Going forward, we could vastly improve the cost efficacy of this intervention by being able to determine exactly which patients were going to progress to active TB disease or not. It had been hoped the answer would lie in serum transcriptional biomarkers and host response-based gene signatures [ 90 , 91 ]. Recently, a four-protein biomarker panel has shown 67.3% sensitivity and 96.3% specificity at determining active from latent TB [ 92 ]. This subclinical phase of TB disease can be difficult to interpret due to its lower inflammatory profile and person specific confounding factors that influence our immune response. Recent results from transcriptomic studies have been disappointing overall, but may potentially suggest a role for these panels in symptomatic patients with known TB infection and their risk of progression to TB disease in an imminent 6-month period [ 93 ].
Vaccination
Given the current prevalence of TB infection, with the associated lifetime risk of progressing to active disease, it is paramount that we protect future generations from this burden by halting transmission entirely. With greater understanding of the cellular processes involved in Mtb susceptibility and pathogenesis, scientists have been able to identify various potential targets with a role in vaccination. Central to this is the cellular immune response, with a need to upregulate T-helper cell (Th)1, and downregulate Th2 and regulatory T-cell responses [ 94 ]. It appears that Mtb has also recognised the need to adapt to this hypo-inflammatory phenotype with more modern strains displaying shorter latency and higher virulence than previously seen [ 95 ].
The only worldwide approved vaccine against TB remains bacillus Calmette–Guérin (BCG), effectively reducing the risk of severe childhood disease from TB, with an 85% reduction in TB meningitis and miliary TB in those <10 years of age [ 96 ]. It has also been noted that infants innoculated with BCG have increased survival and lower rates of other childhood infections. This observation is likely secondary to BCG's ability to prime innate immunity through epigenetic modification of innate immune cells [ 97 ].
Vaccination can be categorised into preventive pre-exposure, preventive post-exposure or therapeutic [ 98 ]. Vaccines can alternatively be classified according to their biochemical forms: live attenuated, inactivated, protein subunit or recombinant [ 99 ]. With each of these forms, the aim is to target various cells or subcellular components of TB pathogenesis.
MTBVAC, a pre-exposure live attenuated vaccine, has shown promising results from preclinical trials with a higher protection against TB than BCG [ 100 ]. This live vaccine is based on a genetically modified mutant Mtb strain containing deletions in transcription factors important for Mtb growth in macrophages and subsequent virulence.
VPM1002, another live recombinant BCG vaccine, is undergoing phase III studies at present to evaluate its efficacy at not only preventing infection, but in preventing active disease in those already affected [ 101 ]. This vaccine can modify T-cell immune response and enhance Th1 immunity, important in TB disease pathogenesis.
Another promising post-exposure candidate is M72/AS01E, a subunit vaccine, that prevents pulmonary TB in adults already infected with Mtb in 54% of patients, and thus could be a potentially life-saving intervention for one quarter of the world's population [ 102 ]. Also known as Mtb72F this vaccine comprises two immunogenic proteins that promote T-cell proliferation and interferon-γ release [ 103 ].
Further randomised control trials are warranted in a timely manner if the END TB strategy is to be achieved.
The future is bright for TB treatment. Never before has there been such a global effort to develop new technologies and treatment for TB patients. Combining these advancements, it is possible that we will base each patient's treatment on their own protein biosignatures in conjunction with the genomic expression of mutations in the Mtb strain they have been affected with. If we are to achieve our goal of global eradication of TB, it is essential that we continue to collaborate and share our expertise on an international scale to ensure each patient gets the appropriate treatment and support to overcome their TB diagnosis without significant morbidity.
Self- evaluation questions
- b) Biomolecular test ( e.g. Xpert MTB/RIF or Truenat MTB)
- c) Line probe assay ( e.g. Genotype MTBDR plus )
- d) Serum interferon-γ release assay
- a) Linezolid
- b) Second-line injectable drugs ( e.g. amikacin)
- c) Fluoroquinolones ( e.g. moxifloxacin)
- d) Bedaquiline
- Rifampicin (10 mg·kg −1 up to 600 mg maximum) daily for 4 months
- Isoniazid (5 mg·kg −1 up to 300 mg maximum) daily for 6–9 months
- Rifampicin (10 mg·kg −1 up to 600 mg maximum) and Isoniazid (5 mg·kg −1 up to 300 mg maximum) daily for 3 months
- Rifapentine (900 mg if weight >50 kg, adjusted if less) and Isoniazid (15 mg·kg −1 up to 900 mg maximum) weekly for 1 month
Suggested answers
Conflict of interest: C.M. Gill has nothing to disclose.
Conflict of interest: L. Dolan has nothing to disclose.
Conflict of interest: L.M. Piggott has nothing to disclose.
Conflict of interest: A.M. McLaughlin has nothing to disclose.
- 1. World Health Organisation . Global tuberculosis report 2020. Geneva, World Health Organisation, 2020. [ Google Scholar ]
- 2. World Health Organisation . WHO consolidated guidelines on tuberculosis: tuberculosis preventive treatment: Module 1: prevention. Geneva, World Health Organisation, 2020. [ PubMed ] [ Google Scholar ]
- 3. Selwyn PA, Hartel D, Lewis VA, et al. . A prospective study of the risk of tuberculosis among intravenous drug users with human immunodeficiency virus infection. N Engl J Med 1989; 320: 545–550. doi: 10.1056/NEJM198903023200901 [ DOI ] [ PubMed ] [ Google Scholar ]
- 4. Uplekar M, Weil D, Lonnroth K, et al. . WHO's new end TB strategy. Lancet 2015; 385: 1799–1801. doi: 10.1016/S0140-6736(15)60570-0 [ DOI ] [ PubMed ] [ Google Scholar ]
- 5. Centers for Disease Control and Prevention . World Tuberculosis Day. 2021. www.cdc.gov/tb/features/wtbd/2021WTBD_Feature.html Date last updated: 4 March 2021.
- 6. World Health Organisation . WHO announces updated definitions of extensively drug-resistant tuberculosis. 2021. https://www.who.int/news/item/27-01-2021-who-announces-updated-definitions-of-extensively-drug-resistant-tuberculosis Date last updated: 27 January 2021.
- 7. Asmar S, Drancourt M. Rapid culture-based diagnosis of pulmonary tuberculosis in developed and developing countries. Front Microbiol 2015; 6: 1184. doi: 10.3389/fmicb.2015.01184 [ DOI ] [ PMC free article ] [ PubMed ] [ Google Scholar ]
- 8. World Health Organisation . Policy framework for implementing new tuberculosis diagnostics. Geneva, World Health Organisation, 2010. [ Google Scholar ]
- 9. Horne DJ, Royce SE, Gooze L, et al. . Sputum monitoring during tuberculosis treatment for predicting outcome: systematic review and meta-analysis. Lancet Infect Dis 2010; 10: 387–394. doi: 10.1016/S1473-3099(10)70071-2 [ DOI ] [ PMC free article ] [ PubMed ] [ Google Scholar ]
- 10. Sulis G, Centis R, Sotgiu G, et al. . Recent developments in the diagnosis and management of tuberculosis. NPJ Prim Care Respir Med 2016; 26: 16078. doi: 10.1038/npjpcrm.2016.78 [ DOI ] [ PMC free article ] [ PubMed ] [ Google Scholar ]
- 11. Bhalla M, Sidiq Z, Sharma PP, et al. . Performance of light-emitting diode fluorescence microscope for diagnosis of tuberculosis. Int J Mycobacteriol 2013; 2: 174–178. doi: 10.1016/j.ijmyco.2013.05.001 [ DOI ] [ PubMed ] [ Google Scholar ]
- 12. Deng Y, Duan Y-F, Ga S-P, et al. . Comparison of LAMP, GeneXpert, mycobacterial culture, smear microscopy, TSPOT.TB, TBAg/PHA ratio for diagnosis of pulmonary tuberculosis. Curr Med Sci 2021; 41: 1023–1028. doi: 10.1007/s11596-021-2404-4 [ DOI ] [ PMC free article ] [ PubMed ] [ Google Scholar ]
- 13. Singhal R, Myneedu VP. Microscopy as a diagnostic tool in pulmonary tuberculosis. Int J Mycobacteriol 2015; 4: 1–6. doi: 10.1016/j.ijmyco.2014.12.006 [ DOI ] [ PubMed ] [ Google Scholar ]
- 14. Gilpin C, Kim SJ, Lumb R, et al. . Critical appraisal of current recommendations and practices for tuberculosis sputum smear microscopy. Int J Tuberc Lung Dis 2007; 11: 946–952. [ PubMed ] [ Google Scholar ]
- 15. Lawn SD, Mwaba P, Bates M, et al. . Advances in tuberculosis diagnostics: the Xpert MTB/RIF assay and future prospects for a point-of-care test. Lancet Infect Dis 2013; 13: 349–361. doi: 10.1016/S1473-3099(13)70008-2 [ DOI ] [ PMC free article ] [ PubMed ] [ Google Scholar ]
- 16. Steingart KR, Schiller I, Horne DJ, et al. . Xpert® MTB/RIF assay for pulmonary tuberculosis and rifampicin resistance in adults. Cochrane Database Syst Rev 2014; 1: CD009593. Doi: 10.1002/14651858.CD009593.pub3 [ DOI ] [ PMC free article ] [ PubMed ] [ Google Scholar ]
- 17. Dorman SE, Schumacher SG, Alland D, et al. . Xpert MTB/RIF Ultra for detection of Mycobacterium tuberculosis and rifampicin resistance: a prospective multicentre diagnostic accuracy study. Lancet Infect Dis 2018; 18: 76–84. doi: 10.1016/S1473-3099(17)30691-6 [ DOI ] [ PMC free article ] [ PubMed ] [ Google Scholar ]
- 18. Arend SM, van Soolingen D. Performance of Xpert MTB/RIF Ultra: a matter of dead or alive. Lancet Infect Dis 2018; 18: 8–10. doi: 10.1016/S1473-3099(17)30695-3 [ DOI ] [ PubMed ] [ Google Scholar ]
- 19. Park M, Kon OM. Use of Xpert MTB/RIF and Xpert Ultra in extrapulmonary tuberculosis. Expert Rev Anti Infect Ther 2021; 19: 65–77. doi: 10.1080/14787210.2020.1810565 [ DOI ] [ PubMed ] [ Google Scholar ]
- 20. Sohn H, Kasaie P, Kendall E, et al. . Informing decision-making for universal access to quality tuberculosis diagnosis in India: an economic-epidemiological model. BMC Med 2019; 17: 155. doi: 10.1186/s12916-019-1384-8 [ DOI ] [ PMC free article ] [ PubMed ] [ Google Scholar ]
- 21. Cao Y, Parmar H, Gaur RL, et al. . Xpert MTB/XDR: a 10-color reflex assay suitable for point-of-care settings to detect isoniazid, fluoroquinolone, and second-line-injectable-drug resistance directly from Mycobacterium tuberculosis -positive sputum. J Clin Microbiol 2021; 59: e02314-20. doi: 10.1128/JCM.02314-20 [ DOI ] [ PMC free article ] [ PubMed ] [ Google Scholar ]
- 22. Bjerrum S, Schiller I, Dendukuri N, et al. . Lateral flow urine lipoarabinomannan assay for detecting active tuberculosis in people living with HIV. Cochrane Database Syst Rev 2019; 10: CD011420. doi: 10.1002/14651858.CD011420.pub3 [ DOI ] [ PMC free article ] [ PubMed ] [ Google Scholar ]
- 23. Engel N, Mwaura M. User perspectives on LF-LAM for the diagnosis of active tuberculosis: results from qualitative research. In: Lateral flow urine lipoarabinomannan assay (LF-LAM) for the diagnosis of active tuberculosis in people living with HIV: policy update (2019). Geneva, World Health Organistaion, 2019. [ Google Scholar ]
- 24. World Health Organisation . The use of molecular line probe assays for the detection of resistance to isoniazid and rifampicin: policy update. Geneva, World Health Organisation, 2016. [ Google Scholar ]
- 25. Muller B, Streicher EM, Hoek KG, et al. . inhA promoter mutations: a gateway to extensively drug-resistant tuberculosis in South Africa? Int J Tuberc Lung Dis 2011; 15: 344–351. [ PubMed ] [ Google Scholar ]
- 26. Somoskovi A, Dormandy J, Rivenburg J, et al. . Direct comparison of the genotype MTBC and genomic deletion assays in terms of ability to distinguish between members of the Mycobacterium tuberculosis complex in clinical isolates and in clinical specimens. J Clin Microbiol 2008; 46: 1854–1857. doi: 10.1128/JCM.00105-07 [ DOI ] [ PMC free article ] [ PubMed ] [ Google Scholar ]
- 27. Huang WL, Chen HY, Kuo YM, et al. . Performance assessment of the GenoType MTBDRplus test and DNA sequencing in detection of multidrug-resistant Mycobacterium tuberculosis . J Clin Microbiol 2009; 47: 2520–2524. doi: 10.1128/JCM.02499-08 [ DOI ] [ PMC free article ] [ PubMed ] [ Google Scholar ]
- 28. HAIN LifeScience . Genotype MTBDRslVER 2.0 instructions for use. Document IFA-317A-01. Nehren, HAIN LifeScience, 2015. [ Google Scholar ]
- 29. Gardee Y, Dreyer AW, Koornhof HJ, et al. . Evaluation of the GenoType MTBDRsl Version 2.0 assay for second-line drug resistance detection of Mycobacterium tuberculosis isolates in South Africa. J Clin Microbiol 2017; 55: 791–800. doi: 10.1128/JCM.01865-16 [ DOI ] [ PMC free article ] [ PubMed ] [ Google Scholar ]
- 30. Javed H, Bakuła Z, Pleń M, et al. . Evaluation of genotype MTBDR plus and MTBDR sl assays for rapid detection of drug resistance in extensively drug-resistant Mycobacterium tuberculosis isolates in Pakistan. Front Microbiol 2018; 9: 2265. doi: 10.3389/fmicb.2018.02265 [ DOI ] [ PMC free article ] [ PubMed ] [ Google Scholar ]
- 31. Siu GK, Zhang Y, Lau TC, et al. . Mutations outside the rifampicin resistance-determining region associated with rifampicin resistance in Mycobacterium tuberculosis . J Antimicrob Chemother 2011; 66: 730–733. doi: 10.1093/jac/dkq519 [ DOI ] [ PubMed ] [ Google Scholar ]
- 32. Zaw MT, Emran NA, Lin Z. Mutations inside rifampicin-resistance determining region of rpoB gene associated with rifampicin-resistance in Mycobacterium tuberculosis . J Infect Public Health 2018; 11: 605–610. doi: 10.1016/j.jiph.2018.04.005 [ DOI ] [ PubMed ] [ Google Scholar ]
- 33. Sanchez-Padilla E, Merker M, Beckert P, et al. . Detection of drug-resistant tuberculosis by Xpert MTB/RIF in Swaziland. N Engl J Med 2015; 372: 1181–1182. doi: 10.1056/NEJMc1413930 [ DOI ] [ PubMed ] [ Google Scholar ]
- 34. Alonso M, Palacios JJ, Herranz M, et al. . Isolation of Mycobacterium tuberculosis strains with a silent mutation in rpoB leading to potential misassignment of resistance category. J Clin Microbiol 2011; 49: 2688–2690. doi: 10.1128/JCM.00659-11 [ DOI ] [ PMC free article ] [ PubMed ] [ Google Scholar ]
- 35. Nikolayevskyy V, Kranzer K, Niemann S, et al. . Whole genome sequencing of Mycobacterium tuberculosis for detection of recent transmission and tracing outbreaks: A systematic review. Tuberculosis (Edinb) 2016; 98: 77–85. doi: 10.1016/j.tube.2016.02.009 [ DOI ] [ PubMed ] [ Google Scholar ]
- 36. Coll F, McNerney R, Preston MD, et al. . Rapid determination of anti-tuberculosis drug resistance from whole-genome sequences. Genome Med 2015; 7: 51. doi: 10.1186/s13073-015-0164-0 [ DOI ] [ PMC free article ] [ PubMed ] [ Google Scholar ]
- 37. CRyPTIC Consortium and the 100,000 Genomes Project , Allix-Béguec C, Arandjelovic I, et al. . Prediction of susceptibility to first-line tuberculosis drugs by DNA sequencing. N Engl J Med 2018; 379: 1403–1415. doi: 10.1056/NEJMoa1800474 [ DOI ] [ PMC free article ] [ PubMed ] [ Google Scholar ]
- 38. Eddabra R, Ait Benhassou H. Rapid molecular assays for detection of tuberculosis. Pneumonia (Nathan) 2018; 10: 4. doi: 10.1186/s41479-018-0049-2 [ DOI ] [ PMC free article ] [ PubMed ] [ Google Scholar ]
- 39. Molloy A, Harrison J, McGrath JS, et al. . Microfluidics as a novel technique for tuberculosis: from diagnostics to drug discovery. Microorganisms 2021; 9: 2330. doi: 10.3390/microorganisms9112330 [ DOI ] [ PMC free article ] [ PubMed ] [ Google Scholar ]
- 40. Roycroft E, Fitzgibbon MM, Kelly DM, et al. . The largest prison outbreak of TB in Western Europe investigated using whole-genome sequencing. Int J Tuberc Lung Dis 2021; 25: 491–497. doi: 10.5588/ijtld.21.0033 [ DOI ] [ PubMed ] [ Google Scholar ]
- 41. Tortoli E, Cichero P, Piersimoni C, et al. . Use of BACTEC MGIT 960 for recovery of mycobacteria from clinical specimens: multicenter study. J Clin Microbiol 1999; 37: 3578–3582. doi: 10.1128/JCM.37.11.3578-3582.1999 [ DOI ] [ PMC free article ] [ PubMed ] [ Google Scholar ]
- 42. World Health Organisation . Technical Report on critical concentrations for drug susceptibility testing of isoniazid and the rifamycins (rifampicin, rifabutin and rifapentine). Geneva, World Health Organisation, 2021. [ Google Scholar ]
- 43. Schon T, Werngren J, Machado D, et al. . Multicentre testing of the EUCAST broth microdilution reference method for MIC determination on Mycobacterium tuberculosis . Clin Microbiol Infect 2021; 27: 288 e1–288 e4. doi: 10.1016/j.cmi.2020.10.019 [ DOI ] [ PubMed ] [ Google Scholar ]
- 44. Harris M, Qi A, Jeagal L, et al. . A systematic review of the diagnostic accuracy of artificial intelligence-based computer programs to analyze chest x-rays for pulmonary tuberculosis. PLoS One 2019; 14: e0221339. doi: 10.1371/journal.pone.0221339 [ DOI ] [ PMC free article ] [ PubMed ] [ Google Scholar ]
- 45. Murphy K, Habib SS, Zaidi SMA, et al. . Computer aided detection of tuberculosis on chest radiographs: An evaluation of the CAD4TB v6 system. Sci Rep 2020; 10: 5492. doi: 10.1038/s41598-020-62148-y [ DOI ] [ PMC free article ] [ PubMed ] [ Google Scholar ]
- 46. Morris TC, Hoggart CJ, Chegou NN, et al. . Evaluation of host serum protein biomarkers of tuberculosis in sub-Saharan Africa. Front Immunol 2021; 12: 639174. doi: 10.3389/fimmu.2021.639174 [ DOI ] [ PMC free article ] [ PubMed ] [ Google Scholar ]
- 47. Migliori GB, Tiberi S, Zumla A, et al. . MDR/XDR-TB management of patients and contacts: challenges facing the new decade. The 2020 clinical update by the Global Tuberculosis Network. Int J Infect Dis 2020; 92S: S15–S25. doi: 10.1016/j.ijid.2020.01.042 [ DOI ] [ PubMed ] [ Google Scholar ]
- 48. Nahid P, Dorman SE, Alipanah N, et al. . Official American Thoracic Society/Centers for Disease Control and Prevention/Infectious Diseases Society of America Clinical Practice Guidelines: Treatment of Drug-Susceptible Tuberculosis. Clin Infect Dis 2016; 63: e147–e195. doi: 10.1093/cid/ciw376 [ DOI ] [ PMC free article ] [ PubMed ] [ Google Scholar ]
- 49. Imperial MZ, Nahid P, Phillips PPJ, et al. . A patient-level pooled analysis of treatment-shortening regimens for drug-susceptible pulmonary tuberculosis. Nat Med 2018; 24: 1708–1715. doi: 10.1038/s41591-018-0224-2 [ DOI ] [ PMC free article ] [ PubMed ] [ Google Scholar ]
- 50. Nahid P, Mase SR, Migliori GB, et al. . Treatment of drug-resistant tuberculosis. An official ATS/CDC/ERS/IDSA clinical practice guideline. Am J Respir Crit Care Med 2019; 200: e93–e142. doi: 10.1164/rccm.201909-1874ST [ DOI ] [ PMC free article ] [ PubMed ] [ Google Scholar ]
- 51. World Health Organisation . WHO consolidated guidelines on tuberculosis: Module 4: Treatment - Drug-resistant tuberculosis treatment. Geneva, World Health Organisation, 2020. [ PubMed ] [ Google Scholar ]
- 52. Mirzayev F, Viney K, Linh NN, et al. . World Health Organization recommendations on the treatment of drug-resistant tuberculosis, 2020 update. Eur Respir J 2021; 57: 2003300. doi: 10.1183/13993003.03300-2020 [ DOI ] [ PMC free article ] [ PubMed ] [ Google Scholar ]
- 53. de Jager VR, Dawson R, van Niekerk C, et al. . Telacebec (Q203), a new antituberculosis agent. N Engl J Med 2020; 382: 1280–1281. doi: 10.1056/NEJMc1913327 [ DOI ] [ PubMed ] [ Google Scholar ]
- 54. Dorman SE, Nahid P, Kurbatova EV, et al. . Four-month rifapentine regimens with or without moxifloxacin for tuberculosis. N Engl J Med 2021; 384: 1705–1718. doi: 10.1056/NEJMoa2033400 [ DOI ] [ PMC free article ] [ PubMed ] [ Google Scholar ]
- 55. Prasad R, Gupta N, Banka A. Multidrug-resistant tuberculosis/rifampicin-resistant tuberculosis: Principles of management. Lung India 2018; 35: 78–81. doi: 10.4103/lungindia.lungindia_98_17 [ DOI ] [ PMC free article ] [ PubMed ] [ Google Scholar ]
- 56. ClinicalTrials.Gov . Trial to evaluate the efficacy, safety and tolerability of BPaMZ in drug sensitive (DS-TB) adult patients and drug resistant (DR-TB) adult patients. https://clinicaltrials.gov/ct2/show/NCT03338621
- 57. ClinicalTrials.Gov . Two-month regimens using novel combinations to augment treatment effectiveness for drug-sensitive tuberculosis (TRUNCATE-TB). https://ClinicalTrials.gov/show/NCT03474198
- 58. ClinicalTrials.Gov . A randomised trial to evaluate toxicity and efficacy of 1200 mg and 1800mg of rifampicin for pulmonary tuberculosis (RIFASHORT). https://clinicaltrials.gov/ct2/show/NCT02581527
- 59. ClinicalTrials.Gov . High-dose rifampicin for the treatment of tuberculous meningitis: a dose-finding study (ReDEFINe). https://clinicaltrials.gov/ct2/show/NCT02169882
- 60. Velasquez GE, Brooks MB, Coit JM, et al. . Efficacy and safety of high-dose rifampin in pulmonary tuberculosis. a randomized controlled trial. Am J Respir Crit Care Med 2018; 198: 657–666. doi: 10.1164/rccm.201712-2524OC [ DOI ] [ PMC free article ] [ PubMed ] [ Google Scholar ]
- 61. Malenfant JH, Brewer TF. Rifampicin mono-resistant tuberculosis-a review of an uncommon but growing challenge for global tuberculosis control. Open Forum Infect Dis 2021; 8: ofab018. doi: 10.1093/ofid/ofab018 [ DOI ] [ PMC free article ] [ PubMed ] [ Google Scholar ]
- 62. ClinicalTrials.Gov . Building evidence for advancing new treatment for rifampicin resistant tuberculosis (RR-TB) comparing a short course of treatment (containing bedaquiline, delamanid and linezolid) with the current South African standard of care. https://clinicaltrials.gov/ct2/show/NCT04062201
- 63. ClinicalTrials.Gov . The evaluation of a standard treatment regimen of anti-tuberculosis drugs for patients with MDR-TB (STREAM). https://clinicaltrials.gov/ct2/show/NCT02409290
- 64. Van Deun A, Maug AK, Salim MA, et al. . Short, highly effective, and inexpensive standardized treatment of multidrug-resistant tuberculosis. Am J Respir Crit Care Med 2010; 182: 684–692. doi: 10.1164/rccm.201001-0077OC [ DOI ] [ PubMed ] [ Google Scholar ]
- 65. ClinicalTrials.Gov . An open-label RCT to evaluate a new treatment for patients with multi-drug resistant tuberculosis (NExT). https://clinicaltrials.gov/ct2/show/NCT02454205
- 66. ClinicalTrials.Gov . Pragmatic clinical trial for a more effective concise and less toxic MDR-TB treatment regimen(s) (TB-PRACTECAL). https://clinicaltrials.gov/ct2/show/NCT02589782
- 67. Medecins San Frontieres . Drug-resistant TB clinical trial ends enrolment early after positive initial data. https://msf.org.uk/article/drug-resistant-tb-clinical-trial-ends-enrolment-early-after-positive-initial-data Date last updated: 24 March 2021.
- 68. ClinicalTrials.Gov . Evaluating the safety, tolerability and pharmacokinetics of bedaqeuiline and delamanid, alone and in combination, for drug-resistant pulmonary tuberculosis. https://clinicaltrials.gov/ct2/show/record/NCT02583048
- 69. ClinicalTrials.Gov . Evaluating newly approved drugs for multidrug-resistant TB (endTB). https://clinicaltrials.gov/ct2/show/NCT02754765 .
- 70. ClinicalTrials.Gov . Safety and efficacy of various doses and treatment durations of linezolid plus bedaquiline and pretomanid in participants with pulmonary XDR-TB, Pre-XDR-TB or Non-responsive/Intolerant MDR-TB (ZeNix). https://clinicaltrials.gov/ct2/show/NCT03086486
- 71. Conradie F, Diacon A, Ngubane N, et al. . Final results of the NIX-TB clinical study of BPaL regimen for highly resistant TB. In: Virtual CROI 2021 Conference on Retroviruses and Opportunistic Infections. 2021. www.croiconference.org/abstract/final-results-of-the-nix-tb-clinical-study-of-bpal-regimen-for-highly-resistant-tb/
- 72. Lee SH. Tuberculosis infection and latent tuberculosis. Tuberc Respir Dis (Seoul) 2016; 79: 201–206. doi: 10.4046/trd.2016.79.4.201 [ DOI ] [ PMC free article ] [ PubMed ] [ Google Scholar ]
- 73. Tobin DM, Roca FJ, Oh SF, et al. . Host genotype-specific therapies can optimize the inflammatory response to mycobacterial infections. Cell 2012; 148: 434–446. doi: 10.1016/j.cell.2011.12.023 [ DOI ] [ PMC free article ] [ PubMed ] [ Google Scholar ]
- 74. Palucci I, Delogu G. Host directed therapies for tuberculosis: futures strategies for an ancient disease. Chemotherapy 2018; 63: 172–180. doi: 10.1159/000490478 [ DOI ] [ PubMed ] [ Google Scholar ]
- 75. Roca FJ, Whitworth LJ, Redmond S, et al. . TNF induces pathogenic programmed macrophage necrosis in tuberculosis through a mitochondrial-lysosomal-endoplasmic reticulum circuit. Cell 2019; 178: 1344–1361.e11. doi: 10.1016/j.cell.2019.08.004 [ DOI ] [ PMC free article ] [ PubMed ] [ Google Scholar ]
- 76. Wallis RS, Ginindza S, Beattie T, et al. . Adjunctive host-directed therapies for pulmonary tuberculosis: a prospective, open-label, phase 2, randomised controlled trial. Lancet Respir Med 2021; 9: 897–908. doi: 10.1016/S2213-2600(20)30448-3 [ DOI ] [ PMC free article ] [ PubMed ] [ Google Scholar ]
- 77. ClinicalTrials.Gov . Using biomarkers to predict TB treatment duration. https://clinicaltrials.gov/ct2/show/NCT02821832
- 78. ClinicalTrials.Gov . NexGen EBA radiologic and immunologic biomarkers of sterilizing drug activity in tuberculosis. https://clinicaltrials.gov/ct2/show/NCT02371681
- 79. Law S, Daftary A, O'Donnell M, et al. . Interventions to improve retention-in-care and treatment adherence among patients with drug-resistant tuberculosis: a systematic review. Eur Respir J 2019; 53: 1801030. doi: 10.1183/13993003.01030-2018 [ DOI ] [ PubMed ] [ Google Scholar ]
- 80. National Institute of Health and Care Excellence . NICE Guideline: Tuberculosis. 2016. https://www.nice.org.uk/guidance/ng33 Published: 13 January 2016. Date last updated: 12 September 2019
- 81. Volmink J, Garner P. Directly observed therapy for treating tuberculosis. Cochrane Database Syst Rev 2007; 4: CD003343. doi: 10.1002/14651858.CD003343.pub3 [ DOI ] [ PubMed ] [ Google Scholar ]
- 82. Tian JH, Lu ZX, Bachmann MO, et al. . Effectiveness of directly observed treatment of tuberculosis: a systematic review of controlled studies. Int J Tuberc Lung Dis 2014; 18: 1092–1098. doi: 10.5588/ijtld.13.0867 [ DOI ] [ PubMed ] [ Google Scholar ]
- 83. Parums DV. Editorial: updates from the World Health Organization (WHO) on global treatment recommendations for drug-susceptible and multidrug-resistant tuberculosis. Med Sci Monit 2021; 27: e934292. [ DOI ] [ PMC free article ] [ PubMed ] [ Google Scholar ]
- 84. Story A, Garfein RS, Hayward A, et al. . Monitoring therapy compliance of tuberculosis patients by using video-enabled electronic devices. Emerg Infect Dis 2016; 22: 538–540. doi: 10.3201/eid2203.151620 [ DOI ] [ PMC free article ] [ PubMed ] [ Google Scholar ]
- 85. Story A, Aldridge RW, Smith CM, et al. . Smartphone-enabled video-observed versus directly observed treatment for tuberculosis: a multicentre, analyst-blinded, randomised, controlled superiority trial. Lancet 2019; 393: 1216–1224. doi: 10.1016/S0140-6736(18)32993-3 [ DOI ] [ PMC free article ] [ PubMed ] [ Google Scholar ]
- 86. Health Innovation Hub Ireland . St James’ Hospital recruits patients with TB for Video Direct Observational Therapy study to investigate smartphone versus community care, in an Irish research first. https://hih.ie/st-james-hospital-recruits-patients-with-tb-for-video-direct-observational-therapy-study-to-investigate-smartphone-versus-community-care-in-an-irish-research-first/ Date last accessed 11 September 2021. Date last updated: 29 October, 2020.
- 87. World Health Organisation . Latent tuberculosis infection: updated and consolidated guidelines for programmatic management. Geneva, World Health Organisation, 2018. [ PubMed ] [ Google Scholar ]
- 88. Sterling TR, Villarino ME, Borisov AS, et al. . Three months of rifapentine and isoniazid for latent tuberculosis infection. N Engl J Med 2011; 365: 2155–2166. doi: 10.1056/NEJMoa1104875 [ DOI ] [ PubMed ] [ Google Scholar ]
- 89. Chaisson RE, Ramchandani R, Swindells S. One month of rifapentine plus isoniazid to prevent HIV-related tuberculosis. reply. N Engl J Med 2019; 381: e23. doi: 10.1056/NEJMc1908492 [ DOI ] [ PubMed ] [ Google Scholar ]
- 90. Warsinske H, Vashisht R, Khatri P. Host–response-based gene signatures for tuberculosis diagnosis: a systematic comparison of 16 signatures. PLoS Med 2019; 16: e1002786. doi: 10.1371/journal.pmed.1002786 [ DOI ] [ PMC free article ] [ PubMed ] [ Google Scholar ]
- 91. Esmail H, Cobelens F, Goletti D. Transcriptional biomarkers for predicting development of tuberculosis: progress and clinical considerations. Eur Respir J 2020; 55: 1901957. doi: 10.1183/13993003.01957-2019 [ DOI ] [ PMC free article ] [ PubMed ] [ Google Scholar ]
- 92. Li Z, Hu J, Liu P, et al. . Microarray-based selection of a serum biomarker panel that can discriminate between latent and active pulmonary TB. Sci Rep 2021; 11: 14516. doi: 10.1038/s41598-021-93893-3 [ DOI ] [ PMC free article ] [ PubMed ] [ Google Scholar ]
- 93. Scriba TJ, Fiore-Gartland A, Penn-Nicholson A, et al. . Biomarker-guided tuberculosis preventive therapy (CORTIS): a randomised controlled trial. Lancet Infect Dis 2021; 21: 354–365. doi: 10.1016/S1473-3099(20)30914-2 [ DOI ] [ PMC free article ] [ PubMed ] [ Google Scholar ]
- 94. Gong W, Liang Y, Wu X. The current status, challenges, and future developments of new tuberculosis vaccines. Hum Vaccin Immunother 2018; 14: 1697–1716. doi: 10.1080/21645515.2018.1458806 [ DOI ] [ PMC free article ] [ PubMed ] [ Google Scholar ]
- 95. Gagneux S. Host-pathogen coevolution in human tuberculosis. Philos Trans R Soc Lond B Biol Sci 2012; 367: 850–859. doi: 10.1098/rstb.2011.0316 [ DOI ] [ PMC free article ] [ PubMed ] [ Google Scholar ]
- 96. Mangtani P, Abubakar I, Ariti C, et al. . Protection by BCG vaccine against tuberculosis: a systematic review of randomized controlled trials. Clin Infect Dis 2014; 58: 470–480. doi: 10.1093/cid/cit790 [ DOI ] [ PubMed ] [ Google Scholar ]
- 97. Kleinnijenhuis, J, Quintin J, Preijers F, et al. . Bacille Calmette-Guerin induces NOD2-dependent nonspecific protection from reinfection via epigenetic reprogramming of monocytes. Proc Natl Acad Sci USA 2012; 109: 17537–17542. doi: 10.1073/pnas.1202870109 [ DOI ] [ PMC free article ] [ PubMed ] [ Google Scholar ]
- 98. Fatima S, Kumari A, Das G, et al. . Tuberculosis vaccine: a journey from BCG to present. Life Sci 2020; 252: 117594. doi: 10.1016/j.lfs.2020.117594 [ DOI ] [ PubMed ] [ Google Scholar ]
- 99. Whitlow E, Mustafa AS, Hanif SNM. An overview of the development of new vaccines for tuberculosis. Vaccines (Basel) 2020; 8: 586. doi: 10.3390/vaccines8040586 [ DOI ] [ PMC free article ] [ PubMed ] [ Google Scholar ]
- 100. Gonzalo-Asensio J, Marinova D, Martin C, et al. . MTBVAC: attenuating the human pathogen of tuberculosis (TB) toward a promising vaccine against the TB epidemic. Front Immunol 2017; 8: 1803. doi: 10.3389/fimmu.2017.01803 [ DOI ] [ PMC free article ] [ PubMed ] [ Google Scholar ]
- 101. Nieuwenhuizen NE, Kulkarni PS, Shaligram U, et al. . The recombinant Bacille Calmette-Guerin vaccine VPM1002: ready for clinical efficacy testing. Front Immunol 2017; 8: 1147. doi: 10.3389/fimmu.2017.01147 [ DOI ] [ PMC free article ] [ PubMed ] [ Google Scholar ]
- 102. Ottenhoff THM. A trial of M72/AS01E vaccine to prevent tuberculosis. N Engl J Med 2020; 382: 1576–1577. doi: 10.1056/NEJMc2001364 [ DOI ] [ PubMed ] [ Google Scholar ]
- 103. Nabavinia MS, Naderi Nasab M, Meshkat Z, et al. . Construction of an expression vector containing Mtb72F of Mycobacterium tuberculosis . Cell J 2012; 14: 61–66. [ PMC free article ] [ PubMed ] [ Google Scholar ]
- 104. Working Group on New TB Drugs . Clinical Pipeline. www.newtbdrugs.org/pipeline/clinical Date last accessed 4 September 2021.
- View on publisher site
- PDF (458.4 KB)
- Collections
Similar articles
Cited by other articles, links to ncbi databases.
- Download .nbib .nbib
- Format: AMA APA MLA NLM
Add to Collections
Thank you for visiting nature.com. You are using a browser version with limited support for CSS. To obtain the best experience, we recommend you use a more up to date browser (or turn off compatibility mode in Internet Explorer). In the meantime, to ensure continued support, we are displaying the site without styles and JavaScript.
- View all journals
- Explore content
- About the journal
- Publish with us
- Sign up for alerts
- Review Article
- Published: 28 February 2024
Therapeutic developments for tuberculosis and nontuberculous mycobacterial lung disease
- Véronique Dartois ORCID: orcid.org/0000-0001-9470-5009 1 , 2 &
- Thomas Dick ORCID: orcid.org/0000-0002-9604-9452 1 , 2 , 3
Nature Reviews Drug Discovery volume 23 , pages 381–403 ( 2024 ) Cite this article
4550 Accesses
7 Citations
38 Altmetric
Metrics details
- Antibiotics
- Pharmaceutics
- Public health
Tuberculosis (TB) drug discovery and development has undergone nothing short of a revolution over the past 20 years. Successful public–private partnerships and sustained funding have delivered a much-improved understanding of mycobacterial disease biology and pharmacology and a healthy pipeline that can tolerate inevitable attrition. Preclinical and clinical development has evolved from decade-old concepts to adaptive designs that permit rapid evaluation of regimens that might greatly shorten treatment duration over the next decade. But the past 20 years also saw the rise of a fatal and difficult-to-cure lung disease caused by nontuberculous mycobacteria (NTM), for which the drug development pipeline is nearly empty. Here, we discuss the similarities and differences between TB and NTM lung diseases, compare the preclinical and clinical advances, and identify major knowledge gaps and areas of cross-fertilization. We argue that applying paradigms and networks that have proved successful for TB, from basic research to clinical trials, will help to populate the pipeline and accelerate curative regimen development for NTM disease.
This is a preview of subscription content, access via your institution
Access options
Access Nature and 54 other Nature Portfolio journals
Get Nature+, our best-value online-access subscription
24,99 € / 30 days
cancel any time
Subscribe to this journal
Receive 12 print issues and online access
195,33 € per year
only 16,28 € per issue
Buy this article
- Purchase on SpringerLink
- Instant access to full article PDF
Prices may be subject to local taxes which are calculated during checkout
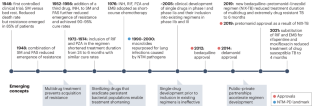
Similar content being viewed by others
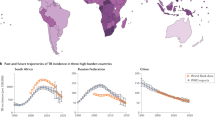
Anti-tuberculosis treatment strategies and drug development: challenges and priorities
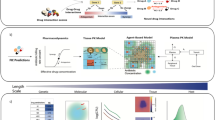
A multi-scale pipeline linking drug transcriptomics with pharmacokinetics predicts in vivo interactions of tuberculosis drugs
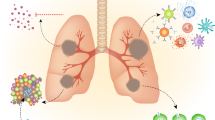
Therapeutic host-directed strategies to improve outcome in tuberculosis
WHO. Global Tuberculosis Report https://www.who.int/teams/global-tuberculosis-programme/tb-reports/global-tuberculosis-report-2022 (Geneva, 2022).
Pai, M., Kasaeva, T. & Swaminathan, S. Covid-19’s devastating effect on tuberculosis care – a path to recovery. N. Engl. J. Med. 386 , 1490–1493 (2022).
Article CAS PubMed Google Scholar
Marais, B. J., Hesseling, A. C. & Cotton, M. F. Poverty and tuberculosis: is it truly a simple inverse linear correlation? Eur. Respir. J. 33 , 943–944 (2009).
Dartois, V. A. & Rubin, E. J. Anti-tuberculosis treatment strategies and drug development: challenges and priorities. Nat. Rev. Microbiol. 20 , 685–701 (2022).
Article CAS PubMed PubMed Central Google Scholar
WHO. Global Tuberculosis Report https://www.who.int/publications/i/item/9789240037021 (Geneva, 2021).
WHO. WHO Consolidated Guidelines on Tuberculosis. Module 4: Drug-resistant Tuberculosis Treatment https://www.who.int/publications/i/item/9789240007048 (Geneva, 2020).
Motta, I. et al. Recent advances in the treatment of tuberculosis. Clin. Microbiol. Infect. https://doi.org/10.1016/j.cmi.2023.07.013 (2023).
Article PubMed Google Scholar
Evangelopoulos, D. & McHugh, T. D. Improving the tuberculosis drug development pipeline. Chem. Biol. Drug Des. 86 , 951–960 (2015).
Cowman, S., van Ingen, J., Griffith, D. E. & Loebinger, M. R. Non-tuberculous mycobacterial pulmonary disease. Eur. Respir. J. 54 , 1900250 (2019).
Winthrop, K. L. et al. Incidence and prevalence of nontuberculous mycobacterial lung disease in a large U.S. managed care health plan, 2008–2015. Ann. Am. Thorac. Soc. 17 , 178–185 (2020).
Article PubMed PubMed Central Google Scholar
Prevots, D. R. & Marras, T. K. Epidemiology of human pulmonary infection with nontuberculous mycobacteria: a review. Clin. Chest Med. 36 , 13–34 (2015).
Raju, R. M., Raju, S. M., Zhao, Y. & Rubin, E. J. Leveraging advances in tuberculosis diagnosis and treatment to address nontuberculous mycobacterial disease. Emerg. Infect. Dis. 22 , 365–369 (2016).
Sawka, A. & Burke, A. Medications and monitoring in treatment of nontuberculous mycobacteria lung disease. Clin. Chest Med. 44 , 815–828 (2023).
Daley, C. L. et al. Treatment of nontuberculous mycobacterial pulmonary disease: an official ATS/ERS/ESCMID/IDSA clinical practice guideline. Clin. Infect. Dis. 71 , 905–913 (2020). A comprehensive review by US and European physicians of evidence-based recommendations for the treatment of NTM-PD.
Egorova, A., Jackson, M., Gavrilyuk, V. & Makarov, V. Pipeline of anti- Mycobacterium abscessus small molecules: repurposable drugs and promising novel chemical entities. Med. Res. Rev. 41 , 2350–2387 (2021). A comprehensive review of the clinical and preclinical pipeline for MAB-PD, by mechanism of action and target.
Johansen, M. D., Herrmann, J. L. & Kremer, L. Non-tuberculous mycobacteria and the rise of Mycobacterium abscessus . Nat. Rev. Microbiol. 18 , 392–407 (2020). A review covering the biology, virulence factors, host interactions and drug resistance mechanisms of M. abscessus , one of the most antibiotic-resistant mycobacteria.
Griffith, D. E. & Aksamit, T. R. Understanding nontuberculous mycobacterial lung disease: it’s been a long time coming. F1000Res 5 , 2797 (2016).
Young, C., Walzl, G. & Du Plessis, N. Therapeutic host-directed strategies to improve outcome in tuberculosis. Mucosal Immunol. 13 , 190–204 (2020).
Tiwari, D. & Martineau, A. R. Inflammation-mediated tissue damage in pulmonary tuberculosis and host-directed therapeutic strategies. Semin. Immunol. 65 , 101672 (2022).
Lee, A., Xie, Y. L., Barry, C. E. & Chen, R. Y. Current and future treatments for tuberculosis. BMJ 368 , m216 (2020).
Anidi, I. U. & Olivier, K. N. Host-directed therapy in nontuberculous mycobacterial pulmonary disease: preclinical and clinical data review. Clin. Chest Med. 44 , 839–845 (2023).
Hatfull, G. F. Phage therapy for nontuberculous mycobacteria: challenges and opportunities. Pulm. Ther. 9 , 91–107 (2022).
Sterling, T. R. et al. Guidelines for the treatment of latent tuberculosis infection: recommendations from the National Tuberculosis Controllers Association and CDC, 2020. MMWR Recomm. Rep. 69 , 1–11 (2020).
Kumar, K. & Loebinger, M. R. Nontuberculous mycobacterial pulmonary disease: clinical epidemiologic features, risk factors, and diagnosis: the nontuberculous mycobacterial series. Chest 161 , 637–646 (2022).
Sharma, S. K., Mohan, A. & Kohli, M. Extrapulmonary tuberculosis. Expert. Rev. Respir. Med. 15 , 931–948 (2021).
Shih, D. C. et al. Extrapulmonary nontuberculous mycobacterial disease surveillance – Oregon, 2014–2016. MMWR Morb. Mortal. Wkly Rep. 67 , 854–857 (2018).
Wilkinson, R. J. et al. Tuberculous meningitis. Nat. Rev. Neurol. 13 , 581–598 (2017).
Ganchua, S. K. C., White, A. G., Klein, E. C. & Flynn, J. L. Lymph nodes — the neglected battlefield in tuberculosis. PLoS Pathog. 16 , e1008632 (2020).
Lin, P. L. et al. Sterilization of granulomas is common in active and latent tuberculosis despite within-host variability in bacterial killing. Nat. Med. 20 , 75–79 (2014).
Xie, Y. L. et al. Fourteen-day PET/CT imaging to monitor drug combination activity in treated individuals with tuberculosis. Sci. Transl. Med. 13 , eabd7618 (2021).
Zhu, J., Liu, Y. J. & Fortune, S. M. Spatiotemporal perspectives on tuberculosis chemotherapy. Curr. Opin. Microbiol. 72 , 102266 (2023).
Dorman, S. E. et al. Four-month rifapentine regimens with or without moxifloxacin for tuberculosis. N. Engl. J. Med. 384 , 1705–1718 (2021). The first successful trial in more than four decades of treatment shortening in patients with DS-TB, reducing therapy duration from 6 to 4 months.
Conradie, F. et al. Treatment of highly drug-resistant pulmonary tuberculosis. N. Engl. J. Med. 382 , 893–902 (2020). A landmark clinical trial that successfully reduced treatment duration from 18–24 months to 6 months for patients with drug-resistant TB, showing the power of novel mechanisms of action.
Dahl, V. N. et al. Global trends of pulmonary infections with nontuberculous mycobacteria: a systematic review. Int. J. Infect. Dis. 125 , 120–131 (2022).
Honda, J. R., Bernhard, J. N. & Chan, E. D. Natural disasters and nontuberculous mycobacteria: a recipe for increased disease? Chest 147 , 304–308 (2015).
Mirsaeidi, M. & Sadikot, R. T. Gender susceptibility to mycobacterial infections in patients with non-CF bronchiectasis. Int. J. Mycobacteriol. 4 , 92–96 (2015).
Andrejak, C. et al. Chronic respiratory disease, inhaled corticosteroids and risk of non-tuberculous mycobacteriosis. Thorax 68 , 256–262 (2013).
Chan, E. D. & Iseman, M. D. Underlying host risk factors for nontuberculous mycobacterial lung disease. Semin. Respir. Crit. Care Med. 34 , 110–123 (2013).
Abidin, N. Z. et al. Trends in nontuberculous mycobacteria infection in children and young people with cystic fibrosis. J. Cyst. Fibros. 20 , 737–741 (2021).
Brugha, R. & Spencer, H. Mycobacterium abscessus in cystic fibrosis. Science 372 , 465–466 (2021).
Honda, J. R., Virdi, R. & Chan, E. D. Global environmental nontuberculous mycobacteria and their contemporaneous man-made and natural niches. Front. Microbiol. 9 , 2029 (2018).
Kim, D. H. et al. In vitro activity and clinical outcomes of clofazimine for nontuberculous mycobacteria pulmonary disease. J. Clin. Med. 10 , 4581 (2021).
Pfaeffle, H. O. I. et al. Clofazimine for treatment of multidrug-resistant non-tuberculous mycobacteria. Pulm. Pharmacol. Ther. 70 , 102058 (2021).
Holt, M. R. & Baird, T. Treatment approaches to Mycobacterium abscessus pulmonary disease. Clin. Chest Med. 44 , 785–798 (2023). A concise but complete review of treatment guidelines, side effects, novel and repurposed therapeutic options for MAB-PD.
van Ingen, J., Boeree, M. J., van Soolingen, D. & Mouton, J. W. Resistance mechanisms and drug susceptibility testing of nontuberculous mycobacteria. Drug Resist. Updat. 15 , 149–161 (2012).
Falkinham, J. O. III Ecology of nontuberculous mycobacteria — where do human infections come from? Semin. Respir. Crit. Care Med. 34 , 95–102 (2013).
Fennelly, K. P. et al. Biofilm formation by Mycobacterium abscessus in a lung cavity. Am. J. Respir. Crit. Care Med. 193 , 692–693 (2016).
Henkle, E. & Winthrop, K. L. Nontuberculous mycobacteria infections in immunosuppressed hosts. Clin. Chest Med. 36 , 91–99 (2015).
O’Connell, M. L. et al. Lung manifestations in an autopsy-based series of pulmonary or disseminated nontuberculous mycobacterial disease. Chest 141 , 1203–1209 (2012).
Klein, J. L., Corbett, E. L., Slade, P. M., Miller, R. F. & Coker, R. J. Mycobacterium kansasii and human immunodeficiency virus co-infection in London. J. Infect. 37 , 252–259 (1998).
Tomashefski, J. F. Jr, Stern, R. C., Demko, C. A. & Doershuk, C. F. Nontuberculous mycobacteria in cystic fibrosis. An autopsy study. Am. J. Respir. Crit. Care Med. 154 , 523–528 (1996).
Swenson, C., Zerbe, C. S. & Fennelly, K. Host variability in NTM disease: implications for research needs. Front. Microbiol. 9 , 2901 (2018).
Koh, W. J., Hong, G., Kim, K., Ahn, S. & Han, J. Pulmonary sequestration infected with nontuberculous mycobacteria: a report of two cases and literature review. Asian Pac. J. Trop. Med. 5 , 917–919 (2012).
Merckx, J. J., Soule, E. H. & Karlson, A. G. The histopathology of lesions caused by infection with unclassified acid-fast bacteria in man. Report of 25 cases. Am. J. Clin. Pathol. 41 , 244–255 (1964).
Yuan, M. K. et al. Comparative chest computed tomography findings of non-tuberculous mycobacterial lung diseases and pulmonary tuberculosis in patients with acid fast bacilli smear-positive sputum. BMC Pulm. Med. 14 , 65 (2014).
Oshitani, Y. et al. Characteristic chest CT findings for progressive cavities in Mycobacterium avium complex pulmonary disease: a retrospective cohort study. Respir. Res. 21 , 10 (2020).
Jeong, Y. J. et al. Nontuberculous mycobacterial pulmonary infection in immunocompetent patients: comparison of thin-section CT and histopathologic findings. Radiology 231 , 880–886 (2004).
Kwon, Y. S. & Koh, W. J. Diagnosis of pulmonary tuberculosis and nontuberculous mycobacterial lung disease in Korea. Tuberc. Respir. Dis. 77 , 1–5 (2014).
Article Google Scholar
Jain, D., Ghosh, S., Teixeira, L. & Mukhopadhyay, S. Pathology of pulmonary tuberculosis and non-tuberculous mycobacterial lung disease: facts, misconceptions, and practical tips for pathologists. Semin. Diagn. Pathol. 34 , 518–529 (2017). A review of the radiological and immunopathological similarities and differences between pulmonary tuberculosis and NTM lung disease.
Barry, C. E. III et al. The spectrum of latent tuberculosis: rethinking the biology and intervention strategies. Nat. Rev. Microbiol. 7 , 845–855 (2009).
Griffith, D. E. et al. An official ATS/IDSA statement: diagnosis, treatment, and prevention of nontuberculous mycobacterial diseases. Am. J. Respir. Crit. Care Med. 175 , 367–416 (2007).
Jo, K. W., Park, Y. E., Chong, Y. P. & Shim, T. S. Spontaneous sputum conversion and reversion in Mycobacterium abscessus complex lung disease. PLoS ONE 15 , e0232161 (2020).
Kim, B., Yu, J. Y. & Jhun, B. W. Spontaneous cultural conversion rate of Mycobacterium avium complex pulmonary disease based on baces severity. J. Clin. Med. 12 , 7125 (2023).
National Tuberculosis Institute. Tuberculosis in a rural population of South India: a five-year epidemiological study. Bull. World Health Organ. 51 , 473–488 (1974).
PubMed Central Google Scholar
Mehra, S. et al. The DosR regulon modulates adaptive immunity and is essential for Mycobacterium tuberculosis persistence. Am. J. Respir. Crit. Care Med. 191 , 1185–1196 (2015).
Gerasimova, A., Kazakov, A. E., Arkin, A. P., Dubchak, I. & Gelfand, M. S. Comparative genomics of the dormancy regulons in mycobacteria. J. Bacteriol. 193 , 3446–3452 (2011).
Belardinelli, J. M. et al. Therapeutic efficacy of antimalarial drugs targeting DosRS signaling in Mycobacterium abscessus . Sci. Transl. Med. 14 , eabj3860 (2022).
Qvist, T. et al. Chronic pulmonary disease with Mycobacterium abscessus complex is a biofilm infection. Eur. Respir. J. 46 , 1823–1826 (2015).
Mishra, R. et al. Mechanopathology of biofilm-like Mycobacterium tuberculosis cords. Cell 186 , 5135–5150.e28 (2023).
Ankomah, P. & Levin, B. R. Exploring the collaboration between antibiotics and the immune response in the treatment of acute, self-limiting infections. Proc. Natl Acad. Sci. USA 111 , 8331–8338 (2014).
Brown-Elliott, B. A. & Woods, G. L. Antimycobacterial susceptibility testing of nontuberculous mycobacteria. J. Clin. Microbiol. 57 , e00834-19 (2019).
WHO. Rapid Communication: Key Changes to the Treatment of Drug-resistant Tuberculosis . https://www.who.int/publications/i/item/WHO-UCN-TB-2022-2 (2022).
Ji, B. et al. In vitro and in vivo activities of moxifloxacin and clinafloxacin against Mycobacterium tuberculosis . Antimicrob. Agents Chemother. 42 , 2066–2069 (1998).
Zurenko, G. E. et al. In vitro activities of U-100592 and U-100766, novel oxazolidinone antibacterial agents. Antimicrob. Agents Chemother. 40 , 839–845 (1996).
Yun, H. Y. et al. Model-based efficacy and toxicity comparisons of moxifloxacin for multidrug-resistant tuberculosis. Open Forum Infect. Dis. 9 , ofab660 (2022).
Alghamdi, W. A. et al. Population pharmacokinetics of linezolid in tuberculosis patients: dosing regimen simulation and target attainment analysis. Antimicrob. Agents Chemother. 64 , e01174-20 (2020).
Leach, K. L., Brickner, S. J., Noe, M. C. & Miller, P. F. Linezolid, the first oxazolidinone antibacterial agent. Ann. N. Y. Acad. Sci. 1222 , 49–54 (2011).
Ahmad, N. et al. Treatment correlates of successful outcomes in pulmonary multidrug-resistant tuberculosis: an individual patient data meta-analysis. Lancet 392 , 821–834 (2018).
Falzon, D. et al. Resistance to fluoroquinolones and second-line injectable drugs: impact on multidrug-resistant TB outcomes. Eur. Respir. J. 42 , 156–168 (2013).
Dheda, K. et al. The Lancet Respiratory Medicine Commission: 2019 update: epidemiology, pathogenesis, transmission, diagnosis, and management of multidrug-resistant and incurable tuberculosis. Lancet Respir. Med. 7 , 820–826 (2019).
Dreyer, V. et al. High fluoroquinolone resistance proportions among multidrug-resistant tuberculosis driven by dominant L2 Mycobacterium tuberculosis clones in the Mumbai Metropolitan Region. Genome Med. 14 , 95 (2022).
Agrawal, D., Udwadia, Z. F., Rodriguez, C. & Mehta, A. Increasing incidence of fluoroquinolone-resistant Mycobacterium tuberculosis in Mumbai, India. Int. J. Tuberc. Lung Dis. 13 , 79–83 (2009).
CAS PubMed Google Scholar
Mbelele, P. M. et al. Whole genome sequencing-based drug resistance predictions of multidrug-resistant Mycobacterium tuberculosis isolates from Tanzania. JAC Antimicrob. Resist. 4 , dlac042 (2022).
Ament, P. W., Jamshed, N. & Horne, J. P. Linezolid: its role in the treatment of Gram-positive, drug-resistant bacterial infections. Am. Fam. Physician 65 , 663–670 (2002).
PubMed Google Scholar
Thwaites, G. & Nguyen, N. V. Linezolid for drug-resistant tuberculosis. N. Engl. J. Med. 387 , 842–843 (2022).
Tse-Chang, A., Kunimoto, D., Der, E. & Ahmed, R. Assessment of linezolid efficacy, safety and tolerability in the treatment of tuberculosis: a retrospective case review. Can. J. Infect. Dis. Med. Microbiol. 24 , 535616 (2013).
Van Rie, A. et al. Balancing access to BPaLM regimens and risk of resistance. Lancet Infect. Dis. 22 , 1411–1412 (2022).
Kim, J. S. et al. Early bactericidal activity of delpazolid (LCB01-0371) in patients with pulmonary tuberculosis. Antimicrob. Agents Chemother. 66 , e0168421 (2022).
Wallis, R. S. et al. Mycobactericidal activity of sutezolid (PNU-100480) in sputum (EBA) and blood (WBA) of patients with pulmonary tuberculosis. PLoS ONE 9 , e94462 (2014).
Conradie, F. et al. Bedaquiline–pretomanid–linezolid regimens for drug-resistant tuberculosis. N. Engl. J. Med. 387 , 810–823 (2022).
Wallace, R. J. Jr et al. Initial clarithromycin monotherapy for Mycobacterium avium–intracellulare complex lung disease. Am. J. Respir. Crit. Care Med. 149 , 1335–1341 (1994).
Li, G. et al. Antimicrobial susceptibility of standard strains of nontuberculous mycobacteria by microplate Alamar Blue assay. PLoS ONE 8 , e84065 (2013).
Zheng, H. et al. In vitro activity of pretomanid against nontuberculous mycobacteria. Antimicrob. Agents Chemother. 66 , e0181021 (2022).
Yu, X. et al. In vitro activities of bedaquiline and delamanid against nontuberculous mycobacteria isolated in Beijing, China. Antimicrob. Agents Chemother. 63 , e00031-19 (2019).
Heifets, L., Higgins, M. & Simon, B. Pyrazinamide is not active against Mycobacterium tuberculosis residing in cultured human monocyte-derived macrophages. Int. J. Tuberc. Lung Dis. 4 , 491–495 (2000).
Reingewertz, T. H. et al. Differential sensitivity of mycobacteria to isoniazid is related to differences in KatG-mediated enzymatic activation of the drug. Antimicrob. Agents Chemother. 64 , e01899-19 (2020).
Cowman, S., Burns, K., Benson, S., Wilson, R. & Loebinger, M. R. The antimicrobial susceptibility of non-tuberculous mycobacteria. J. Infect. 72 , 324–331 (2016).
Kim, D. H. et al. In vitro activity of bedaquiline and delamanid against nontuberculous mycobacteria, including macrolide-resistant clinical isolates. Antimicrob. Agents Chemother. 63 , e00665-19 (2019).
Boorgula, G. D. et al. Isoniazid pharmacokinetics/pharmacodynamics as monotherapy and in combination regimen in the hollow fiber system model of Mycobacterium kansasii . Tuberculosis 138 , 102289 (2022).
Mudde, S. E., Upton, A. M., Lenaerts, A., Bax, H. I. & De Steenwinkel, J. E. M. Delamanid or pretomanid? A Solomonic judgement! J. Antimicrob. Chemother. 77 , 880–902 (2022).
Vilcheze, C. & Jacobs, W. R. Jr The isoniazid paradigm of killing, resistance, and persistence in Mycobacterium tuberculosis . J. Mol. Biol. 431 , 3450–3461 (2019).
Gopal, P., Gruber, G., Dartois, V. & Dick, T. Pharmacological and molecular mechanisms behind the sterilizing activity of pyrazinamide. Trends Pharmacol. Sci. 40 , 930–940 (2019).
Ushtanit, A. et al. Molecular determinants of ethionamide resistance in clinical isolates of Mycobacterium tuberculosis . Antibiotics 11 , 133 (2022).
Guerrero, C. et al. Evaluation of the rpoB gene in rifampicin-susceptible and -resistant Mycobacterium avium and Mycobacterium intracellulare . J. Antimicrob. Chemother. 33 , 661–663 (1994).
Moon, S. M. et al. Relationship between resistance to ethambutol and rifampin and clinical outcomes in Mycobacterium avium complex pulmonary disease. Antimicrob. Agents Chemother. 66 , e0202721 (2022).
Hombach, M., Somoskovi, A., Homke, R., Ritter, C. & Bottger, E. C. Drug susceptibility distributions in slowly growing non-tuberculous mycobacteria using MGIT 960 TB eXiST. Int. J. Med. Microbiol. 303 , 270–276 (2013).
Schon, T. et al. Evaluation of wild-type MIC distributions as a tool for determination of clinical breakpoints for Mycobacterium tuberculosis . J. Antimicrob. Chemother. 64 , 786–793 (2009).
Schildkraut, J. A. et al. The role of rifampicin within the treatment of Mycobacterium avium pulmonary disease. Antimicrob. Agents Chemother. 67 , e0087423 (2023).
Schon, T. & Chryssanthou, E. Minimum inhibitory concentration distributions for Mycobacterium avium complex — towards evidence-based susceptibility breakpoints. Int. J. Infect. Dis. 55 , 122–124 (2017).
Andries, K. et al. A diarylquinoline drug active on the ATP synthase of Mycobacterium tuberculosis . Science 307 , 223–227 (2005).
Lounis, N., Gevers, T., Van den Berg, J., Vranckx, L. & Andries, K. ATP synthase inhibition of Mycobacterium avium is not bactericidal. Antimicrob. Agents Chemother. 53 , 4927–4929 (2009).
Ruth, M. M. et al. A bedaquiline/clofazimine combination regimen might add activity to the treatment of clinically relevant non-tuberculous mycobacteria. J. Antimicrob. Chemother. 74 , 935–943 (2019).
Lindman, M. & Dick, T. Bedaquiline eliminates bactericidal activity of β-lactams against Mycobacterium abscessus . Antimicrob. Agents Chemother. 63 , e00827-19 (2019).
Froberg, G. et al. Towards clinical breakpoints for non-tuberculous mycobacteria – determination of epidemiological cut off values for the Mycobacterium avium complex and Mycobacterium abscessus using broth microdilution. Clin. Microbiol. Infect. 29 , 758–764 (2023).
Roemhild, R., Bollenbach, T. & Andersson, D. I. The physiology and genetics of bacterial responses to antibiotic combinations. Nat. Rev. Microbiol. 20 , 478–490 (2022).
Gupta, R. et al. The Mycobacterium tuberculosis protein LdtMt2 is a nonclassical transpeptidase required for virulence and resistance to amoxicillin. Nat. Med. 16 , 466–469 (2010).
Rifat, D., Chen, L., Kreiswirth, B. N. & Nuermberger, E. L. Genome-wide essentiality analysis of Mycobacterium abscessus by saturated transposon mutagenesis and deep sequencing. mBio 12 , e0104921 (2021).
Nguyen, D. C. et al. “One-Two Punch”: synergistic ss-lactam combinations for Mycobacterium abscessus and target redundancy in the inhibition of peptidoglycan synthesis enzymes. Clin. Infect. Dis. 73 , 1532–1536 (2021).
Negatu, D. A., Zimmerman, M., Dartois, V. A. & Dick, T. Strongly bactericidal all-oral β-lactam combinations for the treatment of Mycobacterium abscessus lung disease. Antimicrob. Agents Chemother. 66 , e0079022 (2022).
Lee, M. et al. Linezolid for treatment of chronic extensively drug-resistant tuberculosis. N. Engl. J. Med. 367 , 1508–1518 (2012).
Ignatius, E. H. & Dooley, K. E. New drugs for the treatment of tuberculosis. Clin. Chest Med. 40 , 811–827 (2019).
Cho, Y. L. & Jang, J. Development of delpazolid for the treatment of tuberculosis. Appl. Sci. 10 , 2211 (2020).
Article CAS Google Scholar
Negatu, D. A., Aragaw, W. W., Cangialosi, J., Dartois, V. & Dick, T. Side-by-side profiling of oxazolidinones to estimate the therapeutic window against mycobacterial infections. Antimicrob. Agents Chemother. 67 , e0165522 (2023).
Mdluli, K. C. et al. TBI-223: a safer oxazolidinone in pre-clinical development for tuberculosis. In ASM Microbe 2017 (ASM, 2017).
McLeay, S. C., Vis, P., van Heeswijk, R. P. & Green, B. Population pharmacokinetics of bedaquiline (TMC207), a novel antituberculosis drug. Antimicrob. Agents Chemother. 58 , 5315–5324 (2014).
Keutzer, L., Akhondipour Salehabad, Y., Davies Forsman, L. & Simonsson, U. S. H. A modeling-based proposal for safe and efficacious reintroduction of bedaquiline after dose interruption: a population pharmacokinetics study. CPT Pharmacomet. Syst. Pharmacol. 11 , 628–639 (2022).
Mallick, J. S., Nair, P., Abbew, E. T., Van Deun, A. & Decroo, T. Acquired bedaquiline resistance during the treatment of drug-resistant tuberculosis: a systematic review. JAC Antimicrob. Resist. 4 , dlac029 (2022).
Brown, T. S. et al. Genotype-phenotype characterization of serial Mycobacterium tuberculosis isolates in bedaquiline-resistant tuberculosis. Clin. Infect. Dis. https://doi.org/10.1093/cid/ciad596 (2023).
Sutherland, H. S. et al. Variations in the C-unit of bedaquiline provides analogues with improved biology and pharmacology. Bioorg. Med. Chem. 28 , 115213 (2020).
Briffotaux, J., Huang, W., Wang, X. & Gicquel, B. MmpS5/MmpL5 as an efflux pump in Mycobacterium species. Tuberculosis 107 , 13–19 (2017).
Xu, J. et al. Contribution of pretomanid to novel regimens containing bedaquiline with either linezolid or moxifloxacin and pyrazinamide in murine models of tuberculosis. Antimicrob. Agents Chemother. 63 , e00021-19 (2019).
Almeida, D. et al. Comparative efficacy of the novel diarylquinoline TBAJ-876 and bedaquiline against a resistant Rv0678 mutant in a mouse model of tuberculosis. Antimicrob. Agents Chemother. 65 , e0141221 (2021).
Yao, R. et al. Sudapyridine (WX-081), a novel compound against Mycobacterium tuberculosis . Microbiol. Spectr. 10 , e0247721 (2022).
Huang, Z. et al. Discovery and preclinical profile of sudapyridine (WX-081), a novel anti-tuberculosis agent. Bioorg. Med. Chem. Lett. 71 , 128824 (2022).
Sarathy, J. P. et al. TBAJ-876, a 3,5-dialkoxypyridine analogue of bedaquiline, is active against Mycobacterium abscessus . Antimicrob. Agents Chemother. 64 , e02404-19 (2020).
Di Modugno, E. et al. In vitro activity of the tribactam GV104326 against Gram-positive, Gram-negative, and anaerobic bacteria. Antimicrob. Agents Chemother. 38 , 2362–2368 (1994).
Lee, R. E. et al. Spectinamides: a new class of semisynthetic antituberculosis agents that overcome native drug efflux. Nat. Med. 20 , 152–158 (2014).
Robertson, G. T. et al. Spectinamides are effective partner agents for the treatment of tuberculosis in multiple mouse infection models. J. Antimicrob. Chemother. 72 , 770–777 (2017).
Rominski, A., Roditscheff, A., Selchow, P., Bottger, E. C. & Sander, P. Intrinsic rifamycin resistance of Mycobacterium abscessus is mediated by ADP-ribosyltransferase MAB_0591. J. Antimicrob. Chemother. 72 , 376–384 (2017).
Kumar, K., Daley, C. L., Griffith, D. E. & Loebinger, M. R. Management of Mycobacterium avium complex and Mycobacterium abscessus pulmonary disease: therapeutic advances and emerging treatments. Eur. Respir. Rev. 31 , 210212 (2022).
Baysarowich, J. et al. Rifamycin antibiotic resistance by ADP-ribosylation: structure and diversity of Arr. Proc. Natl Acad. Sci. USA 105 , 4886–4891 (2008).
Ganapathy, U. S. et al. Blocking bacterial naphthohydroquinone oxidation and ADP-ribosylation improves activity of rifamycins against Mycobacterium abscessus . Antimicrob. Agents Chemother. 65 , e0097821 (2021).
Prideaux, B. et al. The association between sterilizing activity and drug distribution into tuberculosis lesions. Nat. Med. 21 , 1223–1227 (2015).
Sarathy, J. P. et al. Extreme drug tolerance of Mycobacterium tuberculosis in caseum. Antimicrob. Agents Chemother. 62 , e02266–e02317 (2018).
Kolpen, M. et al. Biofilms of Mycobacterium abscessus complex can be sensitized to antibiotics by disaggregation and oxygenation. Antimicrob. Agents Chemother. 64 , e01212-19 (2020).
Lan, T. et al. Redesign of rifamycin antibiotics to overcome ADP-ribosylation-mediated resistance. Angew. Chem. Int. Ed. Engl. 61 , e202211498 (2022).
Paulowski, L. et al. C25-modified rifamycin derivatives with improved activity against Mycobacterium abscessus . PNAS Nexus 1 , pgac130 (2022).
Payne, D. J., Gwynn, M. N., Holmes, D. J. & Pompliano, D. L. Drugs for bad bugs: confronting the challenges of antibacterial discovery. Nat. Rev. Drug. Discov. 6 , 29–40 (2007).
Vincent, F. et al. Phenotypic drug discovery: recent successes, lessons learned and new directions. Nat. Rev. Drug. Discov. 21 , 899–914 (2022). A review discussing the successes and challenges of modern phenotypic — as opposed to target-based — drug discovery, which combines original concepts with modern tools and strategies.
Working Group on New TB Drugs. Clinical Pipeline https://www.newtbdrugs.org/pipeline/clinical (2023).
Working Group on New TB Drugs. 2023 Global New TB Drug Discovery Pipeline https://www.newtbdrugs.org/pipeline/discovery (2023).
Fernandes, G. F. S., Thompson, A. M., Castagnolo, D., Denny, W. A. & Dos Santos, J. L. Tuberculosis drug discovery: challenges and new horizons. J. Med. Chem. 65 , 7489–7531 (2022). A recent update of preclinical anti-TB compounds with in vivo efficacy against TB, and the global pipeline of drug candidates in clinical development, with a focus on mechanism of action.
Sethiya, J. P., Sowards, M. A., Jackson, M. & North, E. J. MmpL3 inhibition: a new approach to treat nontuberculous mycobacterial infections. Int. J. Mol. Sci. 21 , 6202 (2020).
Sarathy, J. P., Zimmerman, M. D., Gengenbacher, M., Dartois, V. & Dick, T. Mycobacterium tuberculosis DprE1 inhibitor OPC-167832 is active against Mycobacterium abscessus in vitro. Antimicrob. Agents Chemother. 66 , e0123722 (2022).
Li, W. et al. Direct inhibition of MmpL3 by novel antitubercular compounds. ACS Infect. Dis. 5 , 1001–1012 (2019).
Rudraraju, R. S. et al. Mycobacterium tuberculosis KasA as a drug target: structure-based inhibitor design. Front. Cell Infect. Microbiol. 12 , 1008213 (2022).
Fang, C. et al. Discovery of heterocyclic replacements for the coumarin core of anti-tubercular FadD32 inhibitors. Bioorg. Med. Chem. Lett. 28 , 3529–3533 (2018).
Aggarwal, A. et al. Development of a novel lead that targets M. tuberculosis polyketide synthase 13. Cell 170 , 249–259 e225 (2017).
Hugonnet, J. E., Tremblay, L. W., Boshoff, H. I., Barry, C. E. III & Blanchard, J. S. Meropenem–clavulanate is effective against extensively drug-resistant Mycobacterium tuberculosis . Science 323 , 1215–1218 (2009).
Vilchèze, C. Mycobacterial cell wall: a source of successful targets for old and new drugs. Appl. Sci. 10 , 2278 (2020).
Li, S. et al. CRISPRi chemical genetics and comparative genomics identify genes mediating drug potency in Mycobacterium tuberculosis . Nat. Microbiol. 7 , 766–779 (2022). A research article that describes the application of CRISPR interference to bacterial gene silencing to discover mechanisms of intrinsic and acquired resistance and targets for synergistic drug combinations.
Beites, T. et al. Plasticity of the Mycobacterium tuberculosis respiratory chain and its impact on tuberculosis drug development. Nat. Commun. 10 , 4970 (2019).
Jeffreys, L. N. et al. Identification of 2-aryl-quinolone inhibitors of cytochrome bd and chemical validation of combination strategies for respiratory inhibitors against Mycobacterium tuberculosis . ACS Infect. Dis. 9 , 221–238 (2023).
Lee, B. S. et al. Dual inhibition of the terminal oxidases eradicates antibiotic-tolerant Mycobacterium tuberculosis . EMBO Mol. Med. 13 , e13207 (2021).
Singh, R. et al. PA-824 kills nonreplicating Mycobacterium tuberculosis by intracellular NO release. Science 322 , 1392–1395 (2008).
Kwon, N. H., Fox, P. L. & Kim, S. Aminoacyl-tRNA synthetases as therapeutic targets. Nat. Rev. Drug. Discov. 18 , 629–650 (2019).
Li, X. et al. Discovery of a potent and specific M. tuberculosis leucyl-tRNA synthetase inhibitor: ( S )-3-(aminomethyl)-4-chloro-7-(2-hydroxyethoxy)benzo[ c ][1,2]oxaborol-1(3H)-ol (GSK656). J. Med. Chem. 60 , 8011–8026 (2017).
Green, S. R. et al. Lysyl-tRNA synthetase, a target for urgently needed M. tuberculosis drugs. Nat. Commun. 13 , 5992 (2022).
Abrahams, K. A. et al. Inhibiting mycobacterial tryptophan synthase by targeting the inter-subunit interface. Sci. Rep. 7 , 9430 (2017).
Brown, K. L. et al. Cyclic AMP-mediated inhibition of cholesterol catabolism in Mycobacterium tuberculosis by the novel drug candidate GSK2556286. Antimicrob. Agents Chemother. 67 , e0129422 (2023).
Kim, M. J. et al. Caseation of human tuberculosis granulomas correlates with elevated host lipid metabolism. EMBO Mol. Med. 2 , 258–274 (2010).
Guerrini, V. et al. Storage lipid studies in tuberculosis reveal that foam cell biogenesis is disease-specific. PLoS Pathog. 14 , e1007223 (2018).
Nuermberger, E. L. et al. GSK2556286 is a novel antitubercular drug candidate effective in vivo with the potential to shorten tuberculosis treatment. Antimicrob. Agents Chemother. 66 , e0013222 (2022).
Lupoli, T. J., Vaubourgeix, J., Burns-Huang, K. & Gold, B. Targeting the proteostasis network for mycobacterial drug discovery. ACS Infect. Dis. 4 , 478–498 (2018).
Bhandari, V. et al. The role of ClpP protease in bacterial pathogenesis and human diseases. ACS Chem. Biol. 13 , 1413–1425 (2018).
Hawkins, P. M. E. et al. Potent bactericidal antimycobacterials targeting the chaperone ClpC1 based on the depsipeptide natural products ecumicin and ohmyungsamycin A. J. Med. Chem. 65 , 4893–4908 (2022).
Maitre, T., Baulard, A., Aubry, A. & Veziris, N. Optimizing the use of current antituberculosis drugs to overcome drug resistance in Mycobacterium tuberculosis . Infect. Dis. Now. 54 , 104807 (2023).
Blondiaux, N. et al. Reversion of antibiotic resistance in Mycobacterium tuberculosis by spiroisoxazoline SMARt-420. Science 355 , 1206–1211 (2017).
Flipo, M. et al. The small-molecule SMARt751 reverses Mycobacterium tuberculosis resistance to ethionamide in acute and chronic mouse models of tuberculosis. Sci. Transl. Med. 14 , eaaz6280 (2022).
Rubin, E. J. Reviving a drug for tuberculosis? N. Engl. J. Med. 376 , 2292–2294 (2017).
Ganapathy, U. S. & Dick, T. Why matter matters: fast-tracking Mycobacterium abscessus drug discovery. Molecules 27 , 6948 (2022). A survey of anti-TB agents that have shown in vivo efficacy in MAB mouse models, showing the value of screening advanced TB chemical matter as a means of fast-tracking MAB drug discovery and highlighting the dire state of the pipeline.
Chong, S. L., Tan, J. L. & Ngeow, Y. F. The resistomes of Mycobacteroides abscessus complex and their possible acquisition from horizontal gene transfer. BMC Genomics 23 , 715 (2022).
Bekes, M., Langley, D. R. & Crews, C. M. PROTAC targeted protein degraders: the past is prologue. Nat. Rev. Drug. Discov. 21 , 181–200 (2022).
Morreale, F. E. et al. BacPROTACs mediate targeted protein degradation in bacteria. Cell 185 , 2338–2353 e2318 (2022).
Hoi, D. M. et al. Clp-targeting BacPROTACs impair mycobacterial proteostasis and survival. Cell 186 , 2176–2192.e22 (2023).
Nuermberger, E. L. Preclinical efficacy testing of new drug candidates. Microbiol. Spectr. 5 , https://doi.org/10.1128/microbiolspec.TBTB2-0034-2017 (2017).
Tasneen, R. et al. Novel regimens of bedaquiline-pyrazinamide combined with moxifloxacin, rifabutin, delamanid and/or OPC-167832 in murine tuberculosis models. Antimicrob. Agents Chemother. 66 , e0239821 (2022).
Irwin, S. M. et al. Presence of multiple lesion types with vastly different microenvironments in C3HeB/FeJ mice following aerosol infection with Mycobacterium tuberculosis . Dis. Model. Mech. 8 , 591–602 (2015).
Ernest, J. P. et al. Development of new tuberculosis drugs: translation to regimen composition for drug-sensitive and multidrug-resistant tuberculosis. Annu. Rev. Pharmacol. Toxicol. 61 , 495–516 (2021).
Via, L. E. et al. A sterilizing tuberculosis treatment regimen is associated with faster clearance of bacteria in cavitary lesions in marmosets. Antimicrob. Agents Chemother. 59 , 4181–4189 (2015).
Lin, P. L. et al. Radiologic responses in cynomolgous macaques for assessing tuberculosis chemotherapy regimens. Antimicrob. Agents Chemother. 57 , 4237–4244 (2013).
Obregon-Henao, A. et al. Susceptibility of Mycobacterium abscessus to antimycobacterial drugs in preclinical models. Antimicrob. Agents Chemother. 59 , 6904–6912 (2015).
Nicola, F., Cirillo, D. M. & Lore, N. I. Preclinical murine models to study lung infection with Mycobacterium abscessus complex. Tuberculosis 138 , 102301 (2023).
De Groote, M. A. et al. GM-CSF knockout mice for preclinical testing of agents with antimicrobial activity against Mycobacterium abscessus . J. Antimicrob. Chemother. 69 , 1057–1064 (2014).
Libardo, J., Boshoff, H. I. & Barry, C. E. III The present state of the tuberculosis drug development pipeline. Curr. Opin. Pharmacol. 42 , 81–94 (2018).
Article PubMed Central Google Scholar
Berg, A. et al. Model-based meta-analysis of relapsing mouse model studies from the critical path to tuberculosis drug regimens initiative database. Antimicrob. Agents Chemother. 66 , e0179321 (2022).
Bartelink, I. H. et al. New paradigm for translational modeling to predict long-term tuberculosis treatment response. Clin. Transl. Sci. 10 , 366–379 (2017).
Larkins-Ford, J., Degefu, Y. N., Van, N., Sokolov, A. & Aldridge, B. B. Design principles to assemble drug combinations for effective tuberculosis therapy using interpretable pairwise drug response measurements. Cell Rep. Med. 3 , 100737 (2022).
Mudde, S. E. et al. Predictive modeling to study the treatment-shortening potential of novel tuberculosis drug regimens, towards bundling of preclinical data. J. Infect. Dis. 225 , 1876–1885 (2021).
Pienaar, E. et al. A computational tool integrating host immunity with antibiotic dynamics to study tuberculosis treatment. J. Theor. Biol. 367 , 166–179 (2015).
Dooley, K. E., Hanna, D., Mave, V., Eisenach, K. & Savic, R. M. Advancing the development of new tuberculosis treatment regimens: the essential role of translational and clinical pharmacology and microbiology. PLoS Med. 16 , e1002842 (2019).
Davies, G. R. & Wallis, R. S. Methods for selecting regimen duration to prevent relapse in drug-susceptible and drug-resistant TB. Int. J. Tuberc. Lung Dis. 20 , 13–17 (2016).
Imperial, M. Z., Phillips, P. P. J., Nahid, P. & Savic, R. M. Precision-enhancing risk stratification tools for selecting optimal treatment durations in tuberculosis clinical trials. Am. J. Respir. Crit. Care Med. 204 , 1086–1096 (2021). A retrospective analyses of large TB clinical trials to develop a risk stratification tool for the selection of patient-specific optimal treatment durations.
Chen, R. Y. et al. Using biomarkers to predict TB treatment duration (Predict TB): a prospective, randomized, noninferiority, treatment shortening clinical trial. Gates Open Res. 1 , 9 (2017).
Nyang’wa, B. T. et al. A 24-week, all-oral regimen for rifampin-resistant tuberculosis. N. Engl. J. Med. 387 , 2331–2343 (2022).
Mok, J. et al. 9 months of delamanid, linezolid, levofloxacin, and pyrazinamide versus conventional therapy for treatment of fluoroquinolone-sensitive multidrug-resistant tuberculosis (MDR-END): a multicentre, randomised, open-label phase 2/3 non-inferiority trial in South Korea. Lancet 400 , 1522–1530 (2022).
Paton, N. I. et al. Treatment strategy for rifampin-susceptible tuberculosis. N. Engl. J. Med. 388 , 873–887 (2023).
The CRyPTIC Consortium.Genome-wide association studies of global Mycobacterium tuberculosis resistance to 13 antimicrobials in 10,228 genomes identify new resistance mechanisms. PLoS Biol. 20 , e3001755 (2022).
Fors, J., Strydom, N., Fox, W. S., Keizer, R. J. & Savic, R. M. Mathematical model and tool to explore shorter multi-drug therapy options for active pulmonary tuberculosis. PLoS Comput. Biol. 16 , e1008107 (2020).
Dheda, K. et al. Drug-penetration gradients associated with acquired drug resistance in patients with tuberculosis. Am. J. Respir. Crit. Care Med. 198 , 1208–1219 (2018).
Strydom, N. et al. Tuberculosis drugs’ distribution and emergence of resistance in patient’s lung lesions: a mechanistic model and tool for regimen and dose optimization. PLoS Med. 16 , e1002773 (2019).
Diacon, A. H. Two steps forward, one step back. N. Engl. J. Med. 387 , 2380–2381 (2022).
Lienhardt, C. et al. Advances in clinical trial design: weaving tomorrow’s TB treatments. PLoS Med. 17 , e1003059 (2020). A review and opinion of present and future advances in clinical trial design to harmonize and accelerate the development of novel TB regimens.
Davies, G., Boeree, M., Hermann, D. & Hoelscher, M. Accelerating the transition of new tuberculosis drug combinations from phase II to phase III trials: new technologies and innovative designs. PLoS Med. 16 , e1002851 (2019).
Biomarkers Development Working Group. Biomarkers and surrogate endpoints: preferred definitions and conceptual framework. Clin. Pharmacol. Ther. 69 , 89–95 (2001).
Boeree, M. J. et al. A dose-ranging trial to optimize the dose of rifampin in the treatment of tuberculosis. Am. J. Respir. Crit. Care Med. 191 , 1058–1065 (2015).
Wallis, R. S., Wang, C., Meyer, D. & Thomas, N. Month 2 culture status and treatment duration as predictors of tuberculosis relapse risk in a meta-regression model. PLoS ONE 8 , e71116 (2013).
Phillips, P. P. et al. Limited role of culture conversion for decision-making in individual patient care and for advancing novel regimens to confirmatory clinical trials. BMC Med. 14 , 19 (2016).
Davies, G. R. Early clinical development of anti-tuberculosis drugs: science, statistics and sterilizing activity. Tuberculosis 90 , 171–176 (2010).
Bouton, T. C. et al. An optimized background regimen design to evaluate the contribution of levofloxacin to multidrug-resistant tuberculosis treatment regimens: study protocol for a randomized controlled trial. Trials 18 , 563 (2017).
Aguilar Diaz, J. M. et al. New and repurposed drugs for the treatment of active tuberculosis: an update for clinicians. Respiration 102 , 83–100 (2022).
Esmail, A. et al. An all-oral 6-month regimen for multidrug-resistant tuberculosis: a multicenter, randomized controlled clinical trial (the NExT Study). Am. J. Respir. Crit. Care Med. 205 , 1214–1227 (2022).
Chang, V., Phillips, P. P. J., Imperial, M. Z., Nahid, P. & Savic, R. M. A comparison of clinical development pathways to advance tuberculosis regimen development. BMC Infect. Dis. 22 , 920 (2022).
Paton, N. I. et al. A treatment strategy for rifampicin-susceptible tuberculosis. N. Engl. J. Med. 388 , 873–887 (2023). A landmark clinical trial based on the pioneering paradigm of ‘stop-treatment-and-watch’ to discover treatment-shortening TB drug regimens.
Nogueira, B. M. F. et al. Diagnostic biomarkers for active tuberculosis: progress and challenges. EMBO Mol. Med. 14 , e14088 (2022).
CDC. Virtual Meeting of the Advisory Council for the Elimination of Tuberculosis https://www.cdc.gov/faca/committees/pdfs/acet/acet-minutes-20211214-15-508.pdf (2022).
Vinnard, C. et al. Assessing response to therapy for nontuberculous mycobacterial lung disease: quo vadis? Front. Microbiol. 9 , 2813 (2018).
Henkle, E. et al. Patient-reported symptom and health-related quality-of-life validation and responsiveness during the first 6 months of treatment for Mycobacterium avium complex pulmonary disease. Chest 164 , 53–64 (2023).
Cella, D. et al. Patient-Reported Outcomes in Performance Measurement (RTI Press, 2015).
Waglechner, N. et al. Genomic epidemiology of Mycobacterium abscessus in a Canadian cystic fibrosis centre. Sci. Rep. 12 , 16116 (2022).
Zumla, A., Nahid, P. & Cole, S. T. Advances in the development of new tuberculosis drugs and treatment regimens. Nat. Rev. Drug. Discov. 12 , 388–404 (2013). The predecessor of the present Review, showing the progress achieved and road travelled over the past 10 years.
Houben, R., Esmail, H., Cobelens, F., Williams, C. M. L. & Coussens, A. K. Tuberculosis prevalence: beyond the tip of the iceberg. Lancet Respir. Med. 10 , 537–539 (2022).
van Ingen, J. et al. Nontuberculous mycobacterial lung disease caused by Mycobacterium avium complex – disease burden, unmet needs, and advances in treatment developments. Expert Rev. Respir. Med. 15 , 1387–1401 (2021). An overview of treatment guidelines and numerous unmet needs for MAC-PD.
Adjemian, J., Olivier, K. N. & Prevots, D. R. Nontuberculous mycobacteria among patients with cystic fibrosis in the United States: screening practices and environmental risk. Am. J. Respir. Crit. Care Med. 190 , 581–586 (2014).
Diel, R., Lipman, M. & Hoefsloot, W. High mortality in patients with Mycobacterium avium complex lung disease: a systematic review. BMC Infect. Dis. 18 , 206 (2018).
Jhun, B. W. et al. Prognostic factors associated with long-term mortality in 1445 patients with nontuberculous mycobacterial pulmonary disease: a 15-year follow-up study. Eur. Respir. J. 55 , 1900798 (2020).
Griffith, D. E. & Daley, C. L. Treatment of Mycobacterium abscessus pulmonary disease. Chest 161 , 64–75 (2022).
Borisov, S. E. et al. Effectiveness and safety of bedaquiline-containing regimens in the treatment of MDR- and XDR-TB: a multicentre study. Eur. Respir. J. 49 , 1700387 (2017).
Ndjeka, N. et al. High treatment success rate for multidrug-resistant and extensively drug-resistant tuberculosis using a bedaquiline-containing treatment regimen. Eur. Respir. J. 52 , 1801528 (2018).
Xu, H. B., Jiang, R. H. & Li, L. Treatment outcomes for Mycobacterium avium complex: a systematic review and meta-analysis. Eur. J. Clin. Microbiol. Infect. Dis. 33 , 347–358 (2014).
Nasiri, M. J. et al. Antibiotic therapy success rate in pulmonary Mycobacterium avium complex: a systematic review and meta-analysis. Expert Rev. Anti Infect. Ther. 18 , 263–273 (2020).
Kwak, N. et al. Treatment outcomes of Mycobacterium avium complex lung disease: a systematic review and meta-analysis. Clin. Infect. Dis. 65 , 1077–1084 (2017).
Kwak, N. et al. Mycobacterium abscessus pulmonary disease: individual patient data meta-analysis. Eur. Respir. J. 54 , 1801991 (2019).
Yano, T. et al. Reduction of clofazimine by mycobacterial type 2 NADH:quinone oxidoreductase: a pathway for the generation of bactericidal levels of reactive oxygen species. J. Biol. Chem. 286 , 10276–10287 (2011).
Borisov, S. E. et al. Efficiency and safety of chemotherapy regimen with SQ109 in those suffering from multiple drug resistant tuberculosis [Russian]. Tuberculosis Lung Dis. 96 , 6–18 (2018).
Kim, J. et al. Safety, tolerability, pharmacokinetics, and metabolism of telacebec (Q203) for the treatment of tuberculosis: a randomized, placebo-controlled, multiple ascending dose phase 1B trial. Antimicrob. Agents Chemother. 67 , e0112322 (2022).
Cevik, M. Abstr. 109. SimpliciTB Results and hepatic safety of pretomanid regimens +/− pyrazinamide. CROI 2023 https://www.croiconference.org/abstract/simplicitb-results-and-hepatic-safety-of-pretomanid-regimens-pyrazinamide/ (2023).
Dierig, A. et al. A phase IIb, open-label, randomized controlled dose ranging multi-centre trial to evaluate the safety, tolerability, pharmacokinetics and exposure-response relationship of different doses of delpazolid in combination with bedaquiline delamanid moxifloxacin in adult subjects with newly diagnosed, uncomplicated, smear-positive, drug-sensitive pulmonary tuberculosis. Trials 24 , 382 (2023).
Jindani, A. et al. Four-month high-dose rifampicin regimens for pulmonary tuberculosis. N. Engl. J. Med. 384 , 1705–1718 (2023).
Google Scholar
Guglielmetti, L. et al. Evaluating newly approved drugs for multidrug-resistant tuberculosis (endTB): study protocol for an adaptive, multi-country randomized controlled trial. Trials 22 , 651 (2021).
Boeree, M. J. et al. High-dose rifampicin, moxifloxacin, and SQ109 for treating tuberculosis: a multi-arm, multi-stage randomised controlled trial. Lancet Infect. Dis. 17 , 39–49 (2017).
Fox, W., Ellard, G. A. & Mitchison, D. A. Studies on the treatment of tuberculosis undertaken by the British Medical Research Council tuberculosis units, 1946–1986, with relevant subsequent publications. Int. J. Tuberc. Lung Dis. 3 , S231–279 (1999).
Dorman, S. E. et al. Substitution of rifapentine for rifampin during intensive phase treatment of pulmonary tuberculosis: study 29 of the tuberculosis trials consortium. J. Infect. Dis. 206 , 1030–1040 (2012).
Gillespie, S. H. et al. Four-month moxifloxacin-based regimens for drug-sensitive tuberculosis. N. Engl. J. Med. 371 , 1577–1587 (2014).
WHO. Global Tuberculosis Report 2020 . Report No. ISBN 978-92-4-001313-1 (WHO, 2020).
Aldridge, B. B. et al. The tuberculosis drug accelerator at year 10: what have we learned? Nat. Med. 27 , 1333–1337 (2021). A review of achievements of the Tuberculosis Drug Accelerator, an experiment designed to facilitate collaboration in TB drug discovery by breaking down barriers between competing labs and institutions.
Mekota, A. M. et al. Building sustainable clinical trial sites in sub-Saharan Africa through networking, infrastructure improvement, training and conducting clinical studies: the PanACEA approach. Acta Trop. 238 , 106776 (2022).
Koele, S. E. et al. Early bactericidal activity studies for pulmonary tuberculosis: a systematic review of methodological aspects. Int. J. Antimicrob. Agents 61 , 106775 (2023).
Ntinginya, N. E. et al. Tuberculosis molecular bacterial load assay reveals early delayed bacterial killing in relapse patients. Clin. Infect. Dis. 76 , e990–e994 (2022).
Jones, A. et al. Sputum lipoarabinomannan (LAM) as a biomarker to determine sputum mycobacterial load: exploratory and model-based analyses of integrated data from four cohorts. BMC Infect. Dis. 22 , 327 (2022).
Kawasaki, M. et al. Lipoarabinomannan in sputum to detect bacterial load and treatment response in patients with pulmonary tuberculosis: analytic validation and evaluation in two cohorts. PLoS Med. 16 , e1002780 (2019).
le Roux, S. P. et al. Resistance-conferring mycobacterial mutations and quantification of early bactericidal activity. Am. J. Respir. Crit. Care Med. 203 , 635–637 (2021).
Mukamolova, G. V., Turapov, O., Malkin, J., Woltmann, G. & Barer, M. R. Resuscitation-promoting factors reveal an occult population of tubercle bacilli in sputum. Am. J. Respir. Crit. Care Med. 181 , 174–180 (2010).
Chengalroyen, M. D. et al. Detection and quantification of differentially culturable tubercle bacteria in sputum from patients with tuberculosis. Am. J. Respir. Crit. Care Med. 194 , 1532–1540 (2016).
Walter, N. D. et al. Mycobacterium tuberculosis precursor rRNA as a measure of treatment-shortening activity of drugs and regimens. Nat. Commun. 12 , 2899 (2021).
Download references
Acknowledgements
The authors thank A. Diacon for stimulating discussions on present and future biomarkers of TB drug response. T.D. and V.D. receive support from the National Institute of Allergy and Infectious Diseases of the NIH, Award Numbers R01AI132374 and U19 AI142731, and from the Bill & Melinda Gates Foundation, award number INV-004704, respectively. The content is solely the responsibility of the authors and does not necessarily represent the official views of the NIH.
Author information
Authors and affiliations.
Center for Discovery and Innovation, Hackensack Meridian Health, Nutley, NJ, USA
Véronique Dartois & Thomas Dick
Department of Medical Sciences, Hackensack Meridian School of Medicine, Nutley, NJ, USA
Department of Microbiology and Immunology, Georgetown University, Washington, DC, USA
Thomas Dick
You can also search for this author in PubMed Google Scholar
Contributions
V.D. researched data for the article. Both authors contributed substantially to discussion of the content, wrote the article, and edited and reviewed the manuscript before submission.
Corresponding author
Correspondence to Véronique Dartois .
Ethics declarations
Competing interests.
The authors declare no competing interests.
Peer review
Peer review information.
Nature Reviews Drug Discovery thanks Payam Nahid, Robert Wilkinson and the other, anonymous, reviewer(s) for their contribution to the peer review of this work.
Additional information
Publisher’s note Springer Nature remains neutral with regard to jurisdictional claims in published maps and institutional affiliations.
Related links
ClinicalTrials.gov: https://clinicaltrials.gov
MARK-TB: https://www.mark-tb.org/
Risk Stratification Tool for Tuberculosis Clinical Trial Design: http://saviclab.org/tb-risk/
STOP-TB partnership: https://www.stoptb.org/
The Critical Path to TB Drug Regimen: https://c-path.org/programs/cptr/
The European Accelerator of Tuberculosis Regime Project: https://era4tb.org/
The Project to Accelerate New Treatments for Tuberculosis: https://fnih.org/our-programs/project-accelerate-new-treatments-tuberculosis-pan-tb
The TB Drug Accelerator: https://www.tbdrugaccelerator.org/
Working Group on New Drugs: https://www.newtbdrugs.org/
The minimum inhibitory concentration above which an antimicrobial agent is considered to have a low probability of treatment success in the clinic.
The lowest concentration that inhibits bacterial growth in vitro.
Designed to show that the effect of a new treatment is not worse than that of an active control by more than a specified margin.
The relationship between concentrations achieved in the body or in the tissue of interest (PK) and the concentrations required to exert antibacterial effect (PD).
PTA analysis evaluates the plasma exposure of an antibiotic in a patient population (pharmacokinetics) against a target exposure required for efficacy and calculates the likelihood of achieving a specific pharmacokinetic–pharmacodynamic criterion (‘target′) expressed relative to the minimum inhibitory concentration for a pathogen in that patient population.
Conversion of sputum from which Mycobacterium tuberculosis 9MTB0 can be grown under standardized condition to sputum from which no M. tuberculosis can be cultured. This conversion from positive to negative is the best way to determine whether a patient is responding to treatment. Spontaneous conversion was seen in a fraction of patients during the pre-antibiotic era.
Rights and permissions
Springer Nature or its licensor (e.g. a society or other partner) holds exclusive rights to this article under a publishing agreement with the author(s) or other rightsholder(s); author self-archiving of the accepted manuscript version of this article is solely governed by the terms of such publishing agreement and applicable law.
Reprints and permissions
About this article
Cite this article.
Dartois, V., Dick, T. Therapeutic developments for tuberculosis and nontuberculous mycobacterial lung disease. Nat Rev Drug Discov 23 , 381–403 (2024). https://doi.org/10.1038/s41573-024-00897-5
Download citation
Accepted : 24 January 2024
Published : 28 February 2024
Issue Date : May 2024
DOI : https://doi.org/10.1038/s41573-024-00897-5
Share this article
Anyone you share the following link with will be able to read this content:
Sorry, a shareable link is not currently available for this article.
Provided by the Springer Nature SharedIt content-sharing initiative
Quick links
- Explore articles by subject
- Guide to authors
- Editorial policies
Sign up for the Nature Briefing: Translational Research newsletter — top stories in biotechnology, drug discovery and pharma.

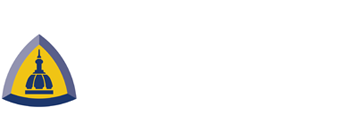
Latest News
Center for tb research — by the numbers.
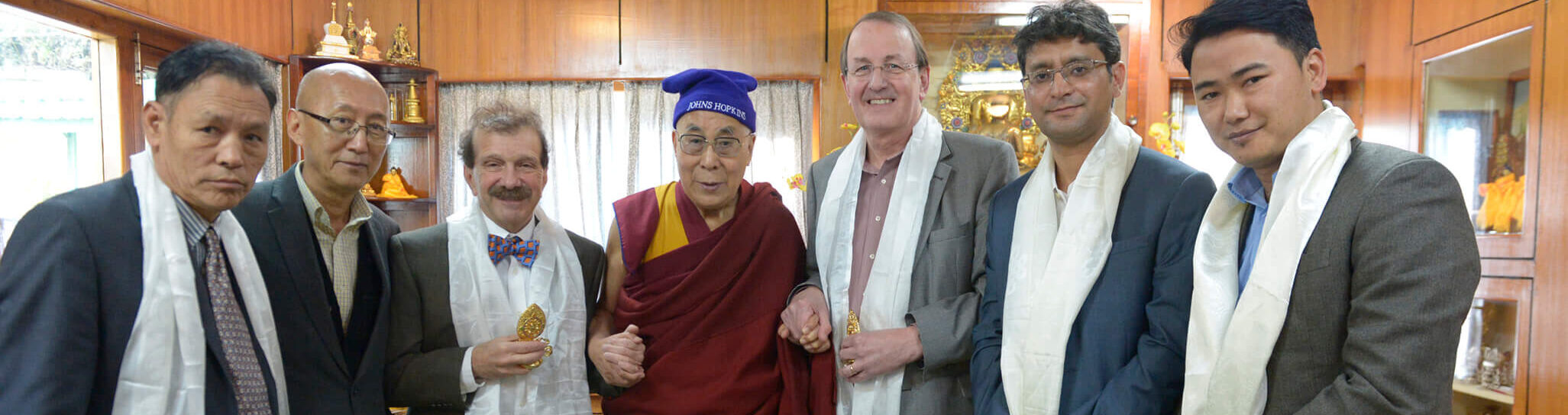
Click through the PLOS taxonomy to find articles in your field.
For more information about PLOS Subject Areas, click here .
Loading metrics
Open Access
Peer-reviewed
Research Article
Research Questions and Priorities for Tuberculosis: A Survey of Published Systematic Reviews and Meta-Analyses
Affiliation McGill University, Montreal, Quebec, Canada
Affiliation Emory University, Atlanta, Georgia, United States of America
Affiliation Stop TB Partnership, World Health Organization, Geneva, Switzerland
* E-mail: [email protected]
- Ioana Nicolau,
- Daphne Ling,
- Lulu Tian,
- Christian Lienhardt,
- Madhukar Pai
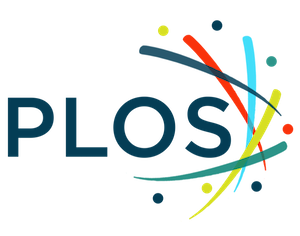
- Published: July 27, 2012
- https://doi.org/10.1371/journal.pone.0042479
- Reader Comments
Systematic reviews are increasingly informing policies in tuberculosis (TB) care and control. They may also be a source of questions for future research. As part of the process of developing the International Roadmap for TB Research, we did a systematic review of published systematic reviews on TB, to identify research priorities that are most frequently suggested in reviews.

Methodology/Principal Findings
We searched EMBASE, MEDLINE, Web of Science, and the Cochrane Library for systematic reviews and meta-analyses on any aspect of TB published between 2005 and 2010. One reviewer extracted data and a second reviewer independently extracted data from a random subset of included studies. In total, 137 systematic reviews, with 141 research questions, were included in this review. We used the UK Health Research Classification System (HRCS) to help us classify the research questions and priorities. The three most common research topics were in the area of detection, screening and diagnosis of TB (32.6%), development and evaluation of treatments and therapeutic interventions (23.4%), and TB aetiology and risk factors (19.9%). The research priorities determined were mainly focused on the discovery and evaluation of bacteriological TB tests and drug-resistant TB tests and immunological tests. Other important topics of future research were genetic susceptibility linked to TB and disease determinants attributed to HIV/TB. Evaluation of drug treatments for TB, drug-resistant TB and HIV/TB were also frequently proposed research topics.
Conclusions
Systematic reviews are a good source of key research priorities. Findings from our survey have informed the development of the International Roadmap for TB Research by the TB Research Movement.
Citation: Nicolau I, Ling D, Tian L, Lienhardt C, Pai M (2012) Research Questions and Priorities for Tuberculosis: A Survey of Published Systematic Reviews and Meta-Analyses. PLoS ONE 7(7): e42479. https://doi.org/10.1371/journal.pone.0042479
Editor: Markus M. Heimesaat, Charité, Campus Benjamin Franklin, Germany
Received: April 23, 2012; Accepted: June 26, 2012; Published: July 27, 2012
Copyright: © Nicolau et al. This is an open-access article distributed under the terms of the Creative Commons Attribution License, which permits unrestricted use, distribution, and reproduction in any medium, provided the original author and source are credited.
Funding: This work was supported in part by the Stop TB Partnership and World Health Organization. Dr Christian Lienhardt from the Stop TB Partnership and WHO provided input in study design and interpretation and revised the manuscript for intellectual content. Additional funding was provided by the European-Developing Countries Clinical Trials Programme (EDCTP; TB-NEAT grant). EDCTP had no role in study design, data collection and analysis, decision to publish, or preparation of the manuscript.
Competing interests: Madhukar Pai is a Section Editor with PLoS ONE. This does not alter the authors' adherence to all the PLoS ONE policies on sharing data and materials.
Introduction
Tuberculosis (TB) continues to pose a major threat to global health [1] , and research is a key component of the Global Plan to Stop TB2011-2015 [2] . Research is particularly critical for developing new tools and approaches needed for eliminating TB by 2050 [3] . Recognizing this, the Stop TB Partnership and the World Health Organization's (WHO) Stop TB Department have launched the TB Research Movement, with the aim of boosting TB research and accelerating progress in TB control towards international targets [4] . One of the main outputs of the TB Research Movement in 2011 was the publication of the International Roadmap for Tuberculosis Research [5] in October of 2011. This roadmap outlines all priority areas for investment in TB research and is intended to promote coordination and harmonization of scientific work on TB. Research priorities are identified in the areas of epidemiology; fundamental research; R&D of new diagnostics, drugs and vaccines; and operational and public health research. The ultimate goal is to reach all populations, including people with TB/HIV co-infection or MDR-TB and children, with new and better methods of prevention, diagnosis and treatment [5] .
The process for developing this roadmap has been recently described by Lienhardt and colleagues [4] . Briefly, the research roadmap was developed through a priority ranking exercise conducted by a multidisciplinary group of 50 research experts, a multidisciplinary Delphi consultation, a series of systematic reviews and an open web-based survey [4] .Among the systematic reviews that were commissioned, one was focused on all the TB research agendas that have been published from 1998 to 2010 [6] . As a next step, we were commissioned to review all the published systematic reviews and meta-analyses on TB (in all areas, including drugs, vaccines, diagnostics), to assess what research priorities have been identified in these reviews. The objectives of our systematic review were as follows: (1) to identify all systematic reviews and meta-analyses pertaining to any aspect of tuberculosis from 2005 to 2010, and (2) to assess, compile and rank the research priorities that were identified.
MEDLINE, EMBASE, Web of Science, and the Cochrane Library were searched for systematic reviews and meta-analyses on TB. The search strategy was developed in consultation with a medical librarian. The search was limited to systematic reviews and meta-analyses published between January 1, 2005 and July 1, 2010, in order to focus on contemporary TB literature and identify research priorities of greatest relevance to current TB control.
The search strategy included the following keywords and MeSH terms: [‘tuberculosis’ (explode) OR ‘ Mycobacterium tuberculosis ’(explode) OR ‘tuberculosis’.ti,ab. OR ‘tuberculos*’.tw] AND [‘meta analysis’ (explode) OR ‘meta analyses’.ti,ab OR ‘meta-analyses’.ti,ab OR ‘meta-analysis’.ti,ab OR ‘metanalys*’.ti,ab OR ‘systematic review’.tw]. The search was not limited to English and the last search was performed on August 18, 2010.
Studies were included if they focused on any aspect of tuberculosis. We included systematic reviews and meta-analyses published in English, French, Spanish, and Italian. The languages included were based on the skill set of our research team. We included systematic review and meta-analyses that had focused on tuberculosis or on a tuberculosis related topic (e.g. BCG), in the title or abstract. We considered a study to be a systematic review or meta-analysis if the authors identified the study as such, or if the title or abstract contained the words “systematic review” or “meta-analysis”. Moreover, studies were regarded as systematic reviews if the authors reported a systematic, explicit approach to identify, select, and synthesize the available evidence.
The first screening of the titles and abstracts obtained following the electronic search was done by one reviewer (IN). Subsequently, the same reviewer (IN) screened the full text articles, determined the eligibility, and decided on the final inclusion of studies in the systematic review. Further, a second reviewer (MP) independently searched, screened and identified studies for the inclusion in the review.
Data abstraction
We developed a data extraction form which was pilot-tested by two reviewers (IN and DL). The reviewers independently piloted the forms until there were no major disagreements in the data extraction process. One reviewer (IN) extracted the data from all the included studies and the second reviewer (DL) extracted data in duplicate for a random subset of 15% of the total number of included articles. Additionally, a third reviewer (LT) independently extracted data for all included studies on the study characteristics section of the data extraction form. Disagreements between the three reviewers were resolved by consensus.
Study characteristics
We extracted data from the text or online supplement of each included systematic review or meta-analysis. Information was collected on two main points: i) the main focus of the systematic review, and ii) questions and priorities identified for future research. The UK Health Research Classification System (HRCS) [7] , developed by the UK Clinical Research Collaboration for the classification and analysis of all types of health research, was used to determine the focus of the included studies as well as the focus of the research questions/priorities. In particular, the HRCS Research Activity Codes [7] were used to assign a category for the main focus of the studies and the research questions/priorities.
The main focus of each included systematic review was determined by extracting keywords from the title and abstract and matching them with the criteria developed by the HRCS. The Codes were divided into eight major categories: (1) Underpinning research; (2) Aetiology; (3) Prevention of disease and conditions, and promotion of well-being; (4) Detection, screening and diagnosis; (5) Development of treatments and therapeutic interventions; (6) Evaluation of treatments and therapeutic interventions; (7) Management of diseases and conditions; and (8) Health and social care services research (see Table 1 for full description). These research categories were used in Tables 2 and 3 , to provide an overarching framework for grouping TB research.
- PPT PowerPoint slide
- PNG larger image
- TIFF original image
https://doi.org/10.1371/journal.pone.0042479.t001
https://doi.org/10.1371/journal.pone.0042479.t002
https://doi.org/10.1371/journal.pone.0042479.t003
In the HRCS, each of the eight major categories is further subdivided into five to nine subcategories with definitions for the type of research that belonged to that subcategory. For instance, “(1)Underpinning research” includes five subcategories: (1.1)studies of normal biological development and functioning, including gene, gene products, biological pathways, molecular and cellular structures, and development and characterization of model systems; (1.2) studies that do not address health directly but cover issues such as psychological and socioeconomic processes, individual or group characteristics and behaviours, and social and cultural beliefs; (1.3) research in chemical and physical sciences that may lead to the future development of diagnostic tools or treatments; (1.4) studies that target the development of novel methodologies and measurements including the development of statistical methods, and the development of mapping methodologies; and (1.5)research involving the development and/or distribution of resources for use by the research community, and infrastructure to support research networks. Using the main categories and the subdivisions within each category, we mapped the corresponding TB research areas found in the literature search (refer to Tables 1 and 2 ).
Quantitative data synthesis
Study characteristics were summarized using descriptive statistics. Measures such as total count, frequency, and proportion, were used to summarize data. Data analyses were performed using STATA Version 11.0.
There were a total of 973 records identified through the electronic database search ( Figure 1 ). The first screening of titles and abstracts was done on 680 records. Following the first screening process, 528 records were excluded. The reasons for exclusion are listed in Figure 1 . The full text screening of articles was performed on 152 records. Overall, there were 137 systematic reviews included in our analysis [8] – [144] .
https://doi.org/10.1371/journal.pone.0042479.g001
Characteristics of included TB systematic reviews
The 137 reviews were published in 61 different journals. The majority of reviews (39.4%) were published in journals with impact factors of five or less, and only six (4.3%) reviews were published in journals with a high impact factor (>15). However, a large proportion of the reviews (38.6%) were published in journals that did not have an impact factor. In addition, approximately 24% of the main authors were from the United States and 41% were from four other countries (China, UK, Canada, and Italy). The remaining 34.1% of authors were from 26 different countries.
Out of the 137 reviews, 131 (95.6%) self identified as a systematic review or meta-analysis, which means that they used the term “systematic review” or “meta-analysis” in the title or abstract. Approximately 91% (124) of all reviews were not Cochrane reviews. Among the 13 Cochrane reviews, 9 of them focused on “evaluation of treatments and therapeutic interventions”.
Half of the reviews (67 [48.9%]) reported having a funding source, whereas only 15 reviews (11.0%) reported not being funded and 55 reviews (40.1%) did not report funding status. Most of the reviews (109 [79.6%]) included less than 50 studies in their review and within those reviews, the majority had between 1,000 and 10,000 participants (34/109[31.2%]).
Focus of TB systematic reviews
The main focus of each review was determined using the HRCS as described in the Methods section. The classification categories were subdivided into major tuberculosis research areas as described in Table 2 . The three most common review categories, in decreasing order, were “Detection, screening and diagnosis” with 46/141(32.6%) systematic reviews, “Development and evaluation of treatments and therapeutic interventions” with 33/141(23.4%) systematic reviews and “Aetiology” with 28/141(19.9%) systematic reviews.
Within the category of “Detection, screening and diagnosis”, 17/46 (37%) of the reviews focused on bacteriological diagnostics for active TB, such as improving processing methods of sputum smear microscopy, and assessing the use of nucleic acid amplification tests (NAATs). The two other most common TB research aims were bacteriological diagnostics for MDR-TB (9/46[20%]) and immunological diagnostics (9/46[20%]). More specifically, bacteriological diagnostics for MDR-TB included tests such as line-probe assays, bacteriophage based assays, and colorimetric redox assays. Immunological diagnostics were focused mainly on testing and evaluating interferon-gamma release assays (IGRAs).
In the category “Development and evaluation of treatments and therapeutic interventions”, 10/33 (30%) studies focused on drug resistant tuberculosis treatment, 9/33 (27.3%) studies on evaluating different regimen combinations for tuberculosis treatment, and 6/33(18.2%) on treatment of latent tuberculosis infection (LTBI).
In the category “Aetiology”, 11/28 (39.3%) systematic reviews focused on biological/genetic risk factors such as genetic susceptibility and gene targets,11/28 (39.3%) studies targeted surveillance and distribution of TB/HIV co-infection, MDRTB and HIV, and diabetes and TB, and 5/28(17.9%) focused on travel risk for LTBI and nosocomial TB exposure.
Research priorities
Out of 137 reviews, 103 (75%) identified at least one research question or a research priority. Of these, 48 (46.6%) identified only one research priority, 33 (32.0%) two research priorities, 7 (6.8%) three, 7 (6.8%) four, and 8 (7.8%) five research priorities. None of the reviews identified more than five research priorities.
Table 3 shows the summary of research priorities by category, subdivision, and TB-specific research priority. The three major categories of research priorities/questions were “Detection, screening and diagnosis” responsible for 50/191 (26.2%) of all the identified research priorities, “Aetiology” with 42/191 (22.0%), and “Evaluation of treatments and therapeutic interventions” with 37/191 (19.4%).
In the most common category, “Detection, screening and diagnosis”, the top research priority was the evaluation of bacteriological TB diagnostic tests in 14/50 (28.0%) reviews. Other frequently cited TB research priorities were: evaluation of immunological TB diagnostic tests (6/50 [12.0%]); discovery and development of new TB diagnostic tests (5/50 [10.2%]); and development of new bacteriological MDR-TB diagnostics (5/50 [10.2%]). Two priorities had almost equal importance and were highly prevalent in TB literature. The main priority in that category was to investigate the detection, screening and diagnosis of drug-resistant TB and MDR-TB. Studies called for the need to develop studies that detect resistance from smear positive specimens, determine the accuracy of colorimetric methods, line-probe assays, phage-based assays for rapid screening and nitrate reductase assay (NRA), and find the clinical usefulness of rapid diagnosis of rifampicin-resistant TB. Another frequency priority was to address unresolved research questions on interferon-gamma release assays (IGRAs), discover new antigens with immunodiagnostic potential, and test IGRAs in various populations and settings to establish test reproducibility. Evaluating sputum processing methods and smear microscopy, assessing nucleic acid amplification tests (NAATs), and evaluating tests for extrapulmonary TB (e.g. adenosine deaminase for pleural TB) were commonly cited priorities.
Within the “Aetiology” category, the main TB research priorities were: development of new research methods; better study designs or statistical tools for studying drug resistant TB, MDR-TB, links between HIV and MDR-TB; comparison of diagnostic tests (17/42 [40.5%]); identification of biological and genetic risk factors (15/42 [35.7%]); and evaluation of the role of risk factors such as tobacco and air pollutants (7/42 [16.7%]). The most frequent priority was to examine gene and gene products in relation to TB disease and susceptibility to disease. Key genes such as vitamin D receptor polymorphisms, IL10 gene, and drug-metabolizing enzyme (DME) gene polymorphisms were commonly mentioned for future research. The second most frequent research priority on TB/HIV included recommendations to conduct studies investigating XDR-TB and HIV co-infection, identifying a comprehensive definition of IRIS (immune reconstitution inflammatory syndrome), and investigating sputum processing methods with direct smears in settings with high and low HIV prevalence.
The category “Evaluation of treatments and therapeutic interventions” was the third most frequent. It focused on TB/HIV drug treatments (12/37 [32.4%]), drug-resistant TB treatments (11/37 [29.7%]), new TB drugs and active tuberculosis regimens (8/37[21.6%]). Implementing studies that evaluate new treatments and therapeutic interventions for drug-resistant TB, MDR-TB, and XDR-TB, was a prominent research priority. Such studies would need to examine methods to improve treatment outcomes for patients with XDR TB such as using later-generation fluoroquinolones, discovering methods to tailor treatment regimens for various forms of TB drug resistance, and investigating the use of quality-controlled laboratory testing for all first and second-line drugs that define XDR-TB. Another frequently cited priority was designing trials to evaluate the optimal duration of TB treatment, the influence of level of immunosuppression on effectiveness of TB drugs, and the combination of anti-TB chemoprophylaxis with antiretroviral therapy.
Systematic reviews and meta-analyses are widely acknowledged as a key component of the policy and guideline development process [145] . A large number of systematic reviews have been published in the area of TB diagnostics [146] , and these are increasingly being used for developing guidelines [147] . To this end, the Grading of Recommendations Assessment, Development and Evaluation (GRADE) tool has increasingly been adopted by policy makers and guideline developers to provide an explicit, comprehensive and transparent process for moving from evidence to recommendations [145] .
Systematic reviews often conclude by making suggestions for the direction of future research, and thus could be a good source for identifying the most important questions for TB research. Our survey collected descriptive information from all eligible systematic reviews and meta-analyses that were subsequently used to generate a list of research priorities in TB which were used for developing the International Roadmap for Tuberculosis Research [5] .
Our systematic search showed that a fairly high number of systematic reviews were published on TB during the period of 2005 to 2010. The findings of our review need to be interpreted along with a recent systematic review by Rylance and colleagues [6] on 33 articles with research agendas on TB. These authors found that the top priority areas for research were new TB drug development (28 articles), diagnosis and diagnostic tests (27), epidemiology (20), health services research (16), basic research (13), and vaccine development and use (13).
In our review of 137 TB systematic reviews, the top three categories for the focus of the research priorities/questions were “Detection, screening and diagnosis” “Aetiology” and “Evaluation of treatments and therapeutic interventions.” TB diagnosis and treatment were among the most important research priorities in both reviews. One possible reason of why TB diagnosis research ranked high on our list could be that our review focused on years 2005 to 2010, a period when major advances have been made in TB diagnostics, especially with IGRAs becoming a very popular subject of research [148] . Also, this time period saw the introduction of several WHO policies on TB diagnostics. Further, the emphasis on new tools in the Global Plan to Stop TB 2006–2015 [149] , along with the creation of product development partnerships such as the Foundation for Innovative New Diagnostics (FIND), AERAS, and Global Alliance for TB Drug Development, may have inspired research on new diagnostics and drugs.
The research priorities determined were mainly focused on the discovery and evaluation of bacteriological TB tests, drug-resistant TB tests and immunological tests, with special focus on IGRA tests. Also, tests for extra-pulmonary TB came up as a frequently cited priority in the Detection of TB category. Other important topics of future research were genetic susceptibility to TB and disease determinants attributed to HIV/TB. Evaluation of drug treatments for TB, drug-resistant TB and HIV/TB were also frequently proposed research topics. Many articles cited the need for improved and tailored treatment methods for MDR-TB and XDR-TB.
Although several systematic reviews identified areas for further research, the questions themselves were often framed in a generic way, rather than in a highly focused manner with specific recommendation for action. Future TB systematic reviews will need to be more focused, and propose highly specific, answerable questions that are amenable to well-designed research studies.
Our study has several limitations. Due to the poor overall quality of reporting of the systematic reviews, the findings may not be representative of the general output from the TB research community [150] . The inclusion of eligible studies was limited by the fact that we only reviewed articles in three other languages besides English. We were also unable to search ‘grey’ literature, contact authors, or hand search journals. The review also did not include any unpublished literature. Due to its overarching and generic nature, the Health Research Classification System categories were at times non-specific and difficult to match with specific areas of TB research. Furthermore, it was difficult to classify research priorities into narrow subdivisions since some research priorities could qualify for more than one subdivision. By categorizing research priorities into larger, predefined categories, we lost detailed information on individual research priorities. To remedy this, we condensed each priority and extracted the topic words from it. The topic words were then grouped together to form the summary of repeated priorities/questions and calculate the frequency.
There has been a lot of recent attention and focus on childhood TB, but because our search was last performed in 2010, our analysis may have missed research priorities in this important area.
In summary, our systematic review of published systematic reviews on TB helped identify several key priorities for future TB research. This exercise was useful to describe the landscape of TB research and the overarching TB research themes arising from systematic reviews and meta-analyses conducted over the last 5 years. Their scope is, however, limited, since systematic reviews themselves are influenced by current hot topics or new technologies. They are nevertheless useful in indicating research priorities on areas that receive high attention, either due to recent scientific developments or increasing questions surrounding advancement of knowledge in these very areas. They bring useful additional arguments and information to the broader, deeper and more rigorously conducted process of international research agenda development.
Author Contributions
Conceived and designed the experiments: IN CL MP. Performed the experiments: IN DL LT. Analyzed the data: IN CL MP. Wrote the paper: IN MP.
- 1. World Health Organization (2011) Global tuberculosis control 2011. Geneva: World Health Organization. 1–242 p.
- 2. World Health Organization (2010) Global Plan to Stop Tuberculosis 2011–2015. Geneva: World Health Organization. 1–242 p.
- View Article
- Google Scholar
- 5. Stop TB Partnership & World Health Organization (2011) An international roadmap for tuberculosis research: towards a world free of tuberculosis. Geneva: World Health Organization.
- 7. UK Clinical Research Collaboration (2011) Health Research Classification System (HRCS). London, UK: UKCRC.
- 39. Fraser A, Paul M, Attamna A, Leibovici L (2006) Drugs for preventing tuberculosis in people at risk of multiple-drug-resistant pulmonary tuberculosis. Cochrane Database of Systematic Reviews. Chichester, UK: John Wiley & Sons, Ltd.
- 85. Lutge Elizabeth E, Knight Stephen E, Volmink J (2009) Incentives for improving patient adherence to anti-tuberculosis treatment. Cochrane Database of Systematic Reviews. Chichester, UK: John Wiley & Sons, Ltd.
- 136. Vlassov Vasiliy V, Reze Andrey G (2006) Low level laser therapy for treating tuberculosis. Cochrane Database of Systematic Reviews. Chichester, UK: John Wiley & Sons, Ltd.
- 149. Stop TB Partnership (2006) The Global Plan to Stop TB, 2006–2015: Actions for life: towards a world free of tuberculosis. Geneva: World Health Organization (WHO/HTM/STB/2006.35).
An official website of the United States government
Official websites use .gov A .gov website belongs to an official government organization in the United States.
Secure .gov websites use HTTPS A lock ( Lock Locked padlock icon ) or https:// means you've safely connected to the .gov website. Share sensitive information only on official, secure websites.
- Publications
- Account settings
- Advanced Search
- Journal List

Priorities for tuberculosis research: a systematic review
Jamie rylance , dr, mrcp, madhukar pai , md, christian lienhardt , phd, paul garner , prof, md.
- Author information
- Copyright and License information
Correspondence to: Dr Jamie Rylance, Malawi-Liverpool-Wellcome Trust Clinical Research Programme, PO Box 30096, Chichiri, Blantyre 3, Malawi [email protected]
This work is licensed under a Creative Commons Attribution 4.0 International License , which allows reusers to distribute, remix, adapt, and build upon the material in any medium or format, so long as attribution is given to the creator. The license allows for commercial use.
Reliable and relevant research can help to improve tuberculosis control worldwide. In recent years, various organisations have assessed research needs and proposed priorities for tuberculosis. We summarise existing priority statements and assess the rigour of the methods used to generate them. We found 33 documents that specifically outline priorities in tuberculosis research. The top priority areas were drug development (28 articles), diagnosis and diagnostic tests (27), epidemiology (20), health services research (16), basic research (13), and vaccine development and use (13). The most focused questions were on the treatment and prevention of multidrug-resistant tuberculosis in people co-infected with HIV. Methods used to identify these priorities were varied. Improvements can be made to ensure the process is more rigorous and transparent, and to use existing research or systematic reviews more often. WHO, Stop TB Partnership, and other organisations could adopt an incremental process of priority development, building on the existing knowledge base.
Introduction
There are more than 9 million new cases of tuberculosis every year worldwide, and incidence is declining at a rate of less than 1% per year. 1 Nearly 2 million people die from tuberculosis every year, and the costs and social consequences of this disease are vast. This worldwide burden of tuberculosis has stimulated much interest in research for new approaches to the management of this disease. Various organisations, individuals, and networks have tried to identify priorities to help guide and to stimulate appropriate funding. The explicit and rational setting of research priorities is integral to the research process: for allocation of resources into areas of strategic importance, to catalyse debate, and to strengthen the role of stakeholders in establishing the research agenda. 2 Ultimately, this strategy should help to improve the allocation and monitoring of funding 3 and the progress towards targets in tuberculosis control. 4
Several approaches to facilitate setting of priorities for research have been described, which aim to increase transparency, objectivity, acceptability, and validity of the results. To judge the merits of competing priorities, agreed criteria are needed. Information on expected cost, existing capacity, effect of the research, and effect on the population that is expected to benefit is also needed. Several techniques have been used, including the Delphi method (iterative broad consultation with a range of experts), trend analysis and modelling (forward extrapolation of historical data on the effect of certain research), scenario discussion (assessment of current priorities on the basis of a structured discussion of the potential outcomes), and matrix approaches (that use information on cost-effectiveness and other quantifiable data on the potential effect to direct the use of restricted resources). 5
We aimed to systematically summarise priority topics for tuberculosis research from available publications and to describe how priorities were identified. This systematic review will help to inform new initiatives for identifying and setting research priorities, such as for the recently established Research Movement of the Stop TB Partnership, which aims to increase the scope, scale, and speed of tuberculosis research and to ensure that research priorities are identified and properly funded.
Search strategy and selection criteria
We searched PubMed for studies published from 1998 to June 5, 2010, with the terms: (1) tuberculosis[tiab] OR mycobacter*[ti] OR (tuberculosis/epidemiology/prevention and control); (2) (research[ti] AND agend*[ti]) OR (research[ti] AND priorit*[ti]) OR (research[ti] AND need[ti]) OR (resource allocation/organisation and administration) OR (health services needs and demand) OR (research support); and (3) #1 AND #2. We also included publications cited in the documents when relevant. We contacted representatives of Stop TB Partnership and WHO to identify potentially relevant documents, especially for articles that might not have been indexed in PubMed; we also accessed information from the TB Research Movement collection . We did not use any restrictions for the language of the published studies. We excluded primary research and individual systematic reviews of specific interventions or topics. We also excluded papers that reprinted research priorities identified in previous studies.
Data abstraction and synthesis
The search results were screened by two authors (JR and PG) independently; documents were included if there was reference to research prioritisation or research topics and specific mention of tuberculosis in the abstract. Discordant decisions were resolved by consensus.
Articles were classified into two types: consensus statements from a convened group or expert panel or reviews or commentaries on the state of tuberculosis research that mentioned priorities or agendas for future work. We noted the affiliation of authors and investigated the methods used to reach conclusions. We identified the most frequently recurring questions and areas of interest. Details were extracted and coded for each article and for each question highlighted. Data were coded according to a piloted list of summary categories to enable analysis. Data extraction was done by JR and PG, and results were directly compared for verification and entered into Microsoft Access.
1004 articles were screened and 51 were shortlisted for full-text review ( figure ). 18 papers were excluded (webappendix); 33 were included. 6 , 7 , 8 , 9 , 10 , 11 , 12 , 13 , 14 , 15 , 16 , 17 , 18 , 19 , 20 , 21 , 22 , 23 , 24 , 25 , 26 , 27 , 28 , 29 , 30 , 31 , 32 , 33 , 34 , 35 , 36 , 37 , 38 12 of 33 articles were consensus statements; 6 , 8 , 13 , 20 , 21 , 22 , 27 , 28 , 29 , 30 , 31 , 32 11 of which were published in the past 5 years. WHO published three overviews of research priorities derived from expert consensus meetings held in 2005 with a wide range of questions. One focused on tuberculosis and HIV, 21 one assessed tuberculosis more broadly, 22 and the 2009 tuberculosis treatment guidelines 28 contributed further suggestions for research arising from a series of systematic reviews. Another multidisciplinary international working group (the International Standards for TB Care steering committee) produced standards for tuberculosis care directed at healthcare providers that are applicable worldwide. As part of this process, the authors derived a set of research priorities covering clinical case management, treatment and monitoring, and operational research. 20
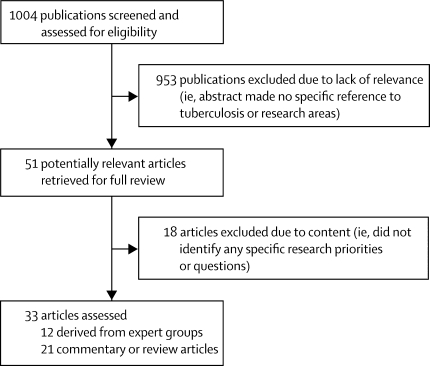
Flow chart of study selection
Three consensus statements highlighted and proposed research priorities for multidrug-resistant (MDR) tuberculosis. A consensus statement on MDR tuberculosis was published by the Stop TB Partnership's working group on MDR tuberculosis. 13 The research subgroup of the working group on MDR tuberculosis produced a document in which they investigated the scale-up of programmatic management of MDR tuberculosis and related research priorities. 8 A collaboration of European scientific academies made recommendations for work on MDR tuberculosis funded by the European Union. 29 A further three consensus statements came from funding agencies, one in the USA and two from Europe. The US statement, from the National Institute of Allergy and Infectious Disease, described current and future plans for research on MDR tuberculosis funded through the National Institutes of Health. 27 A group of authors of European Commission-funded projects produced a prioritisation programme for research in neglected infectious diseases, which made recommendations for tuberculosis research, 6 and another article described the current and European Commission-funded tuberculosis research portfolio with recommendations for future direction of finances. 30 The European Commission also supported another workshop on vaccine adjuvant research priorities. 31 Lastly, a broadly drawn expert group published a paper on how to investigate the joint burden of diabetes mellitus and tuberculosis. 32
The other 21 articles were reviews and commentaries; six focused on tuberculosis in children, 9 , 12 , 14 , 25 , 26 , 33 whereas the rest were not age specific. Three articles were related specifically to HIV, 7 , 25 , 34 and two to MDR tuberculosis. 15 , 35 Seven articles summarised the broad state and priorities of tuberculosis research. Specific topics addressed were drug treatments, 11 , 14 , 26 , 37 diagnostics, 19 , 23 , 25 , 36 preventive therapy, 7 health service limitations, 16 funding, 17 and design of clinical trials for tuberculosis drugs. 35
Table 1 provides a summary of the methods used to develop the research priorities in the studies. 13 articles were derived from the questions from an expert meeting. Of these, three stated that expert advice was sought beyond the panel. Two of the consensus groups reported inclusion of representation of patients or communities. One article reported a systematic review of relevant evidence with a specific search strategy. 9 Two groups seemed to collate data from primary research articles comprehensively, although they did not state their strategy. 7 , 36 Most articles (27 of 33) presented selected details from primary literature in conventional narrative review format, and gave no details of search terms, indexing databases, or inclusion and exclusion criteria. 6 , 7 , 10 , 11 , 12 , 13 , 15 , 16 , 17 , 18 , 19 , 20 , 21 , 22 , 23 , 24 , 25 , 26 , 27 , 29 , 30 , 31 , 32 , 33 , 34 , 35 , 38
Summary of methods used to develop research priorities for tuberculosis
Synthesis refers to a systematic analysis of primary research.
External refers to external advice to the authors or consensus panel members.
Research areas highlighted in the studies were identified with various methods. Four of the 12 consensus statements used the opinion of expert subgroups or were derived from group discussion. 13 , 21 , 22 , 30 In three consensus papers, systematic reviews were commissioned to inform an expert group, 20 , 28 , 32 and the Stop TB Partnership working group on MDR tuberculosis used established WHO guidelines to critically appraise existing publications for gaps in knowledge. 8 The other four consensus articles gave no indication of how research areas were identified. 6 , 27 , 29 , 31 Commentary articles identified knowledge gaps by systematic review in three cases, 7 , 9 , 14 and three articles discussed selected results of existing systematic reviews. 19 , 25 , 36 Two reported on brainstorming exercises. 17 , 18 The rest of the commentary papers (13 of 21) did not specify the methods used and did not make use of systematic reviews.
Four of the consensus articles described some methods for ranking the priorities that they identified. 8 , 13 , 17 , 27 Two used meetings of experts subdivided into ad-hoc committees by research subject area; in one, the number of questions was limited to five; 13 and in the other, participants ranked the questions they had generated by perceived importance, and refined the list by wider expert consultation. 8 One article reported a seven-step analysis process that was used to compare research needs for the portfolio of diseases in the WHO Special Programme for Research and Training in Tropical Diseases. 17 This paper used an analysis of current and projected burden of disease, current control limitations, research gaps, and opportunities. No further details were available from the article on how these opportunities were identified. One article used internal and external advisers to estimate the relative potential of research areas to “contribute substantially to a global public health response”, 27 although it did not show which criteria were used to assess these relative potentials.
The number and type of research questions varied widely across the articles. Some articles provided many questions (ten suggested more than 20, of which only one group had attempted relative prioritisation)—277 question areas were identified. Research was specifically suggested for HIV-infected persons (63 questions), MDR tuberculosis (49), malnutrition (nine), and diabetes (seven). The mean number of research questions per article was 17 (range 1–78). Consensus statements had more questions on average (mean of 21 questions) than did reviews and opinion pieces (mean of 15 questions).
Research priorities were expressed differently across articles. Nine articles 9 , 10 , 12 , 18 , 20 , 22 , 24 , 30 , 33 took into account the wide remit of tuberculosis research in general, of which three 20 , 21 , 22 used expert subgroups within each area of interest. Most other articles were more focused, including five articles on MDR tuberculosis and drug development, 8 , 13 , 15 , 27 , 29 four on HIV co-infection, 7 , 21 , 25 , 34 and two on laboratory diagnostics and vaccination. 19 , 31 Single articles focused individually on health system research, 16 tuberculosis treatment guidelines, 28 tuberculosis and diabetes mellitus, 32 and design factors in drug trials. 35
Most articles identified treatment with drugs, drug development, and diagnostics as areas of research priority ( table 2 ). 17 articles identified questions relating specifically to children. With regard to HIV-infected populations, the most commonly cited questions related to antituberculous drug treatment regimens (16 articles), diagnosis (15), and epidemiology (7). The pattern for MDR tuberculosis was similar, with drug treatment (ten), diagnosis (11), and epidemiology (ten) highlighted. The panel lists the most common research areas highlighted for HIV co-infection and MDR tuberculosis. Systematic reviews were used more often for papers that had questions related to drug development, diagnostics, and epidemiology (five of nine articles that used these methods identified questions in these areas). By contrast, systematic reviews were only done in two articles that identified questions on basic science and vaccines.
Number of studies identifying priority topics for tuberculosis research
Questions most commonly in reference to children (four articles or more).
Panel. Common research areas about HIV co-infection and MDR tuberculosis.
Optimum tuberculosis treatment using existing drugs for HIV co-infection
Optimum duration of therapy (tuberculosis first-line drugs)
Role of intensive case-finding in communities with high prevalence of HIV
Studies of effectiveness of isoniazid as a preventive therapy
Pharmacokinetic interaction studies
Optimum role of co-trimoxazole in HIV co-infection
Best integration of tuberculosis and HIV services
Optimum time for initiation of antiretrovirals in tuberculosis and HIV co-infection
MDR tuberculosis
New diagnostics for drug sensitivity testing
Selection algorithms for drug sensitivity testing in existing programmes
Use of standardised regimens for MDR tuberculosis treatment
Efficacy studies of second-line drugs
Safety studies of second-line drugs
Pharmacokinetic and pharmacodynamic studies of second-line drugs
Chemoprophylactic regimens for those in contact with MDR tuberculosis-affected individuals
Burden of MDR tuberculosis
14 of the 33 articles were by authors who originated from academic institutions, ten were by members of WHO or Stop TB Partnership (often jointly), and nine were by authors from non-governmental organisations (five), governmental or funding agencies (three), and a science advisory body (one). 223 individuals were named as authors. Authors were most commonly affiliated with academic institutions, followed by WHO, and government staff ( table 3 ). Representatives of funding organisations did not commonly co-author the articles (two). Patients were directly represented in two cases, both for papers on treatment guidelines. 40 individuals were listed as authors on more than one of the included articles; 12 contributed to more than two; and five contributed to more than three. Authorship of more than one article was most common for authors from universities (41%) and those affiliated with WHO and Stop TB Partnership (21%). The mean number of authors per article was nine (range 1–28); for consensus statements, the mean number of authors was 15 (range 2–28), and for reviews the mean was five (range 1–14).
Author affiliations (n=223)
Author numbers from these organisations are combined.
Authors from non-governmental organisations (18), national control programmes (nine), private sector workers (four), professional medical organisations (four), clinicians (four), funding organisations (two), medical students (one), activists for patients (two), or unknown (one). TDR=Special Programme for Research and Training in Tropical Diseases.
20 of the articles included a statement of conflicts of interest. In three articles, the author's affiliation indicated that publication would promote an organisation's interests, but no conflict of interest statement was specifically published. 10 , 23 , 24 There is, however, some evidence of a recent change in practice—in articles published between 1998 and 2005, one of six articles included a statement; from 2006 to 2010, 19 of 27 did so.
We identified 33 research agendas for tuberculosis published between 1998 and 2010. Two clear research priorities emerged from this systematic review: the development and testing of both new drugs and treatment regimens, and new diagnostic tests for tuberculosis. These areas also dominated when focusing on the research needs in specific populations such as patients co-infected with HIV or patients with MDR tuberculosis, who are particularly affected by the limitations of current drugs and diagnostics. This finding is indicative of the inefficiencies of the currently used sputum-smear-based diagnosis in many cases and of the observation that short-course chemotherapy, despite 95% efficacy in clinical trials, has not substantially contributed to decreasing the transmission of tuberculosis in areas with high HIV burden nor been effective for patients with drug-resistant tuberculosis. 39 , 40
The use of epidemiology in these studies as a means to better understand the factors involved in the worldwide burden of disease and to assess the effect of case-finding was notable. The frequent inclusion of epidemiology emphasises the importance of doing studies at the population level to better target control interventions and possibly highlights a perception that accurately documenting the burden of disease might help to advocate allocation of resources for research. Epidemiological and impact-assessment studies are also necessary for monitoring and evaluation and to check if the Stop TB Partnership strategy is actually effective in controlling tuberculosis. 41 Research into health services was also identified to be a common priority; this emphasis on operational research might be indicative of the need to optimise the availability and cost-effectiveness of techniques for improved tuberculosis control at the programme level in resource-limited settings.
Investigation of basic science questions aimed at contributing to the research and development pipeline was less commonly identified as a priority. Fundamental research on prominent fields, such as the biology of Mycobacterium tuberculosis , the host-pathogen interactions, and the latency and persistence patterns, is essential for the development of new diagnostic tests and drugs. 24 Similarly, this research should support work on vaccine development, which was also relatively less prioritised than are other research areas.
According to a Treatment Action Group report on tuberculosis research and development funding, investments in tuberculosis research and development in 2007 were mostly in drug development (US$170 million, 35%), basic science ($121 million, 25%), and vaccine development ($71 million, 15%), compared with diagnostics ($41 million, 9%) and operational research ($36 million, 8%). 3 One potential explanation for this apparent difference in allocation of funds is that, in the research topics reviewed here, the relative importance of drugs and diagnosis compared with basic and operation research is probably indicative of the difficulty of establishing clear research agendas in these areas rather than an absence of perception of priority. Basic research is mostly driven by curiosity and might therefore not benefit from fitting into specific topic areas that could be perceived as restrictive or limited. Because operational research is closely linked to programme implementation, this research falls comparatively short of funding and seems to be more difficult to prioritise in definite research agendas.
Use of systematic reviews to help establish tuberculosis research priorities is uncommon. Systematic reviews might not always be necessary; for example, because research in that area is recent. However, the use of systematic review has received growing attention in recent years and is increasingly being recognised as an important approach to the assessment of research 42 by providing an inventory on what is known.
Although there are formal methods for prioritising research, few of the consensus groups used them. Group discussion methods tended to provide large comprehensive lists of questions without prioritisation, such as the report by WHO and the Special Programme for Research and Training in Tropical Diseases report on tuberculosis. 22 Listing of questions might help in reaching a consensus in a large diverse group, but is less helpful in targeting strategic areas or in deciding on research funding allocation. This approach might be useful, however, for raising awareness and for funding in specific research domains. The consensus statement on short-course directly observed treatment for MDR tuberculosis, by contrast, was able to distil the list of identified topics into focused, prioritised research questions. 13 Such an approach might be more useful in guiding scientists and funding agencies.
The pool of academics and specialists involved in these articles was relatively small and many authors contributed to more than one document. There was little representation for patients. Although we do not know what effect they would have on priorities, involvement of patients is likely to help focus research on outcomes that interest those with disease. Collaboration with patients is valuable to researchers, clinicians, and funding organisations, and is well documented in other areas. 43 Additionally, conflicts of interest were not stated in many articles. Disclosure of conflicts of interest should be universally implemented, as has recently been adopted for expert meetings at WHO.
Although care was taken to include a comprehensive search of papers, some notable documents might have been overlooked. We studied the methods used to identify and recommend research priorities. However, this study does not answer an important question for researchers: which of the research questions is supported by high-quality evidence? We expect that by improving prioritisation methods, this question could be answered.
To identify key research questions within specific areas, various people are involved to make decisions about need and scientific opportunities. This process needs a thorough understanding of existing work and a critical analysis of existing data through systematic review where appropriate. We believe that transparent and specific processes are important in setting research priorities. Establishing these priorities would be enhanced by a structured synthesis of existing knowledge, such as that advocated by the Grading of Recommendations Assessment, Development and Evaluation (GRADE) 44 approach.
Consensus panels understandably tend to favour discussion and critical analysis to reach agreement, but generally fail to suggest priorities. Formal methods to assess the relative merit of priority areas should be more widely adopted. This assessment would involve establishing clear criteria by which to judge the importance of the research questions, such as: the potential for progress, the public health need, and the potential effect on public health. 45 Such an approach was adopted for research in childhood diseases. 46 Criteria for establishing research priorities were the following: likelihood that the research question would be answerable in an ethical way; likelihood that the resulting intervention would be effective in reducing disease burden; deliverability, affordability, and sustainability of the resulting intervention; maximum potential of the intervention to reduce disease burden; and effect of disease burden reduction on equity in the population. In some approaches, a system of voting enables large groups of panel members to reach a consensus agreement. This system depends on participants being adequately informed of the existing knowledge base, such as by systematic review. Additionally, panels need to engage representatives of patients and have transparent systems for expressing potential conflicts of interest. Journals should also be consistent in requiring statements about potential conflicts of interest.
The identified research topics indicate the central role of WHO and the Stop TB Partnership in establishing guidelines and advocating for better control of tuberculosis worldwide. These organisations have recently jointly launched the TB Research Movement, which will engage many tuberculosis researchers in a collaborative strategic effort to increase the scope, scale, and speed of research to accelerate progress in worldwide control of tuberculosis.
The research areas frequently identified and summarised here should help to provide a platform for explicit development of a transparent and widely approved system for the establishment of priorities for tuberculosis research, using specific criteria and systematic reviews combined with expert opinion. Such an approach would identify knowledge gaps, inform funding organisations' decisions, and ensure that research is harmonised and effective. All these steps are crucial to improving worldwide control of tuberculosis.
Acknowledgments
PG is supported by a project funded by the UK Department for International Development for the benefit of developing countries. The views expressed here are not necessarily those of the Department for International Development. JR is funded by a Wellcome Trust to promote health priorities in developing countries. MP is supported by a New Investigator Award from the Canadian Institutes of Health Research.
Research areas were identified in more than three articles. MDR=multi-drug resistant.
Contributors.
MP, PG, and CL initiated the paper. JR and PG undertook the literature searches and extracted data, and wrote the draft manuscript. MP and CL provided suggestions, helped to identify relevant studies, and contributed fully to the revisions of the paper. All authors approved the final version.
Conflicts of interest
CL is responsible for the TB Research Movement at the Stop TB Partnership and WHO. MP serves as chair of the Task Force of the TB Research Movement and as a co-chair of the Stop TB Partnership new diagnostics working group; all groups are involved in promoting new diagnostics for tuberculosis. All other authors have received funding from WHO.
Web Extra Material
- 1. WHO . Global tuberculosis control: a short update to the 2009 report. WHO/HTM/TB/2009426. World Health Organization; Geneva: 2009. http://whqlibdoc.who.int/publications/2009/9789241598866_eng.pdf (accessed July 1, 2010). [ Google Scholar ]
- 2. WHO and Special Programme for Research and Training in Tropical Diseases (TDR) Priority setting methodologies in health research. World Health Organization; Geneva: April 10–11, 2008. http://apps.who.int/tdr/stewardship/pdf/Priority_setting_Workshop_Summary10_04_08.pdf (accessed July 1, 2010). [ Google Scholar ]
- 3. Agarwal N. Tuberculosis research and development: a critical analysis of funding trends, 2005–2007 an update. Treatment Action Group; New York: March, 2009. http://www.treatmentactiongroup.org/uploadedFiles/About/Publications/TAG_Publications/2009/TAG%20TB%202009%20web-version2.pdf (accessed July 1, 2010). [ Google Scholar ]
- 4. Stop TB Partnership and WHO . The global plan to stop TB, 2006–2015. Stop TB Partnership and World Health Organization; Geneva: 2006. http://www.stoptb.org/assets/documents/global/plan/GlobalPlanFinal.pdf (accessed Aug 28, 2009). [ Google Scholar ]
- 5. Council on Health Research for Development (COHRED) Priority setting for health research: toward a management process for low and middle income countries. COHRED; 2006. http://www.cohred.org/sites/default/files/WP1_PrioritySetting.pdf (accessed June 17, 2009). [ Google Scholar ]
- 6. Boraschi D, Abebe Alemayehu M, Aseffa A. Immunity against HIV/AIDS, malaria, and tuberculosis during co-infections with neglected infectious diseases: recommendations for the European Union research priorities. PLoS Negl Trop Dis. 2008;2:e255. doi: 10.1371/journal.pntd.0000255. [ DOI ] [ PMC free article ] [ PubMed ] [ Google Scholar ]
- 7. Churchyard GJ, Scano F, Grant AD, Chaisson RE. Tuberculosis preventive therapy in the era of HIV infection: overview and research priorities. J Infect Dis. 2007;196:S52–S62. doi: 10.1086/518662. [ DOI ] [ PubMed ] [ Google Scholar ]
- 8. Cobelens FG, Heldal E, Kimerling ME. Scaling up programmatic management of drug-resistant tuberculosis: a prioritized research agenda. PLoS Med. 2008;5:e150. doi: 10.1371/journal.pmed.0050150. [ DOI ] [ PMC free article ] [ PubMed ] [ Google Scholar ]
- 9. Donald PR, Maher D, Qazi S. A research agenda to promote the management of childhood tuberculosis within national tuberculosis programmes. Int J Tuberc Lung Dis. 2007;11:370–380. [ PubMed ] [ Google Scholar ]
- 10. Ginsberg AM. The tuberculosis epidemic. Scientific challenges and opportunities. Public Health Rep. 1998;113:128–136. [ PMC free article ] [ PubMed ] [ Google Scholar ]
- 11. Ginsberg AM, Spigelman M. Challenges in tuberculosis drug research and development. Nat Med. 2007;13:290–294. doi: 10.1038/nm0307-290. [ DOI ] [ PubMed ] [ Google Scholar ]
- 12. Graham SM, Gie RP, Schaaf HS, Coulter JB, Espinal MA, Beyers N. Childhood tuberculosis: clinical research needs. Int J Tuberc Lung Dis. 2004;8:648–657. [ PubMed ] [ Google Scholar ]
- 13. Gupta R, Espinal M. A prioritised research agenda for DOTS-Plus for multidrug-resistant tuberculosis (MDR-TB) Int J Tuberc Lung Dis. 2003;7:410–414. [ PubMed ] [ Google Scholar ]
- 14. Hill S, Regondi I, Grzemska M, Matiru R. Children and tuberculosis medicines: bridging the research gap. Bull World Health Organ. 2008;86:658. doi: 10.2471/BLT.08.056804. [ DOI ] [ PMC free article ] [ PubMed ] [ Google Scholar ]
- 15. Mitnick CD, Castro KG, Harrington M, Sacks LV, Burman W. Randomized trials to optimize treatment of multidrug-resistant tuberculosis. PLoS Med. 2007;4:e292. doi: 10.1371/journal.pmed.0040292. [ DOI ] [ PMC free article ] [ PubMed ] [ Google Scholar ]
- 16. Nunn P, Harries A, Godfrey-Faussett P, Gupta R, Maher D, Raviglione M. The research agenda for improving health policy, systems performance, and service delivery for tuberculosis control: a WHO perspective. Bull World Health Organ. 2002;80:471–476. [ PMC free article ] [ PubMed ] [ Google Scholar ]
- 17. Remme JH, Blas E, Chitsulo L. Strategic emphases for tropical diseases research: a TDR perspective. Trends Parasitol. 2002;18:421–426. doi: 10.1016/s1471-4922(02)02387-5. [ DOI ] [ PubMed ] [ Google Scholar ]
- 18. Onyebujoh P, Rodriguez W, Mwaba P. Priorities in tuberculosis research. Lancet. 2006;367:940–942. doi: 10.1016/S0140-6736(06)68385-2. [ DOI ] [ PubMed ] [ Google Scholar ]
- 19. Pai M, Dheda K, Cunningham J, Scano F, O'Brien R. T-cell assays for the diagnosis of latent tuberculosis infection: moving the research agenda forward. Lancet Infect Dis. 2007;7:428–438. doi: 10.1016/S1473-3099(07)70086-5. [ DOI ] [ PubMed ] [ Google Scholar ]
- 20. Tuberculosis Coalition for Technical Assistance International Standards for Tuberculosis Care (ISTC). The Hague: Tuberculosis Coalition for Technical Assistance. 2006. http://www.who.int/tb/publications/2006/istc_report.pdf (accessed Aug 28, 2008).
- 21. WHO . TB/HIV research priorities in resource-limited settings: report of an expert consultation. WHO; Geneva: Feb 14–15, 2005. http://www.who.int/hiv/pub/tb/tbhivresearchpriorities.pdf (accessed Aug 28, 2008). [ Google Scholar ]
- 22. WHO . Report of the scientific working group meeting on tuberculosis. WHO and The Special Programme for Research and Training in Tropical Diseases; Geneva: Oct 3–6, 2006. http://apps.who.int/tdr/publications/tdr-research-publications/swg-report-tuberculosis/pdf/swg_tub.pdf (accessed Nov 11, 2008). [ Google Scholar ]
- 23. Perkins MD, Roscigno G, Zumla A. Progress towards improved tuberculosis diagnostics for developing countries. Lancet. 2006;367:942–943. doi: 10.1016/S0140-6736(06)68386-4. [ DOI ] [ PubMed ] [ Google Scholar ]
- 24. Chaisson RE, Harrington M. How research can help control tuberculosis. Int J Tuberc Lung Dis. 2009;13:558–568. [ PubMed ] [ Google Scholar ]
- 25. Hesseling AC, Cotton MF, Marais BJ. BCG and HIV reconsidered: moving the research agenda forward. Vaccine. 2007;25:6565–6568. doi: 10.1016/j.vaccine.2007.06.045. [ DOI ] [ PubMed ] [ Google Scholar ]
- 26. Burman WJ, Cotton MF, Gibb DM, Walker AS, Vernon AA, Donald PR. Ensuring the involvement of children in the evaluation of new tuberculosis treatment regimens. PLoS Med. 2008;5:e176. doi: 10.1371/journal.pmed.0050176. [ DOI ] [ PMC free article ] [ PubMed ] [ Google Scholar ]
- 27. Fauci AS. Multidrug-resistant and extensively drug-resistant tuberculosis: the National Institute of Allergy and Infectious Diseases research agenda and recommendations for priority research. J Infect Dis. 2008;197:1493–1498. doi: 10.1086/587904. [ DOI ] [ PubMed ] [ Google Scholar ]
- 28. WHO . Treatment of tuberculosis guidelines. 4th edn. World Health Organization; Geneva: 2009. http://whqlibdoc.who.int/publications/2010/9789241547833_eng.pdf (accessed June 11, 2010). [ PubMed ] [ Google Scholar ]
- 29. Fears R, Kaufmann S, Ter Meulen V, Zumla A, EASAC Working Group Drug-resistant tuberculosis in the European Union: opportunities and challenges for control. Tuberculosis. 2010;90:182–187. doi: 10.1016/j.tube.2010.03.008. [ DOI ] [ PubMed ] [ Google Scholar ]
- 30. Lang H, Quaglio G, Olesen OF. Tuberculosis research in the European union: past achievements and future challenges. Tuberculosis. 2010;90:1–6. doi: 10.1016/j.tube.2009.10.002. [ DOI ] [ PubMed ] [ Google Scholar ]
- 31. Harandi AM, Medaglini D, Shattock RJ. Vaccine adjuvants: a priority for vaccine research. Vaccine. 2010;28:2363–2366. doi: 10.1016/j.vaccine.2009.12.084. [ DOI ] [ PubMed ] [ Google Scholar ]
- 32. Harries AD, Murray MB, Jeon CY. Defining the research agenda to reduce the joint burden of disease from diabetes mellitus and tuberculosis. Trop Med Int Health. 2010;15:659–663. doi: 10.1111/j.1365-3156.2010.02523.x. [ DOI ] [ PMC free article ] [ PubMed ] [ Google Scholar ]
- 33. Swaminathan S, Rekha B. Pediatric tuberculosis: global overview and challenges. Clin Infect Dis. 2010;50(suppl 3):S184–S194. doi: 10.1086/651490. [ DOI ] [ PubMed ] [ Google Scholar ]
- 34. Chamie G, Luetkemeyer A, Charlebois E, Havlir DV. Tuberculosis as part of the natural history of HIV infection in developing countries. Clin Infect Dis. 2010;50(suppl 3):S245–S254. doi: 10.1086/651498. [ DOI ] [ PMC free article ] [ PubMed ] [ Google Scholar ]
- 35. Lienhardt C, Davies G. Methodological issues in the design of clinical trials for the treatment of multidrug-resistant tuberculosis: challenges and opportunities. Int J Tuberc Lung Dis. 2010;14:528–537. [ PubMed ] [ Google Scholar ]
- 36. Pai M, Minion J, Steingart K, Ramsay A. New and improved tuberculosis diagnostics: evidence, policy, practice, and impact. Curr Opin Pulm Med. 2010;16:271–284. doi: 10.1097/MCP.0b013e328338094f. [ DOI ] [ PubMed ] [ Google Scholar ]
- 37. Ma Z, Lienhardt C, McIlleron H, Nunn AJ, Wang X. Global tuberculosis drug development pipeline: the need and the reality. Lancet. 2010;375:2100–2109. doi: 10.1016/S0140-6736(10)60359-9. [ DOI ] [ PubMed ] [ Google Scholar ]
- 38. Marais BJ, Raviglione MC, Donald PR. Scale-up of services and research priorities for diagnosis, management, and control of tuberculosis: a call to action. Lancet. 2010;375:2179–2191. doi: 10.1016/S0140-6736(10)60554-5. [ DOI ] [ PubMed ] [ Google Scholar ]
- 39. Dye C, Williams BG. Eliminating human tuberculosis in the twenty-first century. J R Soc Interface. 2008;5:653–662. doi: 10.1098/rsif.2007.1138. [ DOI ] [ PMC free article ] [ PubMed ] [ Google Scholar ]
- 40. Dowdy DW, Chaisson RE. The persistence of tuberculosis in the age of DOTS: reassessing the effect of case detection. Bull World Health Organ. 2009;87:296–304. doi: 10.2471/BLT.08.054510. [ DOI ] [ PMC free article ] [ PubMed ] [ Google Scholar ]
- 41. Stop TB, WHO . TB impact measurement: policy and recommendations for how to assess the epidemiological burden of TB and the impact of TB control. World Health Organization; Geneva: 2009. [ Google Scholar ]
- 42. Lavis JN. How can we support the use of systematic reviews in policymaking? PLoS Med. 2009;6:e1000141. doi: 10.1371/journal.pmed.1000141. [ DOI ] [ PMC free article ] [ PubMed ] [ Google Scholar ]
- 43. Stewart R, Oliver S. A systematic map of studies of patients' and clinicians' research priorities. James Lind Alliance; London: 2008. http://www.lindalliance.org/pdfs/JLA%20Internal%20Reports/090712_JLAreport_RS_map_studies_PPI%20&%20Clinicians_research%20priorities_with%20appendices.pdf (accessed June 29, 2010). [ Google Scholar ]
- 44. Guyatt GH, Oxman AD, Vist GE. GRADE: an emerging consensus on rating quality of evidence and strength of recommendations. BMJ. 2008;336:924–926. doi: 10.1136/bmj.39489.470347.AD. [ DOI ] [ PMC free article ] [ PubMed ] [ Google Scholar ]
- 45. Normand C. Ten popular health economic fallacies. J Public Health Med. 1998;20:129–132. doi: 10.1093/oxfordjournals.pubmed.a024730. [ DOI ] [ PubMed ] [ Google Scholar ]
- 46. Rudan I, El Arifeen S, Black RE, Campbell H. Childhood pneumonia and diarrhoea: setting our priorities right. Lancet Infect Dis. 2007;7:56–61. doi: 10.1016/S1473-3099(06)70687-9. [ DOI ] [ PubMed ] [ Google Scholar ]
Associated Data
This section collects any data citations, data availability statements, or supplementary materials included in this article.
Supplementary Materials
- View on publisher site
- Collections
Similar articles
Cited by other articles, links to ncbi databases.
- Download .nbib .nbib
- Format: AMA APA MLA NLM
Add to Collections
- Frontiers in Tuberculosis
- Epidemiology of Tuberculosis
- Research Topics
Community Insights in Tuberculosis Epidemiology
Total Downloads
Total Views and Downloads
About this Research Topic
Frontiers in Tuberculosis has organized this Research Topic to highlight the latest advancements in research across the field of tuberculosis. This editorial initiative is focused on new insights, novel developments, current challenges, latest discoveries, recent advances, and future perspectives in the field of tuberculosis epidemiology. Contributors from various fields are encouraged to submit their work, with a focus on epidemiologic methods and data-driven actions. Manuscripts should contribute to TB epidemiologic understanding, or demonstrate the public health impact of tuberculosis interventions. Please see the section description here for further information on the scope of articles accepted. The goal of this special edition Research Topic is to further our understanding of tuberculosis transmission, infection, and progression, to disseminate best practices for tuberculosis control and prevention, and to identify future challenges to provide a thorough overview of the field.
Keywords : Tuberculosis Epidemiology, Tuberculosis control and prevention, Epidemiologic methods, Disease burden
Important Note : All contributions to this Research Topic must be within the scope of the section and journal to which they are submitted, as defined in their mission statements. Frontiers reserves the right to guide an out-of-scope manuscript to a more suitable section or journal at any stage of peer review.
Topic Editors
Topic coordinators, submission deadlines, participating journals.
Manuscripts can be submitted to this Research Topic via the following journals:
total views
- Demographics
No records found
total views article views downloads topic views
Top countries
Top referring sites, about frontiers research topics.
With their unique mixes of varied contributions from Original Research to Review Articles, Research Topics unify the most influential researchers, the latest key findings and historical advances in a hot research area! Find out more on how to host your own Frontiers Research Topic or contribute to one as an author.

A .gov website belongs to an official government organization in the United States.
A lock ( ) or https:// means you've safely connected to the .gov website. Share sensitive information only on official, secure websites.
- Signs and Symptoms
- Causes and Spread
- Testing for Tuberculosis
- Risk Factors
- Clinical Overview
- Clinical Signs and Symptoms
- Bacille Calmette-Guérin (BCG) Vaccine
- Clinical Testing and Diagnosis
- Mantoux Tuberculin Skin Test Toolkit
- Health Care Provider Communication and Education Resources
- ICD-10-CM Codes for TB
- Tuberculosis Case Reporting
- Guidance for Public Health Professionals
- Laboratory Information
- False-Positive Investigation Toolkit
- Tuberculosis Genotyping
- Dear Colleague Letters
- MDDR Request Form
- TB Programs
Related Topics:
- View All Home
- TB Prevention in Healthcare Settings
- Information for Tuberculosis Programs
Health Disparities in Tuberculosis
- Some groups of people have an increased risk of becoming infected with TB germs due to higher rates of active TB disease in their communities.
- Many social, economic, and demographic factors affect a person's risk for TB.
- CDC works to address disparities in TB in the United States.
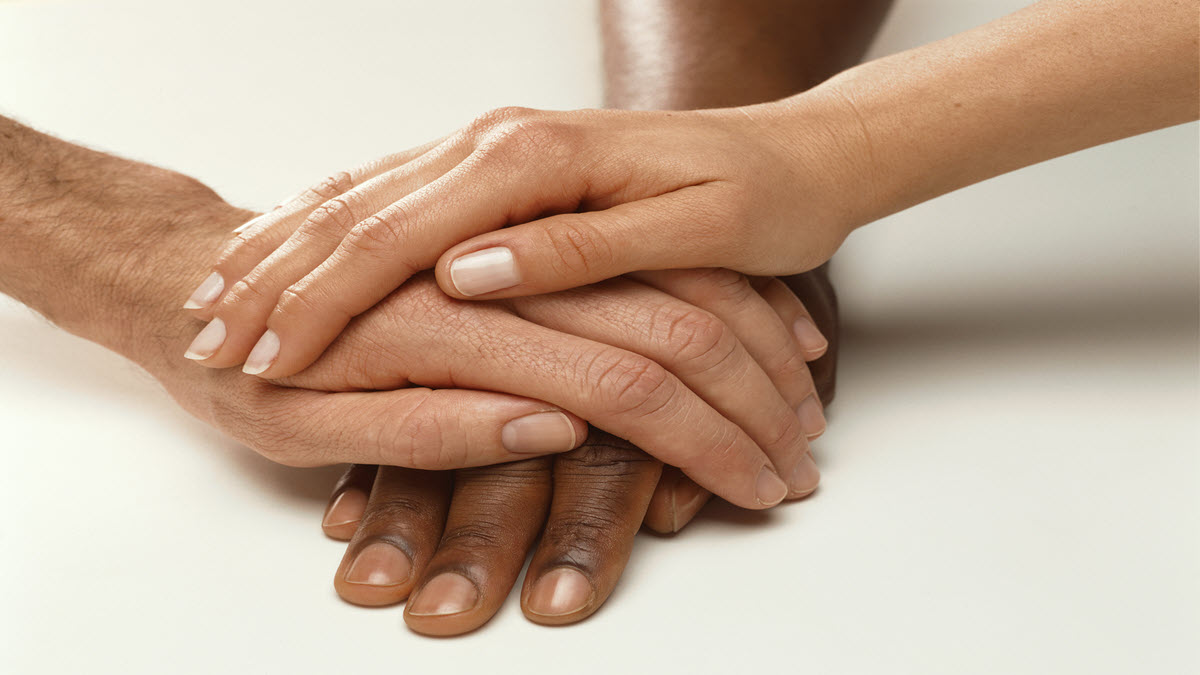
What is a health disparity?
Despite prevention efforts, TB affects some groups of people more than others.
A health disparity is the occurrence of diseases at greater levels among certain population groups more than among others. Differences in rates of diseases, like TB, may occur by race or ethnicity, health conditions, and geographic location.
Social determinants of health are the non-medical factors that influence health outcomes. They are the conditions in which people are born, grow, work, live, and age, and the wider set of forces and systems shaping the conditions of daily life. Social determinants of health like poverty, unequal access to health care, lack of education, stigma, and racism are linked to health disparities. For example, stigma around TB may deter people from seeking medical care or follow-up care if they are sick.
Health disparities
Some population groups have higher rates of TB disease in their communities. These groups are at higher risk of being exposed to TB germs.
Health disparities and TB
Additionally, a range of social, economic, and demographic factors affect a person’s risk for TB disease.
Racial and ethnic disparities
In 2022 , 88.4% of the TB cases reported in the United States occurred among racial and ethnic minority groups.
In the United States, TB affects some racial and ethnic minority groups more than others, including:
- Asian persons
- Black or African American persons
- Hispanic or Latino persons
Medicines or health conditions
Certain medicines or health conditions contribute to disparities in the rate of TB. Health conditions such as diabetes , cancer, or HIV infection weaken the body's immune system. A weakened immune system lowers a person's ability to fight TB germs. As a result, people with these health conditions are more likely to develop TB disease if they are infected with TB germs.
Geographic disparities
United states.
TB disease rates vary across the United States. Consistent with previous years, in 2022 , four states accounted for half of all reported U.S. TB cases:
- New York (including New York City)
Around the world
TB disease rates also vary around the world. Because of the substantially greater risk of exposure to TB germs outside of the United States, people who were born in or frequently travel to countries where TB is more common are more likely to be exposed to TB germs. In 2022 , the TB incidence rate was 17.1 times higher among non-U.S.–born persons compared with U.S.-born persons.
Other disparities
People experiencing homelessness and people who live or work in correctional facilities are at increased risk for TB.
What prevents health equity
TB is a challenging disease to diagnose, treat, and control. Dwindling resources and loss of public health capacity, including access to care, and maintaining clinical and public health expertise, add to the challenge.
Communities affected by TB disparities face a number of challenges.
Treatment duration and completion
TB treatment takes several months to complete. Some people may not be able to or want to take TB medicines for several months.
People with TB disease who do not take their medicine correctly could:
- Get sick with TB disease again
- Spread TB to others
- Develop drug-resistant TB
People with inactive TB (also called latent TB infection) do not feel sick , do not have symptoms, and cannot spread TB germs to others. It might not be a priority to treat inactive TB since they do not feel sick.
Socioeconomic factors
Socioeconomic factors can directly or indirectly increase a person's risk for TB disease and make treatment difficult. These factors include:
- Quality health care,
- Employment opportunities,
- Transportation, and
- Adverse health outcomes.
Language barriers and cultural factors may also place certain populations at higher risk for TB. These include factors such as:
- Health knowledge,
- Stigma associated with the disease,
- Values, and
What CDC is doing
CDC is committed to improving the health of people disproportionately affected by TB by identifying the underlying causes of health disparities in TB and developing strategies to improve health equity.
Partnerships
- Collaborate with other national and international public health organizations to improve TB control and prevention activities among immigrants and refugees.
- Support the TB Elimination Alliance to increase knowledge, testing, and treatment of TB among communities at increased risk.
- Provide assistance through the TB Centers of Excellence for Training, Education, and Medical Consultation , which support TB control and prevention efforts in the United States
- Engage with domestic partners such as, We Are TB , a community of TB survivors, people being treated for TB, and their family members, working together to eliminate TB.
Communication and outreach
- Maintain a Spanish TB website that provides Spanish-language TB information.
- Develop culturally and linguistically appropriate patient education materials, and share through platforms like Find TB Resources .
- Those most at risk for inactive TB and
- Their health care providers to encourage TB testing and treatment.
- Highlight the personal stories of people who were diagnosed and treated for TB, as well as the work of TB control professionals.
- Engage health care providers about the latest TB diagnostics and treatment options available.
- Lead community engagement and outreach to engage communities at risk to raise awareness of TB.
- Compile national reports of TB cases and TB case rates by gender, race and ethnicity, risk factors, and geographic location.
- Examine more effective TB treatment options and
- Study the risks for TB among persons with certain medical conditions.
Materials are available free of charge for order via CDC-Info On Demand Publications ( see ordering instructions ).
Tuberculosis (TB)
Tuberculosis is caused by bacteria called Mycobacterium tuberculosis . The bacteria usually attack the lungs but can attack any part of the body.
For Everyone
Health care providers, public health.

COMMENTS
Tuberculosis is the leading cause of death from a single infectious agent, with over 25% of these occurring in the African region. Multi-drug resistant strains which do not respond to first-line ...
Tuberculosis (TB) research and innovation is essential to achieve the global TB targets of the United Nations (UN) Sustainable Development Goals (SDGs) and the World Health Organization (WHO) End TB Strategy. The SDG target is to "end the epidemic" by 2030; more specific targets for 2030 set in the End TB Strategy are a 90% reduction in TB ...
Introduction. Tuberculosis (TB) continues to pose a major threat to global health , and research is a key component of the Global Plan to Stop TB2011-2015 .Research is particularly critical for developing new tools and approaches needed for eliminating TB by 2050 .Recognizing this, the Stop TB Partnership and the World Health Organization's (WHO) Stop TB Department have launched the TB ...
A multidisciplinary journal focused on understanding the mechanisms behind the development, progression, treatment of tuberculosis and non-tuberculous mycobacterial infections. ... Research Topics. Submission open Community Insights in Tuberculosis Epidemiology. Isdore Chola Shamputa; Raquel Duarte Bessa De Melo;
Tuberculosis research. Tuberculosis is the leading cause of death from a single infectious agent and remains a global health emergency. In 2018 alone, there were 1.5 million deaths and 10 million new cases globally, among whom half a million had rifampicin resistant TB. The annual rate of decline in TB incidence is still much lower than what ...
Abstract. Tuberculosis (TB) is a major cause of morbidity and mortality worldwide. It is estimated that 25% of the world's population are infected with Mycobacterium tuberculosis, with a 5-10% lifetime risk of progression into TB disease.Early recognition of TB disease and prompt detection of drug resistance are essential to halting its global burden.
A structural problem. If we consider the current epidemiological picture, the good news is that mortality from TB is declining (Fig. 1 A), but the reduction in deaths is mainly attributable to antiretroviral treatment of patients coinfected with TB and HIV.Tragically, the incidence of TB hasn't changed in decades (Fig. 1 B).The overarching finding of the World Health Organization (WHO) report ...
Tuberculosis (TB) research and innovation is essential to achieve global TB targets for reductions in TB incidence and TB deaths. The targets of the WHO End TB Strategy ( 1 ), adopted in 2014, required a global rate of decline in TB incidence of 17% per year between 2025 and 2035, compared with a baseline level of 2% per year in 2015.
The WHO reported an alarming 10.6 million cases of tuberculosis in 2021 [1].Indirect evidence suggests that one fourth of the world's population is estimated to be infected with Mycobacterium tuberculosis [2], and 5-10% of those infected develop tuberculosis in their lifetime [3].There is a paradigm shift in our understanding of tuberculosis natural history—instead of perpetuating a binary ...
Treatments for tuberculosis have markedly improved in recent years, but lung disease caused by nontuberculous mycobacteria (NTM) is on the rise and lacks effective cures. This Review discusses ...
Tuberculosis Research and Treatment publishes original research articles and review articles related to all aspects of tuberculosis, from the immunological basis of disease to translational and clinical research. Articles Most Recent; Most Cited; Editorial. Open access.
Tuberculosis (TB) research and innovation is essential to achieve global TB targets for reductions in TB incidence and TB deaths. The targets of the WHO End TB Strategy (1) , adopted in 2014, required a global rate of decline in TB incidence of 17% per year between 2025 and 2035, compared with a baseline level of 2% per year in 2015 and 10% per ...
Tuberculosis (TB) disease incidence has decreased steadily since 1993 (), a result of decades of work by local TB programs to detect, treat, and prevent TB disease and transmission.During 2020, a total of 7,163 TB cases were provisionally reported to CDC's National Tuberculosis Surveillance System (NTSS) by the 50 U.S. states and the District of Columbia (DC), a relative reduction of 20% ...
This Topic aims to propel clinical as well as basic research in the area of TB treatment, disease management, drug discovery and understanding the metabolic re-modelling of Mycobactrium tuberculosis as a pathogen in response to various drugs. We aspire to reach new heights in diagnosis, treatment and control of the tuberculosis epidemic, worldwide.
A multidisciplinary journal focused on understanding the mechanisms behind the development, progression, treatment of tuberculosis and non-tuberculous mycobacterial infections. ... Research Topics See all (13) Learn more about Research Topics. Footer. Guidelines. Author guidelines; Editor guidelines ...
Research Topic Assess Safety, Tolerability, PK, and Efficacy of Delamanid (OPC-67683) in Pediatric Multidrug-resistant Tuberculosis Patients (without HIV) on Therapy With an Optimized Background Regimen of Anti-tuberculosis Drugs Over a 6-Month Treatment Period
Bringing together experts to transform TB prevention and care Latest News. 2024 TRAC Developmental Awardees Announced
Behavioral and social science research. Behavioral and social science research has the potential to make a tremendous impact on TB elimination efforts. This research includes, but is not limited to: Understanding how behaviors of persons at risk for TB, patients and health care providers affect. TB-related care seeking. Diagnosis. Treatment ...
Introduction. Tuberculosis (TB) continues to pose a major threat to global health , and research is a key component of the Global Plan to Stop TB2011-2015 .Research is particularly critical for developing new tools and approaches needed for eliminating TB by 2050 .Recognizing this, the Stop TB Partnership and the World Health Organization's (WHO) Stop TB Department have launched the TB ...
We aimed to systematically summarise priority topics for tuberculosis research from available publications and to describe how priorities were identified. This systematic review will help to inform new initiatives for identifying and setting research priorities, such as for the recently established Research Movement of the Stop TB Partnership ...
Tuberculosis (TB) is a priority for NIAID. It is the leading infectious cause of death worldwide. ... How Is NIAID Addressing This Critical Topic? NIAID supports and conducts basic, translational and clinical research to better understand TB and expedite the development of innovative new tools and strategies to improve diagnosis, prevention and ...
High-quality research evidence is critical for improving global health and health equity, and for achieving the World Health Organization (WHO)'s objective of the attainment of the highest possible level of health by all peoples [1]. This need is most apparent when responding to complex epidemics such as tuberculosis (TB). TB is the leading killer among diseases caused by an infectious agent ...
Frontiers in Tuberculosis has organized this Research Topic to highlight the latest advancements in research across the field of tuberculosis.This editorial initiative is focused on new insights, novel developments, current challenges, latest discoveries, recent advances, and future perspectives in the field of tuberculosis epidemiology.Contributors from various fields are encouraged to submit ...
Additionally, a range of social, economic, and demographic factors affect a person's risk for TB disease.. Racial and ethnic disparities. In 2022, 88.4% of the TB cases reported in the United States occurred among racial and ethnic minority groups.. In the United States, TB affects some racial and ethnic minority groups more than others, including: